General Anesthetic Agents
Richard J. Rogers
CASE SUMMARY
An 8-year-old, 19-kg female child presented for a repeat embolization of a vein of Galen malformation. The patient had undergone three previous embolizations under general halothane anesthesia over the previous 2 years without complications. As with the prior procedures, the patient was premedicated with oral midazolam (8 mg) in sweetened apple juice. Once appropriate sedation had been achieved, the patient was transported to the neuroradiology suite where standard monitors were applied. Mask induction of anesthesia was easily achieved with nitrous oxide-halothane anesthesia as in the previous embolization procedures. While spontaneous ventilation was maintained, a 22-gauge intravenous catheter was inserted in the left upper extremity, after which the nitrous oxide was discontinued, atracurium (9 mg) was administered, and ventilation was then controlled with the halothane vaporizer set to 1.0% to maintain an end-tidal CO2 of 32 mmHg. During the onset of neuromuscular blockade, occasional atrial premature beats were noted on the electrocardiogram. The halothane vaporizer setting was decreased to 0.5%, and subsequently the atrial premature beats resolved. After the onset of satisfactory neuromuscular blockade, a small dose of thiopental (50 mg) was administered before laryngoscopy. During laryngoscopy, the patient’s cardiac rhythm deteriorated into ventricular fibrillation. While the crash cart was being brought to the bedside, the patient was intubated, ventilated and chest compressions begun. The defribrillator was charged to 50 J, and defibrillation resulted in the return of normal sinus rhythm with normal blood pressure and pulse oximetry values. The patient was maintained on 100% oxygen ventilation and transported to the pediatric intensive care unit (ICU) where she was extubated an hour later. Overnight monitoring and laboratory tests for cardiac enzymes were negative, and the patient was discharged home in the care of her parents the following day.
What Do You Need to Know About Anesthetic Drug Safety?
The administration of drugs for general anesthesia involves several levels of safety considerations. In the United States, the U.S. Food and Drug Administration (FDA) is responsible for ensuring the safety and efficacy of human drugs and biologic products and assuring that they are honestly, accurately, and informatively represented. After approval by the FDA, these drugs must be manufactured and labeled appropriately. The basis of the FDA regulatory authority involves the combination of Title 21 of the Code of Federal Regulations, as well as other scientific information about a specific drug. The powers of the FDA are based on the enactment of the Federal Food, Drug and Cosmetic Act of 1938 (FD&C Act). The law was written to mandate that a drug manufacturer had to provide evidence of the safety of a new product before it could be marketed. In 1962, passage of the Kefauver-Harris Amendments to the FD&C Act stipulated that both the effectiveness and safety of a drug must be proved before marketing. In addition, the amendment required that research on unapproved new drugs be regulated through the review of an investigational new drug application, before the drug can be studied in humans.
For the anesthesiologist, prevention of drug complications involves the proper selection of an anesthetic drug, which includes consideration of the risk-benefit ratio for the specific patient. This process assumes a detailed knowledge of the indications, contraindications, potency, therapeutic effect, and possible side effects of the selected pharmaceutical agent. After the route of administration is chosen, the initial and maintenance dose requirements must be calculated. The particular drug-dosing regimen depends on knowledge of pharmacokinetics as well as pharmacodynamics. In addition to specific knowledge about each drug’s specific storage and
handling, the anesthesiologist should be familiar with the specific adverse effects of each drug.
handling, the anesthesiologist should be familiar with the specific adverse effects of each drug.
What Types of Complications Arise from the Administration of General Anesthetic Agents?
Despite appropriate safety measures, some degree of uncertainty will always exist regarding each patient’s response to specific therapeutic agents. Therefore, adverse drug reactions will occur. An adverse drug reaction, as defined by the World Health Organization, is a noxious, unintended, and undesired effect of a drug that occurs at doses used in humans for prevention, diagnosis, or treatment. Adverse drug reactions are divided into two broad categories: (1) type A and (2) type B.1 Type A reactions are predictable and an extension of the normal pharmacologic actions of the drug; they are often dose-related (e.g., agent-specific side effects). Type B reactions are unpredictable reactions, which may not appear to be directly related to the pharmacologic actions of the drug (e.g., anaphylactic/anaphylactoid and idiosyncratic reactions).
