Dyslipidemias
Joseph Reilly PharmD
Steven Richardson MD
Coronary artery disease (CAD) is the leading cause of death for both men and women in the United States, and its incidence increases linearly with age. Large population studies demonstrate a clear relation between cholesterol levels and CAD risk, especially in subjects with cholesterol levels more than 200 to 240 mg/dL. Over the last two decades, overwhelming evidence has accumulated demonstrating this relation as well as the benefits of reducing serum cholesterol with a reduced risk of CAD. This bidirectional relation has led to the establishment of a National Cholesterol Education Program (NCEP) (Expert Panel, 1994). The approach of the NCEP is to identify and treat high-risk patients with elevated cholesterol levels and to encourage the general public to modify life habits to reduce cholesterol concentrations with other risk factors for CAD that have been identified (see Chap. 9) (Table 10-1).
Successful management of hyperlipidemic patients requires a thorough understanding of nonpharmacologic and pharmacologic therapies. Health care providers must also have an understanding of the multifactorial nature of the process of atherogenesis (see Chap. 9). Although the focus of this review is the role of hyperlipidemia in CAD and the current approaches to correcting hyperlipidemia, many patients who have CAD and suffer a myocardial infarction (MI) do not have high cholesterol levels.
ANATOMY, PHYSIOLOGY, AND PATHOLOGY
Lipid Metabolism and Pathophysiology
Cholesterol is a water-insoluble molecule that is essential for cell membrane formation and the synthesis of steroid hormones and bile acids. Cells derive cholesterol from intracellular synthesis or extraction from the systemic circulation. Daily cholesterol intake ranges from 250 to 500 mg, with approximately 50% being absorbed (Grundy et al, 1978). Body synthesis of cholesterol ranges from 500 to 1000 mg/dL (Grundy et al, 1966, 1969). Therefore, approximately 25% of the body’s total cholesterol source is from dietary intake and 75% is synthesized in vivo. Cellular synthesis of cholesterol takes place through a series of biochemical steps involving a number of enzymes (Fig. 10-1). An important class of drugs, the hydroxymethylglutaryl coenzyme A (HMG CoA) reductase inhibitors, also referred to as the statins, disrupts this process; these drugs are commonly used in patients with elevated cholesterol levels.
Cholesterol and triglycerides in the diet enter into the exogenous pathway of lipid transport. Cholesterol in the intestine must be solubilized before it can be absorbed, and absorption is incomplete because cholesterol is highly insoluble in aqueous solutions (Grundy et al, 1996). In the intestine, cholesterol is in the unesterified (free) form and becomes esterified with a fatty acid when it enters the intestinal mucosa. Triglycerides are reformulated from absorbed fatty acids and monoglycerides and are incorporated with cholesterol esters into chylomicrons. These lipoproteins eventually reach the systemic circulation and undergo partial catabolism to chylomicron remnants carrying newly absorbed cholesterol into the liver (Grundy et al, 1996).
Hepatic cholesterol comes from newly absorbed cholesterol, from the uptake of serum lipoproteins, and via hepatic synthesis. In the liver, cholesterol can be incorporated into lipoproteins that are secreted into the plasma; their density is determined by their relative content of protein and lipid. Hepatic cholesterol synthesis (endogenous pathway) is regulated by the quantity of cholesterol in the liver. Bile acid synthesis is governed by the quantity of bile acids returning to the liver via enterohepatic circulation (Grundy et al, 1996). The primary route of cholesterol excretion is into the bile as cholesterol or as bile acids. About one third of the cholesterol synthesized or absorbed is converted into bile acids, which can be reabsorbed into the portal circulation and return to the liver (Grundy et al, 1966, 1969).
Lipid disorders can result from a dysfunction in any one of the numerous steps involved in the process of lipid metabolism. Table 10-2 categorizes the more common lipid disorders.
