Sasha Shillcutt
Patients with heart disease present unique challenges for the anesthesiologist. This chapter provides an overview of those challenges and the associated physiologic changes and anesthetic management strategies needed to safely provide care to patients undergoing cardiac surgical interventions.
I. Coronary Artery Disease
Coronary artery disease (CAD) is one of the most common causes of death in highly developed nations. It results from the buildup of atherosclerotic lesions in the coronary arteries. Myocardial ischemia is a hallmark of CAD. It is caused by an imbalance between myocardial oxygen supply and demand. The anesthesiologist must understand the determinants of this delicate relation and avoid myocardial injury by minimizing myocardial oxygen demand while optimizing myocardial oxygen delivery.
A. Myocardial Oxygen Demand
Systolic wall tension, contractility, and heart rate are the primary determinants of myocardial oxygen demand. Wall tension is directly proportional to systolic blood pressure and chamber size (preload) and inversely proportional to wall thickness. Thus, increases in preload increase wall tension exponentially because as chamber size increases, ventricular wall thickness must thin to accommodate the additional volume. Increases in heart rate are especially deleterious because increases in heart rate increase oxygen demand directly and decrease oxygen delivery indirectly by shortening diastole. The left ventricle (LV) receives its coronary blood flow only during diastole. Thus, increases in wall tension, contractility, and heart rate above normal resting levels must be avoided in patients with CAD.
The left ventricle receives its blood flow only during diastole, while the right ventricle is perfused throughout the cardiac cycle.
B. Myocardial Oxygen Supply
The two main factors contributing to myocardial oxygen supply are arterial oxygen content and coronary blood flow. Recall that arterial oxygen content is represented by the formula:
Because hemoglobin levels and blood volume are usually adequately maintained during cardiac surgery, coronary blood flow is the most critical factor in maintaining myocardial oxygen supply. Coronary blood flow is directly related to coronary perfusion pressure and inversely related to coronary vascular resistance and heart rate (time for perfusion in diastole). Coronary perfusion pressure is estimated as the difference between systemic (aortic) diastolic pressure and left ventricular diastolic pressure. In normal hearts, coronary blood flow is autoregulated for systolic blood pressures between 50 and 150 mm Hg. In patients with CAD, the area of the heart most at risk for ischemia is the subendocardium of the LV. The right ventricle (RV) is perfused during the entire cardiac cycle due to its low intracavitary pressure. Thus, low left ventricular diastolic pressure, normal systemic diastolic pressure, and low heart rate improve myocardial oxygen supply.
C. Monitoring for Ischemia
Flat or down-sloping ST segment depression ≥0.1 mV on the electrocardiogram (ECG) is the most reliable ECG sign of myocardial ischemia. However, transesophageal echocardiography (TEE) has been shown to detect myocardial ischemia earlier and more frequently than the ECG and is very often used in cardiac surgery. Pulmonary artery catheters may reveal acute increases in left atrial pressures associated with ischemia induced stiffening of the LV. However, the pulmonary artery catheters is not a sensitive or specific monitor for myocardial ischemia because so many other things influence left atrial pressure during surgery.
D. Treatment of Ischemia
Myocardial ischemia may occur at any time during coronary bypass surgery. The treatment depends largely on the etiology and can be seen in Table 35-1. Thorough review of the pharmacologic effects of nitrates, peripheral vasoconstrictors, calcium channel blockers and beta-blockers can be found in Chapter 13.
Table 35-1 Treatment of Intraoperative Ischemia
II. Valvular Heart Disease
Growing experience with transesophageal echocardiography has significantly increased the role of anesthesiologists in the intraoperative evaluation and management of valvular heart disease (VHD). VHD can be classified into two primary lesions: regurgitant and stenotic. Regurgitant lesions lead to volume overload, while stenotic valve disease leads to pressure overload. Although disease of the tricuspid and pulmonic valves presents unique challenges to the anesthesiologist, this chapter will focus on the much more common left-sided valvular lesions. Additional information is provided in the 2014 AHA/ACC “Guidelines for the Management of Patients with VHD” (1).