Complications from the administration of general anesthetic agents are usually the result of adverse drug reactions. Many of the adverse effects are dose-related, particularly those with cardiopulmonary manifestations. Type A reactions are fairly common. They may be serious but generally carry a low mortality (e.g., respiratory depression from induction of anesthesia with propofol). Type B reactions are less common but may carry a high mortality (e.g., anaphylaxis).
The most striking example of the type B adverse drug reaction is the unanticipated allergic reaction, which represents an immediate, life-threatening, immunoglobulin E (IgE)-mediated, type-I hypersensitivity to a specific polyvalent antigenic determinant, usually requiring prior sensitization. In contrast, anaphylactoid reactions are non-IgE-mediated and nonallergic in origin and may be clinically indistinguishable from true anaphylaxis.2,3 Examples of anaphylactoid reactions include complement activation and nonspecific histamine release. In the absence of laboratory data documenting an IgE-mediated mechanism, a suspected anaphylactic event must be considered anaphylactoid until proven otherwise.4
Typically, perioperative anaphylaxis occurs quickly and without warning. Attempts to measure the risk of anaphylaxis during all types of anesthesia place the overall incidence between 1/6,000 to 1/20,000 delivered anesthetics;3 of these, two thirds will occur at induction. The associated mortality is estimated to be <10%. Similarly, the risk of anaphylactoid reactions has been estimated at 1/5,000 to 1/7,000 anesthetics.5 Neuromuscular blocking drugs account for more than two thirds of these events.6 It is worth noting that, although 40% of patients who manifest perioperative anaphylaxis have a history of atopy (including pruritis, eczema, wheezing, and rhinitis), there is no evidence that a personal history of atopy predisposes an individual to anaphylaxis.7,8 Furthermore, no correlations have been found between the perioperative risk of anaphylaxis and other variables including age, gender, race, occupation, or geographic location.3
Another type B reaction is the idiosyncratic drug reaction, which is described as an adverse reaction that does not involve known pharmacologic properties of a drug and does not occur in most patients at any dose. The properties of these reactions suggest involvement of the immune system, but in most cases the mechanism has never been determined with any degree of certainty. Examples of serious idiosyncratic drug reactions include liver necrosis, agranulocytosis, aplastic anemia, and toxic epidermal necrolysis. Such reactions also introduce a large degree of uncertainty into drug development because they are not detected by animal testing and are not usually apparent until late during clinical trials or after the drug has been released on the market.
The incidence of adverse drug reactions in hospitalized patients has been estimated at 15%, on the basis of a meta-analysis.9 Nearly half of these reactions were classified as serious in nature. There are less data available on the incidence of adverse drug reactions in the outpatient setting. In ambulatory practices, up to 25% of patients reported adverse drug reactions, with more than half of these considered serious.10,11 Anaphylactic and anaphylactoid reactions are thought to account for approximately 6% to 10% of all adverse drug reactions. The incidence of idiosyncratic reactions is unknown but felt to be much smaller than 1%. Therefore, type A reactions (agent-specific effects) may account for approximately 90% of all adverse drug reactions involving general anesthetic agents. Many of these complications of general anesthetic agents arise as a result of the agent-specific effects being amplified by the combination with other anesthetic agents.
What Are the Possible Adverse Reactions with General Anesthetic Agents?
An encyclopedic cataloging and discussion of all adverse drug reactions associated with the general anesthetic agents currently in use in the United States is beyond the scope of this chapter. Most of these complications are of the type A variety (exaggerated responses to the normal pharmacologic actions of the drug). A thorough knowledge of the pharmacokinetics and pharmacodynamics of the general anesthetic agents will allow prediction of adverse effects in most patients cared for by anesthesia providers. In the following pages, brief synopses of each of the commonly used general anesthetic drugs will be followed by a discussion of pertinent or unusual adverse drug reactions.