Lipoproteins
Lipids are present in the plasma as circulating lipoproteins that transport cholesterol and triglycerides. The basic structure of a typical lipoprotein is shown in Figure 10-2. These lipoproteins are divided into classes, typically based on their density and composition. The major lipoproteins include chylomicrons, very-low-density lipoproteins (VLDL), intermediate-density lipoproteins or remnant particles, low-density lipoproteins (LDL), and high-density lipoproteins (HDL) (Table 10-3). LDL accounts for approximately 60% to 70% of total serum cholesterol, HDL 20% to 30%, and VLDL about 10% to 15%. In addition, each lipoprotein particle contains proteins on its outer surface called apolipoproteins. These proteins have several functions, such as activating enzyme systems, providing structure to the lipoprotein, and binding with cell receptors.
Apolipoproteins
Four major classes of apolipoproteins exist: apo B, apo A, apo C, and apo E (Table 10-4). Patients with abnormal metabolism of apolipoproteins may be at an increased risk of atherosclerosis even if they have normal cholesterol levels. Therefore, many health care providers believe that apolipoprotein levels should be used to evaluate hyperlipidemic patients.
Chylomicrons
Synthesized in the intestine, chylomicrons are large triglyceride-rich particles with apolipoproteins B-48, apo A, apo C, and apo E. In the peripheral circulation, chylomicrons interact with lipoprotein lipase on the vascular endothelium, which hydrolyzes the triglycerides into free fatty acids and monoglycerides, which are then absorbed by muscle and adipose tissues. The resultant product is a cholesterol-rich chylomicron remnant that is taken up by the liver. In patients with a lipoprotein lipase deficiency, this process is defective and hypertriglyceridemia results.
Newly absorbed cholesterol thus passes into the liver in chylomicron remnants. Fatty acids of chylomicron triglycerides are released into the peripheral circulation and can be used by muscle for energy, adipose tissue, and the liver. After a fatty meal, chylomicron levels are elevated, and therefore triglycerides are high. However, after a 12- to 24-hour fast, chylomicrons will have cleared from the blood. Fasting triglyceride concentrations reflect hepatic production and that which is carried by in VLDL and other remnant particles. For this reason, patients are required to fast before obtaining a lipoprotein profile.
Very-Low-Density Lipoproteins
Triglyceride-rich VLDL particles are produced by the liver and contain apo B-100 and apo E on their surface. The nascent VLDL particles circulate and acquire cholesterol esters and apolipoproteins (including apo C), developing into mature VLDLs. The B and E proteins are ligands for LDL receptors, and a defect in these receptors results in increased levels of cholesterol. Apolipoprotein C-II activates lipoprotein lipase, which interacts with VLDL as it does with chylomicrons. Fatty acids are released, producing remnant VLDL and intermediate-density lipoproteins. These remnants are eventually metabolized by the liver or converted to LDL. Most of the triglycerides are removed and replaced with cholesterol esters. Deficiencies of C-II apolipoproteins result in faulty triglyceride metabolism and hypertriglyceridemia.
Low-Density Lipoproteins
The LDLs are the major cholesterol transport lipoproteins in the plasma. They contain cholesterol ester in their core and only apolipoprotein B-100 on their surface. LDL is derived from VLDL catabolism and cellular synthesis. LDL is cleared by the liver and extrahepatic tissues, which recognize the apo B-100 and bind and internalize the particle, which is then degraded. Excess circulating LDL will result in deposition of cholesterol outside the cell, causing the formation of atherogenic plaques in the vascular endothelium. Because LDL contains 60% to 70% of the total blood cholesterol and there is a direct relation between the LDL concentration in the systemic circulation and atherosclerosis, LDL is the primary target of cholesterol-lowering therapies, in accordance with NCEP guidelines.