A. Aortic Stenosis
The normal adult aortic valve comprises three equally sized cusps and has an area of 2 to 3.5 cm2. Aortic stenosis (AS) is the most common valvular lesion in the heart and can result from congenital or acquired valvular disease. In congenital AS, there is a partial or complete commissural fusion between cusps, resulting in a unicuspid or bicuspid valve. A bicuspid aortic valve is the most commonly occurring congenital heart defect, affecting approximately 1% to 2% of the population. Bicuspid aortic valves are associated with other congenital abnormalities, specifically diseases of the aorta including coarctation and dilatation of the aortic root. Acquired aortic stenosis results from calcific degeneration or, less commonly, rheumatic disease.
VIDEO 35-1
Aortic Stenosis Asculatation
Progressive narrowing of the aortic valve leads to an increased transvalvular gradient. This in turn increases the work of the LV and over time results in concentric ventricular hypertrophy. This compensatory response allows the internal diameter of the LV to remain unchanged and preserves systolic function and stroke volume. However, as the LV thickens, its diastolic function declines, causing an increase in the diastolic filling pressure. Patients often remain asymptomatic until the valve area is <1 to 1.2 cm2, correlating to a peak transvalvular gradient exceeding 50 mm Hg. The classic triad of symptomatic AS is angina, syncope, and congestive heart failure (dyspnea). The development of any of these is ominous, indicating a life expectancy from 2 to 5 years without valve replacement.
The consequence of elevated intraventricular pressure and concentric hypertrophy is increased myocardial oxygen demand. At the same time, diastolic filling pressure is increased, resulting in a lower coronary perfusion pressure. Thus, patients with severe AS may experience myocardial ischemia and angina in the absence CAD, especially if the heart rate increases much beyond resting levels (see prior discussion in the section “Myocardial Oxygen Demand”). Maintenance of systemic vascular resistance (SVR) is critical in patients with AS to ensure adequate aortic diastolic pressure and thus coronary perfusion pressure. Vasoconstrictors such as vasopressin or phenylephrine will increase SVR without increasing myocardial oxygen demand because the increase in systemic blood pressure they cause is not “seen” by the LV due to the stenotic aortic valve.
B. Hypertrophic Cardiomyopathy
Although not a disease of a valve, hypertrophic cardiomyopathy (HCM) can cause an obstructive lesion similar to that of AS. HCM is an uncommon autosomal dominant genetic disorder with highly variable penetrance. It leads to ventricular hypertrophy that occurs in varying patterns, not just involving the interventricular septum. Presenting symptoms are often dyspnea on exertion, poor exercise tolerance, syncope, palpitations, and fatigue. Some patients remain asymptomatic much of their lives and unfortunately are diagnosed after sudden cardiac death.
Approximately one-third of patients with HCM will have hypertrophy of the interventricular septum that leads to dynamic obstruction of the left ventricular outflow tract. The resulting pressure gradient increases throughout systole, creating obstruction to cardiac output. Any factor decreasing left ventricular size will increase this gradient and further obstruct cardiac output. Examples include increases in heart rate and contractility and decreases in preload and afterload. Therefore, anesthetic management focuses on avoiding tachycardia and maintaining euvolemia and normal systemic vascular resistance. Hypotension in this population is best treated with α-adrenergic agonists and volume. Treatment with inotropic drugs such as epinephrine is contraindicated and may worsen the dynamic obstruction and hypotension.
C. Aortic Insufficiency
Aortic insufficiency (AI) can be the result of primary valvular disease or in association with aortic root dilatation (Marfan disease, degenerative aortic dilatation, aortic dissection) despite a normal aortic valve. The natural progression of AI varies depending on the pathophysiology and chronicity of the disease.
Acute AI is often the result of traumatic injury to the aortic root or valvular endocarditis. The consequences of acute AI are immediate and profound volume overload to the LV. Frequently, the LV is not able to maintain forward stroke volume despite compensatory mechanisms, including increased sympathetic tone, leading to tachycardia and increased contractility. Rapid deterioration of left ventricular function develops, leading to dyspnea and eventual cardiovascular collapse. Acute AI often requires urgent or emergent surgical intervention.
