Sign
Pathophysiology
Hypotension
Low vasomotor tone or decreased cardiac output reduce mean arterial pressure
Oliguria/anuria
Decreased renal arterial blood flow
Depressed mental status
Decreased cerebral arterial blood flow
Metabolic/lactic acidosis
Tissue ischemia leading to increased anaerobic metabolism
Elevated liver function tests
Decreased portal and hepatic arterial blood flow
Coagulopathy
Disseminated vascular coagulation secondary to release of tissue factor in the setting of cytokine release or end-organ damage
15.2.1 Differentiation of Shock States
The equation for mean arterial pressure (MAP) vastly simplifies an understanding of shock. Considering MAP as the product of cardiac output (CO) and systemic vascular resistance (SVR), shock represents a perturbation of preload, contractility, systemic vascular resistance, or a combination of the three (Table 15.2). The challenge in differentiating between shock states lies in identifying which of these parameters requires restoration.
Table 15.2
Shock states as functions of mean arterial pressure (MAP = CO*SVR)
Contractility | Preload | SVR | |
---|---|---|---|
Hypovolemic | Early↑ Late↓ | ↓ | ↑ |
Distributive | No change,↑ or↓ | ↓ | ↓ |
Cardiogenic | ↓ | ↑ | ↑ |
Obstructive | Early↑ Late↓ | ↓ | ↑ |
The first step is to assess for preload deficits through a determination of volume responsiveness. The concept of volume responsiveness arises from the Frank-Starling curve (Fig. 15.1). Along the steep slope of the Frank-Starling curve, increases in left ventricular (LV) end-diastolic volume (preload) translate into improved stroke volume (SV) and, therefore, cardiac output. Beyond the inflection point of the Frank-Starling curve, however, the myocardial fibers of the LV are maximally stretched, such that further volume loading does not increase stroke volume. In such patients, intravenous fluid boluses do not improve cardiac output.
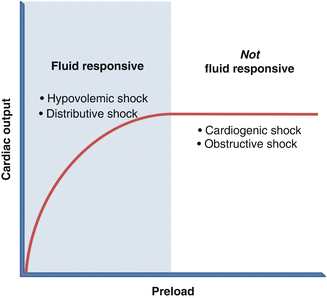
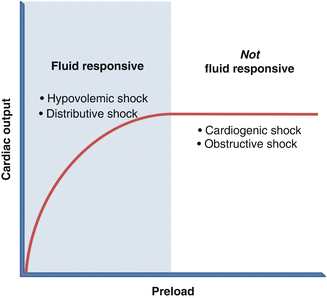
Fig. 15.1
The Frank-Starling curve
In general, patients in shock whose blood pressure rises with volume are volume responsive and have either distributive or hypovolemic shock. In contrast, if fluids have minimal impact on cardiac output, clinicians should suspect cardiogenic or obstructive shock (Fig. 15.1). Note that although patients with obstructive shock have deficient preload, they rarely improve with boluses because the inciting pathology (pneumothorax, pericardial tamponade, etc.) obstructs LV filling.
Administration of intravenous crystalloid is the simplest option to investigate volume responsiveness. However, injudicious fluid resuscitation delivers well-documented detrimental effects, including acute lung injury and compartment syndrome [2–9]. Clinicians should therefore consider an array of additional methods to measure volume responsiveness:
- 1.
Passive leg raise (PLR). Noninvasive and reversible, this method requires lifting the legs of a supine patient 45°, which increases venous return from the lower extremities for an approximate total volume of 200 cc. A 10 % increase in pulse pressure with PLR has 79 % sensitivity and 85 % specificity for volume responsiveness [10]. Drawbacks of the PLR include unreliability in severely hypovolemic patients (in whom total blood volume may be too low to detect a change), as well as in patients with abdominal hypertension.
- 2.
Pulse pressure variation (PPV). Changes in the arterial waveform throughout the cardiac cycle predict volume responsiveness in mechanically ventilated patients. During inspiration, increased intrathoracic pressure decreases venous return, shifting the interventricular septum to the right and improving LV compliance. Return of blood from the pulmonary veins to the left atrium increases, with the net effect of increased preload and, therefore, higher systolic blood pressure at end inspiration. During expiration, the decreased right ventricular (RV) volume seen on end inspiration translates into decreased LV preload, with a resultant drop in systolic blood pressure. Hypovolemia augments this pattern of pulse pressure variation. In mechanically ventilated patients, a PPV of ≥13 % during the respiratory cycle confers 94 % sensitivity and 96 % specificity for volume responsiveness [11, 12].
- 3.
Stroke volume variation (SVV). Recent technology termed pulse contour analysis (PCA) monopolizes upon stroke volume variation during mechanical ventilation to predict volume responsiveness. Although the specific algorithms vary, all PCA devices analyze the area beneath the arterial pressure waveform and correlate these measurements with stroke volume. The PiCCO system (Pulsion Medical Systems, Germany) accomplishes this through thermodilution techniques similar to the Swan-Ganz catheter. The Flo-Trac or Vigileo devices (Edward Lifesciences, USA), meanwhile, sample the waveform at regular intervals and apply statistical models to calculate SVV. The threshold above which SVV predicts volume responsiveness depends upon the device and is more accurate for tidal volumes >8 cc/kg, an important caveat given the low-tidal volume ventilation strategy now commonplace in surgical ICUs [13–15].