▪ NITROUS OXIDE
Joseph Priestly first discovered nitrous oxide in 1772. Although Sir Humphrey Davey first described its analgesic properties in 1800, it was not used as an anesthetic agent until 1884. Currently, nitrous oxide is quantitatively the most widely used anesthetic agent in the United States, most likely as a result of the widespread use by dental and oral surgery practices for outpatient procedures.
The physical properties of nitrous oxide are the basis for most of its anesthetic limitations. The blood-gas partition coefficient of nitrous oxide is 0.47, whereas that of nitrogen is 0.013. Therefore, for every molecule of nitrogen that escapes from air-filled spaces in the body, 36 molecules of nitrous oxide will diffuse in at equilibrium, leading to an increase in hydrostatic pressure in these spaces. Therefore, in clinical settings involving air accumulation, such as the pneumothorax, pneumoencephalon, pneumopericardium, bowel obstruction, or any procedure associated with intracorporeal air enclosure—including middle ear surgery and ophthalmic surgery—and in all cases with an increased risk of air embolism, nitrous oxide use may be contraindicated.
Two serious clinical conditions precipitated by nitrous oxide exposure include megaloblastic anemia12 and subacute, combined degeneration of the spinal cord.13 Exposure to nitrous oxide either at high levels for short term or low levels more chronically has been shown to inactivate the enzyme, methionine synthetase, first demonstrated in vitro.14 Within the vitamin B12 molecule, the highly reduced transition metal (cobalt I) has been shown to catalyze the reductive degradation of nitrous oxide to nitrogen gas and hydroxyl radical15 that inactivates vitamin B12 by an irreversible oxidation of the central cobalt ion. Therefore, nitrous oxide inhibits both methionine synthesis and folate metabolism.
Prospective studies concerning teratogenic effects of nitrous oxide during operations in pregnant women are lacking in the literature. However, experimental evidence in animals suggests that its use in the first trimester of pregnancy, as well as in patients undergoing chemotherapy and patients in severe catabolic states, should be prohibited.16,17
▪ HALOTHANE, ENFLURANE, AND ISOFLURANE
Halothane, a halogenated hydrocarbon, was introduced into clinical use in 1957 and, by the mid-1960s, had become the most commonly used volatile agent in the world. Its introduction was followed by enflurane, a methyl ethyl ether, much later in 1972 and, subsequently, isoflurane, an isomer of enflurane, in 1980. For most of the last 40 years, these halogenated volatile agents have been the mainstay of potent inhalational anesthetics in the United States until the introduction of sevoflurane and desflurane in the 1990s.
A large share of the toxicity of early potent inhalation agents occurred as a result of metabolic breakdown to reactive byproducts. Halothane is extensively metabolized through the cytochrome P450 system, with two isoenzymes (CYP2E1 and CYP2B4) being primarily responsible for its toxicity.18,19 Approximately 25% to 45% of the absorbed halothane undergoes oxidative metabolism, with the major metabolites being trifluoroacetyl chloride and trifluoroacetic acid. The end products of the oxidative pathways that are detected in the urine are the sodium salts of trifluoroacetic acid, chloride, and bromide. Only approximately 2% of inhaled enflurane20 and 0.2% of inhaled isoflurane are metabolized.21 Traces of trifluoroacetic acid have been detected in human urine after isoflurane anesthesia.
