vasoconstriction occurs in areas of the body in which the sympathetic nervous system is intact, usually in areas cephalad to the site of spinal or epidural anesthetic block. The effectiveness of this reflex vasoconstriction in maintaining normotension is a function of the extent of the sympathetic block. If, for instance, sympathetic denervation reaches the fourth thoracic dermatome (T4) or higher, the intact upper limb vasculature may contribute only 5% of the total cardiac output (CO). Even maximal vasoconstriction will be insufficient to compensate for the profound arterial vasodilation in the rest of the body. Reflex cerebral vasoconstriction does not occur, because of the intrinsic cerebral autoregulatory mechanisms.
pressure decrease (48%) was compensated by a relatively larger decrease in myocardial work and oxygen utilization (53%).12
Speed of injection
Total dose and/or volume and concentration of local anesthetic
Intravascular volume status
Patient positioning and
Patient comorbidities (hypertension, diabetes and autonomic dysfunction, chronic diuretic or β-blockade therapy, etc.)
Increasing total circulating blood volume (by the administration of intravenous fluids)
Facilitating venous return to the heart (by placing the operative patient in the horizontal position while elevating the legs)
Augmenting preload (by administering vasoactive agents), and increasing HR (by administering vagolytic agents)
Large volumes of crystalloids produce hemodilution and a decrease in blood viscosity, resulting in increased perfusion and flow in previously shunted areas, and leading to slightly decreased central venous pressure and increased CO. At the same time, hemodilution decreases the blood oxygen-carrying capacity. If hemodilution is excessive, decreased oxygen content may offset the improved blood flow.
The rapid administration of large volumes of crystalloid may be tolerated poorly by patients with decreased myocardial function or with valvular heart disease.
Large volumes of crystalloids will increase the possible complications associated with postoperative urinary retention, because the parasympathetic block of the urinary bladder far outlasts the sympathetic block.
Large volumes of crystalloid may increase the coagulability of blood, leading to an increased incidence of deep venous thrombosis.18
of choice, because glycopyrrolate has been shown to be ineffective in this setting.28 Treatment consists of pharmacologic treatment of bradycardia, rapid administration of fluids, and physical maneuvers to enhance venous return such as head-down positioning.36
TABLE 61.1 Risk Factors Associated with Spinal Hematoma during Low Molecular Weight Heparin Thromboprophylaxis | ||||||||||||||||||||||||||||
---|---|---|---|---|---|---|---|---|---|---|---|---|---|---|---|---|---|---|---|---|---|---|---|---|---|---|---|---|
|
TABLE 61.2 Management of Patients Receiving Low Molecular Weight Heparin | ||||||||||||||||
---|---|---|---|---|---|---|---|---|---|---|---|---|---|---|---|---|
|
TABLE 61.3 Management of Patients Receiving Oral Anticoagulants | ||||||||||||||||||
---|---|---|---|---|---|---|---|---|---|---|---|---|---|---|---|---|---|---|
|
TABLE 61.4 Management of Patients Receiving Antiplatelet Agents | ||||||||||||||||
---|---|---|---|---|---|---|---|---|---|---|---|---|---|---|---|---|
|
liver failure, leukemia, and other entities also preclude the use of central neuraxial block. Cutaneous angiomas may be associated with spinal arterial venous abnormalities.48 Hydraulic pressure changes induced by epidural anesthesia have been associated with rupture of spinal angiomas.49 Therefore, performance of central neuraxial block in these patients should be approached with caution.