High-Density Lipoproteins
The smallest of the lipoprotein particles, HDL appears to transport cholesterol from peripheral cells to the liver. The liver and the gut secrete nascent HDL that consist of apo A and phospholipids. These particles acquire unesterified cholesterol that becomes esterified by lecithin–cholesterol acyltransferase, forming HDL-3 particles. HDL-3 acquires more cholesterol, forming HDL-2, which in turn may be converted back to HDL-3 by hepatic lipase and by transferring cholesterol esters to the liver, LDL, and VLDL. The transfer of cholesterol from HDL particles to lipoproteins is catalyzed by the enzyme cholesterol ester transfer protein. This complex HDL cycle is a critical part of the reverse cholesterol transport process, where cholesterol is returned to the liver for excretion.
In contrast to LDL, high HDL concentrations are desirable because they provide protective effects against the atherogenic process. Because HDL particles contain apo A-I, which activates lecithin–cholesterol acyltransferase, levels of apo A-I have a strong inverse correlation with CAD. Patients with abnormally low HDL levels (<35 mg/dL) are at an increased risk for CAD, presumably because of the decreased ability to remove
circulating cholesterol. In general, for every 1% decrease in HDL, there is a 2% to 3% increase in CAD (Gordon et al, 1989). In contrast, an elevated HDL level (≥60 mg/dL) is considered a negative risk factor for CAD.
circulating cholesterol. In general, for every 1% decrease in HDL, there is a 2% to 3% increase in CAD (Gordon et al, 1989). In contrast, an elevated HDL level (≥60 mg/dL) is considered a negative risk factor for CAD.
Low-Density-Lipoprotein Receptors
Cholesterol uptake by hepatic and peripheral cells is accomplished by the binding of apolipoproteins found on circulating lipoproteins to LDL receptors on cell surfaces. LDL receptor synthesis occurs when there are low concentrations of intracellular cholesterol. These receptors are capable of binding with lipoproteins containing apolipoproteins E or B-100. Once bound, these lipoproteins are degraded. Drugs capable of reducing the intracellular synthesis of cholesterol can upregulate LDL receptors, thus enhancing removal of cholesterol from the systemic circulation.
ETIOLOGY OF HYPERLIPIDEMIA
The most severe forms of hyperlipidemia occur in patients with a defect in lipid metabolism or transport (see Table 10-2). Persons with these hereditary diseases or primary causes of hyperlipidemia typically require pharmacotherapy in conjunction with lifestyle modifications to correct or normalize their condition.
Secondary causes of hyperlipidemia can be attributed to lifestyle and underlying disease states (Table 10-5). In addition, many medications can have a negative effect on the lipid profile (Table 10-6). Many of these medications are used to treat comorbid disease states such as hypertension, congestive heart failure, and angina. Health care providers should evaluate the hyperlipidemic patient’s current medications and identify the drugs that may be contributing to the dyslipidemic state. In some cases, a suspected medication can be replaced by one that has a neutral effect on lipids or one that has a beneficial effect. Angiotensin-converting enzyme (ACE) inhibitors and calcium channel blockers have a neutral effect on lipids; postsynaptic alpha-1 receptor blockers (eg, prazosin, terazosin, doxazosin) have favorable lipoprotein effects.
A person’s dietary habits and lifestyle have a significant effect on the lipid profile and body weight, which can be positively correlated with LDL and triglyceride levels and negatively correlated with HDL levels. Patients who are overweight will benefit from weight loss, as seen by reduced LDL and triglyceride levels and an increased HDL level. Modifications in diet can result in weight loss and improvements in the lipoprotein profile. For these reasons, lifestyle modifications including dietary therapy are considered the foundation of therapy.
RATIONALE FOR THERAPY OF DYSLIPIDEMIA
Epidemiologic studies have greatly contributed to our understanding of CAD in patients with high blood cholesterol levels. A direct relation between total cholesterol and LDL levels in the blood and death and disability from coronary heart disease (CHD) has been demonstrated consistently. One of the most notable studies is the Framingham Heart Study (Kannel et al, 1971). In this ongoing study, the population of Framingham, Massachusetts, has been followed for more than 30 years, documenting that cardiovascular risk factors are related to CHD rates where serum cholesterol levels are correlated with CHD rates. In addition, long-term survival is inversely related to total cholesterol level at entry to the study (Anderson et al, 1987).