The organ most affected by breakdown products of potent inhalational anesthetic agents is the liver. The incidence and extent of hepatotoxicity directly attributable to inhaled anesthetics are difficult to determine. Drugmediated hepatotoxicity ranges in severity from mild dysfunction to massive hepatic necrosis. All anesthetic techniques reduce hepatic blood flow to some degree and may contribute to postoperative liver dysfunction. However, surgical manipulation and the surgical site appear to be more important factors in decreasing liver blood flow than is the anesthetic agent or technique.22 The trifluoroacetyl chloride intermediate of halothane metabolism is unstable and reactive with multiple proteins within the liver. The trifluoroacetylated hepatic proteins are believed to be involved in the immune response leading to halothane hepatitis.23,24
Enflurane can be associated with hepatitis, but the incidence is very low, approximately 1 in 800,000.25 The mechanism is not clear, because the metabolism of enflurane is very different from that of halothane. The evidence for postisoflurane hepatitis is very scant, and it is highly unlikely that isoflurane is even rarely responsible for postoperative hepatotoxicity.26
Other potential adverse effects of halothane and other potent inhalational agents involve their effects on the cardiovascular system. All of the older inhaled anesthetic agents (halothane, enflurane, isoflurane) cause a direct, potent, and dose-dependent depression of myocardial contractility (halothane causes myocardial depression to approximately 50% of control values at 1 mean alveolar concentration). The order of potency for depressing myocardial contractility is enflurane > halothane > isoflurane.27 An index of the cardiac safety of an anesthetic agent is provided by the difference in the concentration producing anesthesia and the concentration producing lethal circulatory failure in animals.28 The greatest safety is provided by isoflurane, followed by halothane and enflurane, reflecting the order of potency for myocardial depression.
Inhaled anesthetic agents cause decreases in peripheral vascular resistance. The effect of halothane on smooth muscle is similar to its effect on cardiac muscle, but the effect depends on the region of the vasculature and on the dose of the agent.29 Peripheral vascular resistance is reduced most with isoflurane and least with enflurane.
Halothane, enflurane, and isoflurane depress the baroreceptor reflex, which increases heart rate in response to hypotension. Isoflurane has the least effect. Hence, tachycardia secondary to the hypotension caused by
both a decrease in peripheral vascular resistance and myocardial depression is often seen in patients receiving isoflurane.30 All three inhalational agents decrease the rate of sinoatrial node discharge, with halothane being the most dramatic.31 A reduction in the automaticity of the SA node leads to slowing of the heart rate and often to junctional rhythms.
both a decrease in peripheral vascular resistance and myocardial depression is often seen in patients receiving isoflurane.30 All three inhalational agents decrease the rate of sinoatrial node discharge, with halothane being the most dramatic.31 A reduction in the automaticity of the SA node leads to slowing of the heart rate and often to junctional rhythms.
Halothane as a hydrocarbon will sensitize the heart to the arrhythmogenic properties of catecholamines.32,33 Increased levels of catecholamines, whether endogenous (stress of anesthesia and surgery) or exogenous, in combination with a slowing of nodal conduction, can lead to reentry arrhythmias. In animals, the arrhythmogenic potential enhancement to catecholamines is independent of the dose of halothane between alveolar concentrations of 0.5% and 2%.34 Therefore, therapeutic interventions, other than decreasing the inhaled halothane concentration, may be required to treat and prevent cardiac arrhythmias due to catecholamines. Unlike halothane, enflurane and isoflurane do not sensitize the heart to the arrythmogenic effects of catecholamines at commonly used anesthetic concentrations. This disparity is likely due to the chemical differences between the agents; halothane is an alkane whereas enflurane and isoflurane are ethers.
▪ SEVOFLURANE AND DESFLURANE
Sevoflurane and desflurane, introduced as new volatile anesthetic agents in the early 1990s, exhibit low blood solubility as their principal advantage over older potent inhalational agents. The cardiovascular effects of sevoflurane and desflurane are similar to isoflurane.35 They produce only a mild depression in myocardial contractility compared to halothane. The arterial blood pressure declines because of a direct effect of the ether-based anesthetics to relax vascular smooth muscle. The compensatory increase in heart rate needed to maintain perfusion occurs to a lesser degree with sevoflurane compared to isoflurane and desflurane.