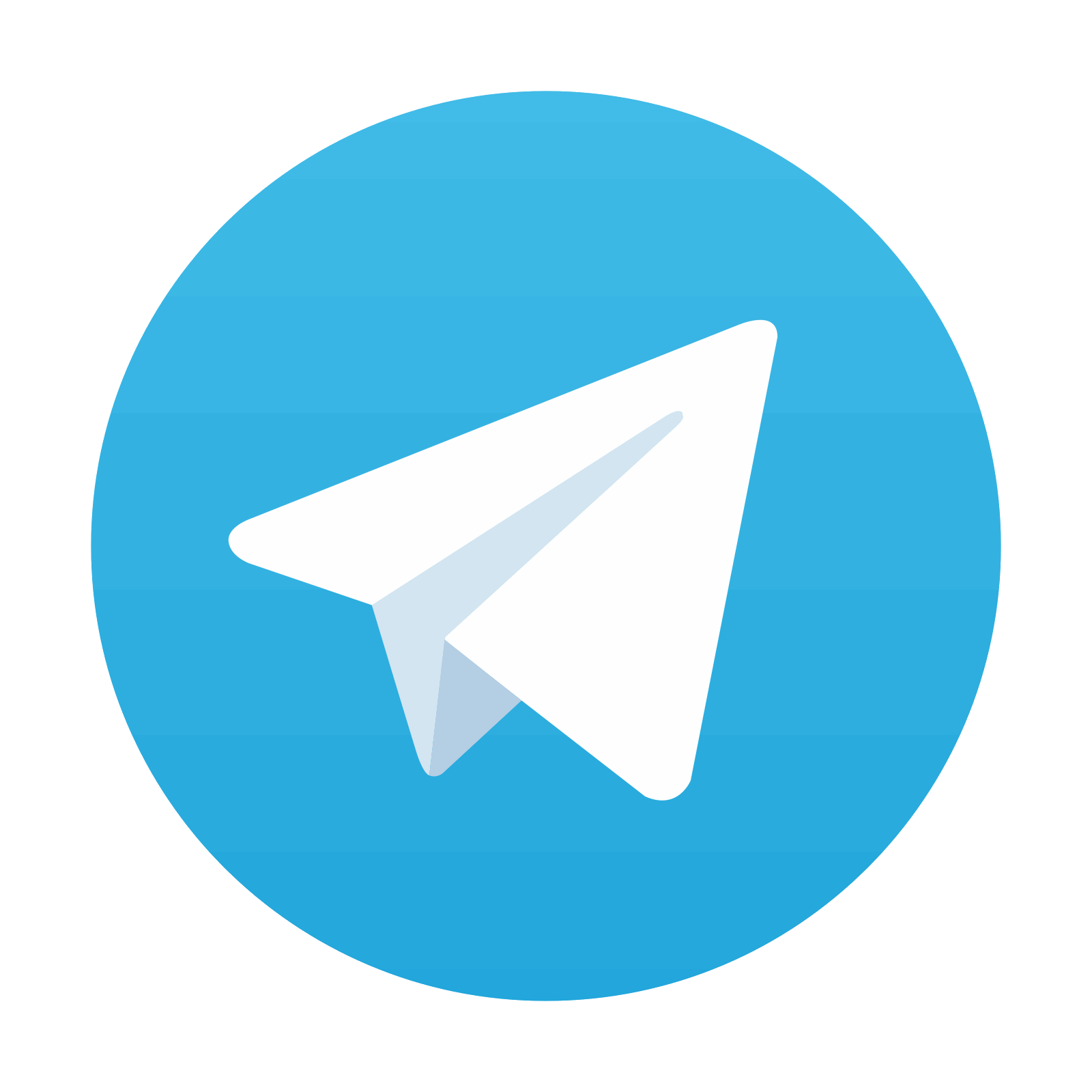
Stay updated, free articles. Join our Telegram channel

Full access? Get Clinical Tree
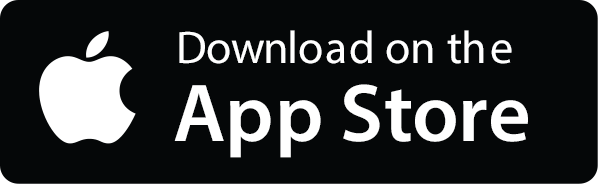
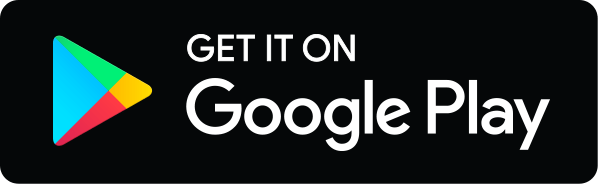