Chronic AI results in an increased left ventricular end diastolic volume that over time leads to eccentric hypertrophy (cavity dilatation). The course of chronic AI is gradual, limiting the increase in left ventricular diastolic pressure. It is not typical for patients to develop symptoms associated with AI until decades into the disease process when the LV has significantly dilated and myocardial dysfunction occurs. Once symptomatic, life expectancy diminishes dramatically, with expected survival of only 5 to 10 years.
The anesthetic management of AI focuses on preserving forward stroke volume and minimizing regurgitant volume by maintaining a relatively rapid heart rate (about 90 beats per minute) and normal to low SVR.
D. Mitral Stenosis
The mitral valve area is typically 4 to 6 cm2 and is made up of an anterior and posterior leaflet. Mitral stenosis is almost always due to rheumatic heart disease and is therefore quite rare in the United States and other highly developed nations. It leads to impaired filling of the LV and a resulting decreased stroke volume. Consequently, the left atrial pressure becomes chronically elevated, resulting in left atrial dilatation and increased pulmonary venous pressure. Patients with mitral stenosis are at high risk for developing atrial fibrillation, which may be the presenting sign of the disease. Mitral stenosis patients are often asymptomatic for decades until the mitral valve area has decreased to 1 to 1.5 cm2 and the heart is faced with an increased demand for systemic stroke volume (exercise, pregnancy, infection). Any high cardiac output state or the onset of atrial fibrillation can cause significant increases in the left atrial and pulmonary arterial pressures, leading to acute congestive heart failure. Chronically elevated left atrial pressures lead to increases in pulmonary vascular resistance, pulmonary hypertension, restrictive lung disease, and right heart failure.
VIDEO 35-2
Atrial Fibrillation
Frequently, patients with mitral stenosis have received diuretics preoperatively to control their pulmonary congestion and are relatively hypovolemic. Induction of anesthesia may unmask the hypovolemia and compromise the transit of blood across the stenotic mitral valve. Thus, adequate fluid administration during anesthesia is crucial, but too much fluid administration can lead to further pulmonary congestion and pulmonary edema. A relatively slow heart rate (about 60 to 70 beats per minute) allows ample time for the LV to fill. Tachycardia compromises that filling and may result in severe hypotension. SVR should be maintained to ensure adequate coronary perfusion pressure, especially to the RV because it is facing increased pulmonary artery pressures.
E. Mitral Regurgitation
Mitral regurgitation (MR) results from excessive leaflet motion (prolapse or flail) or restricted leaflet motion (ischemic dilatation, rheumatic heart disease). Similar to AI, MR leads to volume overload. In MR, the stroke volume is made up of blood ejected into the systemic circulation and then regurgitated into the left atrium. The regurgitated blood causes left atrial and ventricular dilatation (eccentric ventricular hypertrophy) and increased ventricular compliance. Unless the MR is due to CAD (e.g., ischemic rupture of a papillary muscle), an elevated heart rate (about 90 beats per minute) may be best because it will limit ventricular dilatation. However, the cornerstone in the management of MR is reduction of the SVR to promote forward ejection of blood and limit regurgitation. In patients with MR and others undergoing valvular heart surgery, TEE has proven beneficial to assess volume status, ventricular function, and, most important, the adequacy of the surgical procedure. Table 35-2 summarizes the hemodynamic goals in patients with valvular heart disease.
The cornerstone in the management of mitral regurgitation is reduction of the systemic vascular resistance to promote forward ejection of blood and limit regurgitation.