Another investigation, the Multiple Risk Factor Intervention Trial, involved more than 360,000 men in the United States (Stamler et al, 1986). The study participants were
screened and followed for 6 years for CHD mortality. This trial showed a positive curvilinear correlation between initial cholesterol levels and subsequent CHD mortality, further emphasizing the relation between high cholesterol levels and CHD.
screened and followed for 6 years for CHD mortality. This trial showed a positive curvilinear correlation between initial cholesterol levels and subsequent CHD mortality, further emphasizing the relation between high cholesterol levels and CHD.
Individual lipoprotein fractions and CHD rates have similarly been established. The Framingham Heart Study (Kannel et al, 1979). revealed the association between risk factors and CHD: a high LDL level was found to be more predictive of CHD than the total cholesterol level. The significance of this relation is evident in the NCEP’s recommendations, in which LDL is the primary target of therapy in patients with hyperlipidemia. This same study (Kannel et al, 1979; Cordon et al, 1977) has also provided valuable data about the negative relation between HDL level and CHD.
Serum triglyceride concentrations are also correlated with CHD risk (Austin, 1991). The Framingham Heart Study (Castelli, 1986) showed that the VLDL level (reflecting serum triglycerides) was a predictor of CHD. However, the specific relation between serum triglyceride levels and CAD was not very clear. Atherosclerotic plaques primarily contain cholesterol, not triglycerides. However, in patients with hypertriglyceridemia, there is often an accompanying increase in other atherogenic lipoproteins and a decreased HDL level. Therefore, an elevation in triglycerides may result in an increase of other lipoproteins that promote CAD. When serum triglycerides exceed 1000 mg/dL, the most immediate danger is acute pancreatitis. In patients with diabetes mellitus, reduction of glucose levels may significantly lower triglyceride levels.
The benefits of cholesterol-lowering therapies in patients with existing CHD (secondary prevention) and those without CHD (primary prevention) have been firmly established. The following is a brief review of the major primary and secondary prevention trials.
TEACHING AND SELF-CARE
Primary Prevention
The key primary prevention trials—those establishing the relation between blood cholesterol levels and CHD in patients without evidence of CHD—are summarized in Table 10-7. These studies evaluated the treatment of hyperlipidemic patients with diet and with and without pharmacotherapy. These trials justify the use of cholesterol-lowering therapies in asymptomatic patients to prevent CHD.
The Lipid Research Clinics Coronary Primary Prevention Trial (Lipid Research Clinics Program, 1984a, 1984b) involved more than 3800 hypercholesterolemic men, dividing them into placebo and cholestyramine treatment groups. After 7 years of treatment, the active treatment group had 19% fewer CHD events than the placebo group. Thus, patients receiving cholestyramine had significantly fewer CHD deaths and nonfatal MIs.
Another major drug trial was the Helsinki Heart Study, which compared gemfibrozil and placebo in more than 4000 Finnish men (Frick et al, 1987; Manninen et al, 1988). Over the 5-year period of the trial, the gemfibrozil group had a 34% reduction in major coronary events.
The West of Scotland Coronary Prevention Study (Shepherd et al, 1995) included more than 6500 men with hyperlipidemia. Patients were assigned to receive pravastatin 40 mg/day or placebo for approximately 5 years. Patients receiving the pravastatin had a more than 30% reduction in CHD deaths, nonfatal MIs, and the need for revascularization procedures when compared to the placebo group. There was also a 22% reduction in total mortality in the pravastatin group, a clinically significant finding.
These clinical trials provide ample support for the identification and management of patients for primary prevention. More specifically, they demonstrated that for every 1 mg/dL reduction in cholesterol, the risk for CAD is reduced by 1%. However, most primary prevention trials have failed to show a decrease in the total mortality rate. This can be explained by a number of reasons, including study design and a lack of statistical power. In contrast, the pharmacologic agents used in these studies may have had deleterious side effects that offset their beneficial cholesterol-lowering properties. However, the abundance of data supporting cholesterol-lowering therapies justifies the treatment of patients without CAD and with elevated cholesterol levels.