Desflurane is highly pungent and commonly produces airway irritation. Approximately 30% to 40% of patients develop coughing, breath-holding, excessive salivation, or laryngospasm during induction of general anesthesia. On occasion, similar but less intense responses are seen on emergence from anesthesia.36,37 Sevoflurane possesses low pungency and does not produce respiratory irritation on induction compared with isoflurane and desflurane.38 Therefore, sevoflurane is commonly used for induction of anesthesia before gaining intravenous access.39,40
Desflurane and isoflurane cause sympathetic nervous system activation at concentrations of 5% to 6%, especially with abrupt and large increases in concentration. The critical difference between desflurane and isoflurane is that only desflurane causes it at a clinical concentration (approximately 1 mean alveolar concentration).41 Sevoflurane does not produce this effect at any concentration. This effect becomes relevant during procedures in which rapid changes in the level of anesthesia are required, such as on induction or with sudden changes in surgical stimulation.42
Sevoflurane is metabolized at a faster rate than isoflurane or desflurane, producing higher plasma levels of the nephrotoxic byproduct, inorganic fluoride. Concerns arose regarding the high plasma fluoride concentrations and the potential effects on renal function with the use of sevoflurane. However, subsequent studies have demonstrated that, despite high plasma fluoride levels (>50 µM), sevoflurane does not cause clinically significant renal damage.43
A great deal of attention has been focused on the metabolic degradation products of sevoflurane, most notably Compound A (fluoromethyl-2,2-difluoro-1-[trifluoromethyl] vinyl ether). Compound A is a fluorinated olefin formed in the presence of alkali such as that found in commercial CO2 absorbers. Several studies have documented the renal and pulmonary toxicity of Compound A in rats, in