Table 35-2 Hemodynamic Goals in Patients with Valvular Heart Disease
III. Aortic Diseases
The aorta is made up of the aortic root, the ascending aorta, the aortic arch, and the descending thoracic aorta, as seen in Figure 35-1
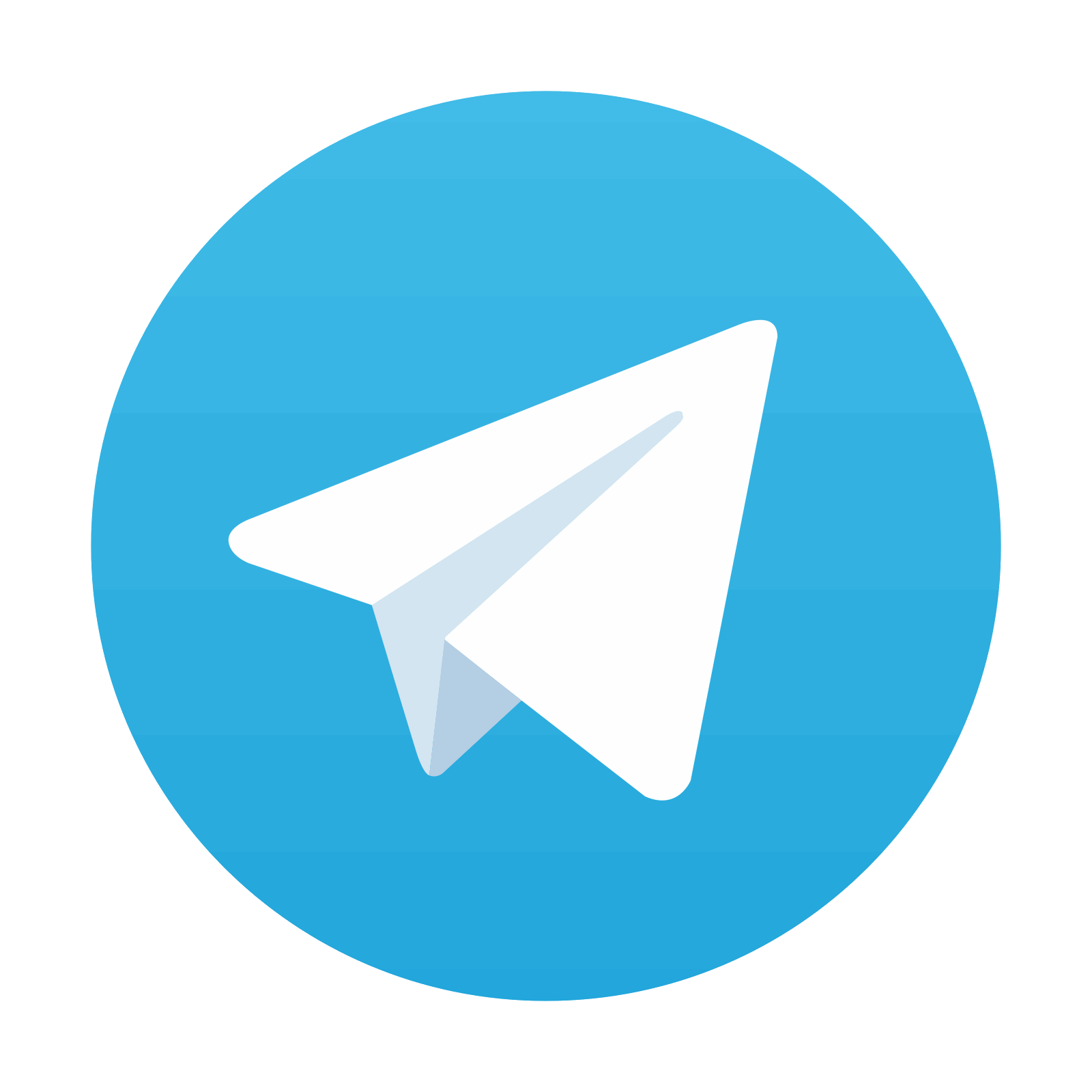
Stay updated, free articles. Join our Telegram channel

Full access? Get Clinical Tree
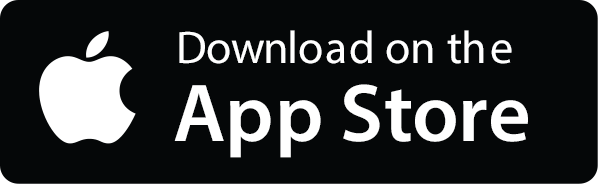
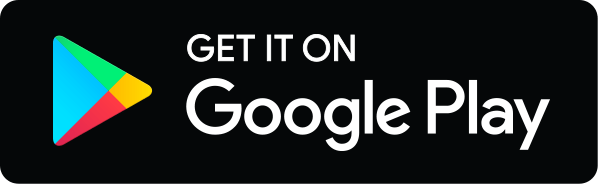