Secondary Prevention
A number of secondary prevention trials, conducted in patients who have had MI or evidence of atherosclerotic vascular disease, have demonstrated the benefits of cholesterol-lowering interventions (see Table 10-7). The first study using an HMG CoA reductase inhibitor demonstrated the benefits of this potent class of drugs. In the Scandinavian Simvastatin Survival Study (4S, 1994), 4444 patients received simvastatin or placebo and were followed for more than 5 years. Patients in the simvastatin group had a 30% decrease in the total mortality rate, a 42% decrease in CHD deaths, a 37% decrease in revascularization procedures (eg, percutaneous transluminal coronary angioplasty or coronary artery bypass grafting), a 34% decrease in nonfatal MI or CHD death, and a 30% decrease in stroke. The difference between the treatment groups was evident by 2 years, after which the groups continued to diverge. Positive results were seen across all patient types, including women and the elderly. This landmark trial was the first to improve the overall mortality rate with a statin and therefore justified what many health care providers were already doing—using a statin as a first-line agent.
Since the 4S trial, another study involving pravastatin, the Cholesterol and Recurrent Events trial (Sacks et al, 1996), has provided more evidence for the use of statins in secondary prevention. This study involved more than 4100 patients with lower baseline cholesterol levels than those in the 4S trial. Study participants received either pravastatin or placebo, and the mean follow-up was 5 years. When compared to placebo, patients receiving pravastatin had a 24% reduction in CHD deaths and nonfatal MI, a 25% reduction in MI alone, and a 27% reduction in revascularization procedures.
From these secondary prevention trials, we have learned that the magnitude of LDL reduction was again correlated with the degree of benefit. It is clear from the results of these trials that aggressive LDL reduction in patients with high LDL levels has profound effects. There is still a benefit if the LDL is lower, but as expected it is not quite as great.
Angiographic Regression Trials
Another group of studies in patients with established CAD used angiography (see Table 10-7). The goal of these studies was to determine whether lipid-lowering interventions can retard
progression and promote regression of coronary lesions. The findings of these trials indicate that by reducing cholesterol, the rate of coronary lesion progression was slowed, and in some cases regression was evident with changes in lumen size. An important benefit found was lesion stabilization and a decrease in CHD events. The lipid-lowering effects of an intervention are believed to reduce the lipid core of the unstable atherosclerotic lesion, rendering the lesion more stable and less likely to rupture and form a thrombus, producing an acute coronary event. These results support aggressive lipid-lowering interventions in patients with angiographic evidence of atherosclerosis to prevent CHD events.
progression and promote regression of coronary lesions. The findings of these trials indicate that by reducing cholesterol, the rate of coronary lesion progression was slowed, and in some cases regression was evident with changes in lumen size. An important benefit found was lesion stabilization and a decrease in CHD events. The lipid-lowering effects of an intervention are believed to reduce the lipid core of the unstable atherosclerotic lesion, rendering the lesion more stable and less likely to rupture and form a thrombus, producing an acute coronary event. These results support aggressive lipid-lowering interventions in patients with angiographic evidence of atherosclerosis to prevent CHD events.