which the median lethal concentration (LC50) has been shown to decrease as the duration of exposure increases. Furthermore, unlike fluoride, the breathing circuit concentration of Compound A has been shown to be highest during low flow anesthetic gas administration, which may be prompted as a cost-saving measure. Prolonged low flow (1 L per minute) anesthesia with sevoflurane produces Compound A concentrations of only 13 to 35 ppm, values that are fourfold to tenfold lower than the rat LC50 of 120 to 220 ppm. Multiple clinical studies have failed to document significant renal, hepatic, or pulmonary impairment at these low levels of exposure.44,45,46 The formation of Compound A can be influenced by several factors. In particular, higher levels of Compound A have been found in the presence of bariumcontaining CO2 absorbers, fresh and dry CO2 absorbent, higher volume percent sevoflurane, lower total gas flows, and elevated CO2 absorbent temperatures.46,47 Compound A concentrations may be reduced and exposure limited by using higher total fresh gas flows (minimum flow rate of 2 L per minute mandated by the FDA), lower concentrations of sevoflurane, and sodium hydroxide containing CO2 absorbent.
When carbon dioxide absorbents are desiccated, the absorbents degrade all modern anesthetics and produce carbon monoxide.48,49,50 High temperature increased the production of carbon monoxide, and barium hydroxide was shown to produce a higher level of carbon monoxide than did sodium hydroxide.49,51 Factors that contribute to the differences in carbon monoxide formation among the anesthetic agents are inconclusive. The rank order of carbon monoxide formation is desflurane > enflurane > isoflurane. Sevoflurane and halothane form negligible amounts.49
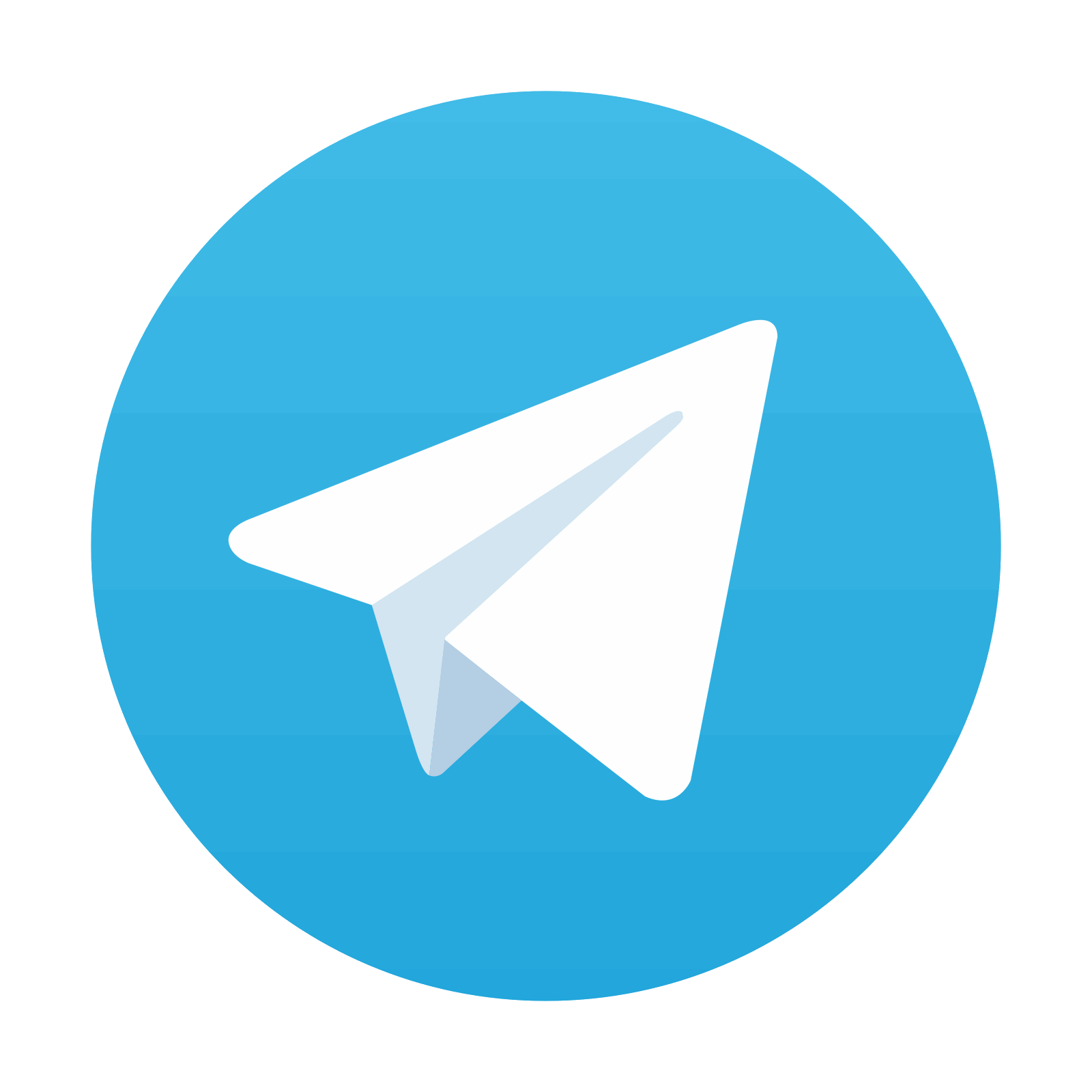
Stay updated, free articles. Join our Telegram channel

Full access? Get Clinical Tree
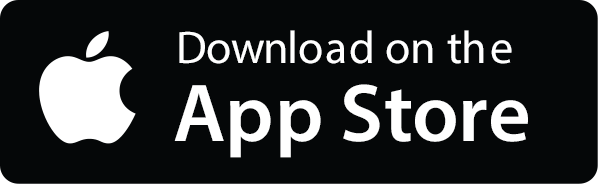
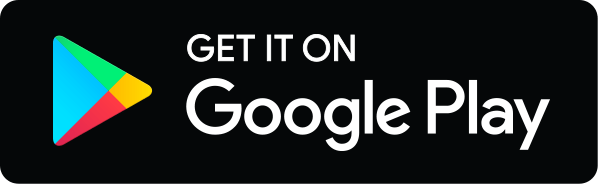