ZONA GLOMERULOSA: This layer comprises approximately 15% of the gland and is the main site of mineralocorticoid production. The major mineralocorticoid is aldosterone (95%), with the balance being made up by corticosterone and deoxycorticosterone.
ZONA FASICULATA: This layer is the major part of the gland and is the primary site for glucocorticoid production (mainly cortisol and, to a much lesser extent, cortisone).
ZONA RETICULARIS: This is the site of both anabolic and sex hormone synthesis. The adrenal steroids contain either 19 or 21 carbon atoms. C19 steroids are mainly androgenic in activity, whereas the C21 steroids have either glucocorticoid or mineralocorticoid properties. The hormones are transported bound to plasma proteins.
and its biologically inactive metabolites can be filtered at the glomerulus. The average production rate of cortisol is 12 to 15 mg/m2/day. Cortisol is rapidly metabolized, mainly in the liver. One of the main enzymes that regulates cortisol metabolism is 11 β-hydroxysteroid dehydrogenase. The major metabolites are formed by the reduction of the double bond and ketone groups to produce tetrahydrocortisol, tetrahydrocortisone, cortol, and cortolone.4 These metabolites are excreted into the urine in conjugation with glucoronic acid, and account for approximately 60% to 70% of the total cortisol produced. A small amount of cortisol (up to 50 µg per day) is excreted unchanged in the urine and represents the unbound cortisol in plasma that is filtered through the kidney.
and the release can be selective. Under normal physiologic conditions, approximately 80% of the catecholamine output from the adrenal medulla is epinephrine.
chest pain and ECG changes suspicious for ischemia. Despite striking repolarization changes, many patients who proceed to coronary angiography preoperatively are found to have no obstruction of their coronary arteries. Anecdotal reports suggest ECG changes to be a manifestation of toxic myocarditis. In addition to the ECG changes mentioned, many patients with a pheochromocytoma are noted to have a long QTc interval which may predispose to ventricular arrhythmias.18 After removal of the pheochromocytoma, the QTc intervals tend to normalize. An elevated temperature more commonly reflects a catecholaminemediated increase in the metabolic rate and diminished heat dissipation secondary to vasoconstriction. Polyuria is an occasional finding, and rhabdomyolysis with resultant myoglobinuric renal failure may result from extreme vasoconstriction and ensuing muscle ischemia.
TABLE 43.1 Specific Tests for Diagnosing Pheochromocytoma | ||||||||||||||||
---|---|---|---|---|---|---|---|---|---|---|---|---|---|---|---|---|
|
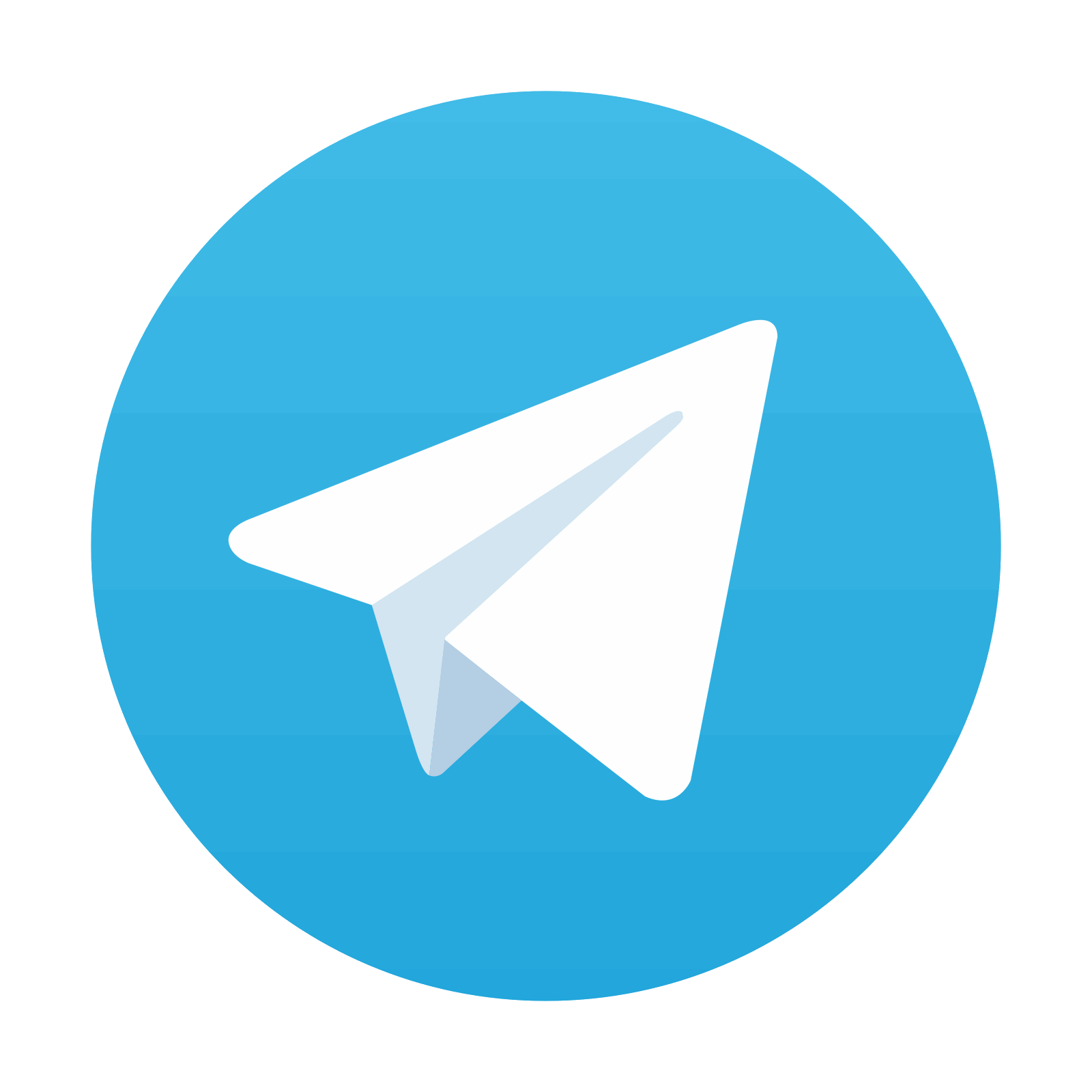
Stay updated, free articles. Join our Telegram channel

Full access? Get Clinical Tree
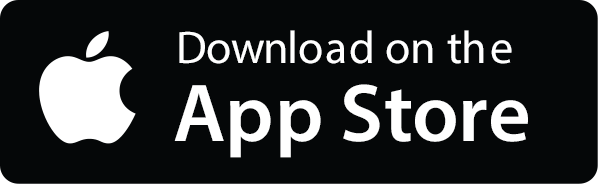
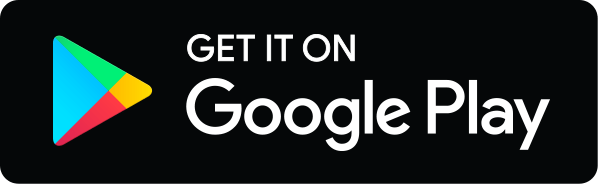