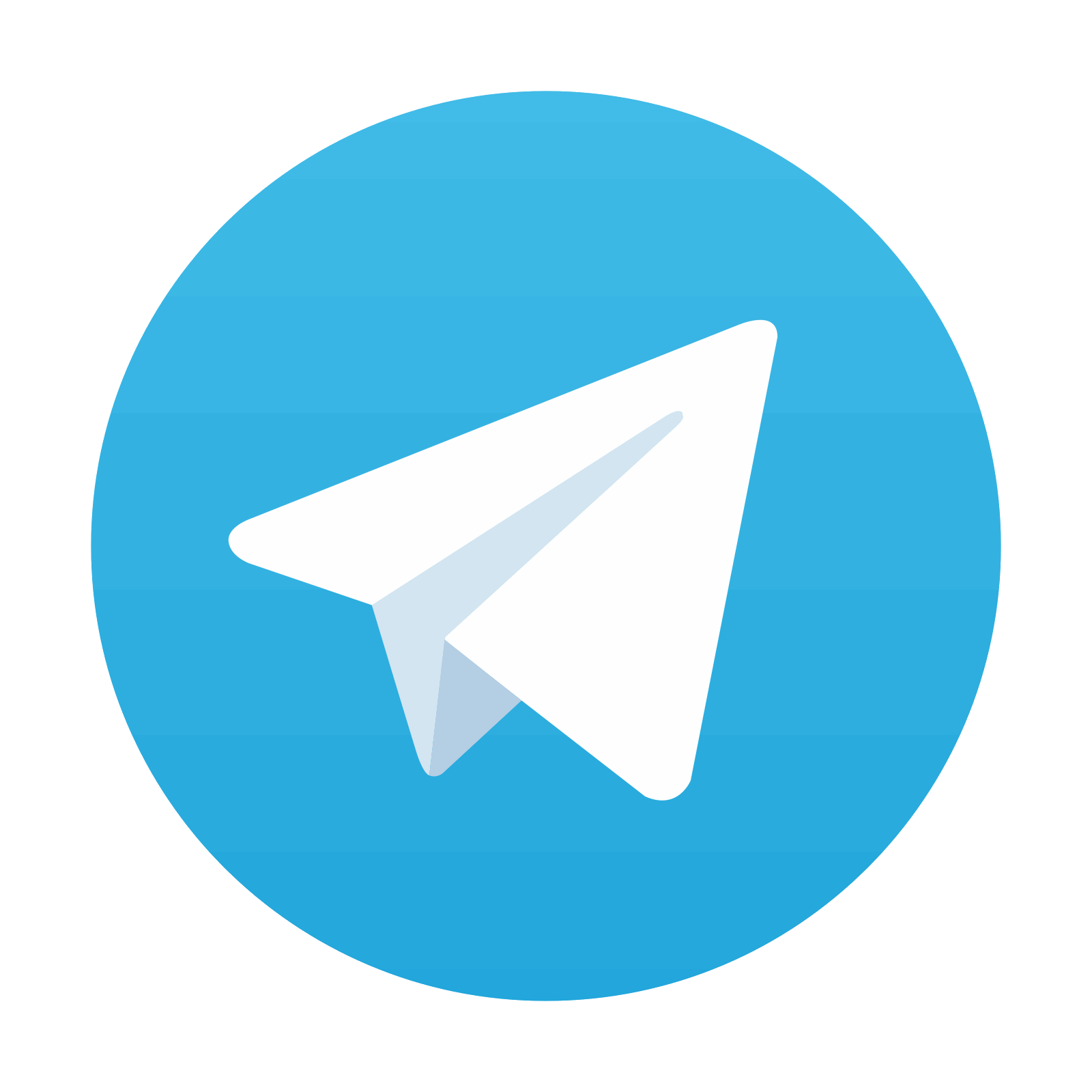
Stay updated, free articles. Join our Telegram channel

Full access? Get Clinical Tree
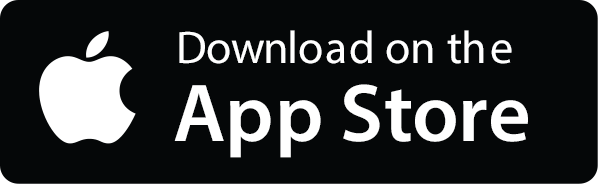
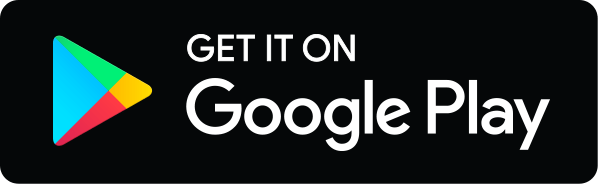