- 4.
IVC diameter. Point-of-care ultrasound (POCUS) has garnered enthusiasm over the past decade for its versatility, reproducibility, noninvasiveness, and accuracy in skilled hands. A common metric for fluid responsiveness using ultrasound is variation of inferior vena caval (IVC) diameter with respiration. During positive pressure inspiration, the diameter of the IVC increases. Practitioners can quantify this change with M-mode, and values >18 % correlate with volume responsiveness [16]. In contrast, static recordings of IVC diameter warrant cautious interpretation, as high-tidal volumes, right ventricular failure, and intra-abdominal hypertension confound measurements [16–18].
Although traditionally surrogates for volume status, central venous pressure (CVP), and pulmonary capillary wedge pressure (PCWP) poorly predict fluid responsiveness and should not guide shock workup and therapy [19]. The high and low extremes of these measurements may correlate with hypovolemia and volume overload, respectively, but the vast middle ground of values are inconclusive.
Once tests have confirmed a patient as volume responsive or nonresponsive, further characterization of shock entails careful attention to a patient’s history, physical examination, and diagnostic study results. The algorithm in Fig. 15.2 outlines a basic approach. Point-of-care ultrasound (POCUS) plays an increasingly important role in the diagnosis of shock [20], and as such, Table 15.3 summarizes the key findings. POCUS is an evolving practice, and an in-depth discussion of the technique is beyond the scope of this chapter.
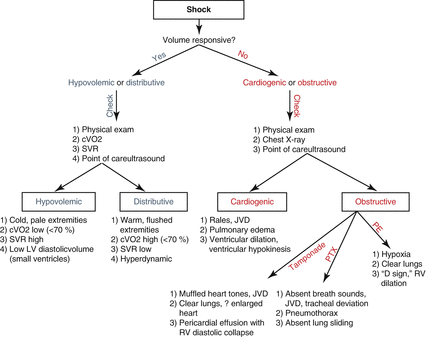
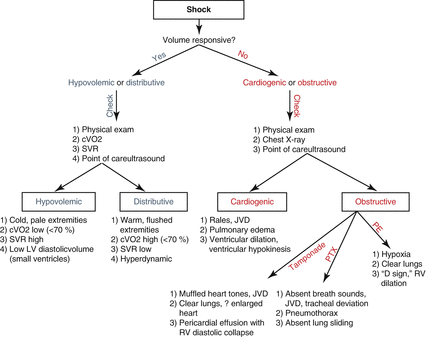
Fig. 15.2
Algorithm for characterization of shock. cVO2 central venous oxygen saturation, SVR systemic vascular resistance, LV left ventricle, JVD jugular venous distension, PTX pneumothorax, PE pulmonary embolus, RV right ventricle
Table 15.3
Point-of-care ultrasound (POCUS) findings in specific shock states
POCUS findings | |
---|---|
Hypovolemic | “Kissing ventricles:” Collapse of ventricular walls during systole Small ventricular diameter during diastole |
Distributive | “Hyperdynamic:” Increased heart rate Increased contractility (early) May have collapse of ventricular walls during systole, but end-diastolic ventricular diameter approximates normal |
Cardiogenic | Dilated, hypokinetic LV |
Obstructive | Tamponade: Pericardial effusion Diastolic collapse of RV Tension pneumothorax: Absent lung sliding Absent B-lines Pulmonary embolus: “D-sign:” flattening of intra-ventricular septum due to RV hypertension, with “D”-shaped appearance of RV on parasternal short axis view Dilated RV |
15.2.2 Management of Shock
The following sections detail the management of specific shock variants. Acute care surgeons frequently encounter both septic (distributive) shock and obstructive shock from abdominal compartment syndrome. This handbook dedicates separate chapters to these topics, and so the ensuing discussion will not duplicate such in-depth coverage.
For all diagnoses discussed below, monitoring of serum lactate, base deficit, and laboratory parameters of end-organ function (creatinine, liver function tests, coagulation factors) can guide therapy.
15.2.2.1 Hemorrhagic Shock
Massive hemorrhage subjects patients to hypothermia, acidosis, and coagulopathy, a “lethal triad” that further potentiates bleeding. Survival depends upon rapid resuscitation with blood products through large-bore access, and correction of these abnormalities [21]. Patients in hemorrhagic shock must be warmed to normothermia, and coagulopathy and acidosis reversed through attentive resuscitation. A growing body of data supports the transfusion of fresh frozen plasma, packed red blood cells, and platelets in a 1:1:1 ratio to approximate whole blood and limit dilutional coagulopathy [22]. Patients with a large transfusion requirement must be closely monitored for electrolyte derangements, particularly hypocalcemia and hyperkalemia, and should undergo serial examinations for the development of compartment syndrome.
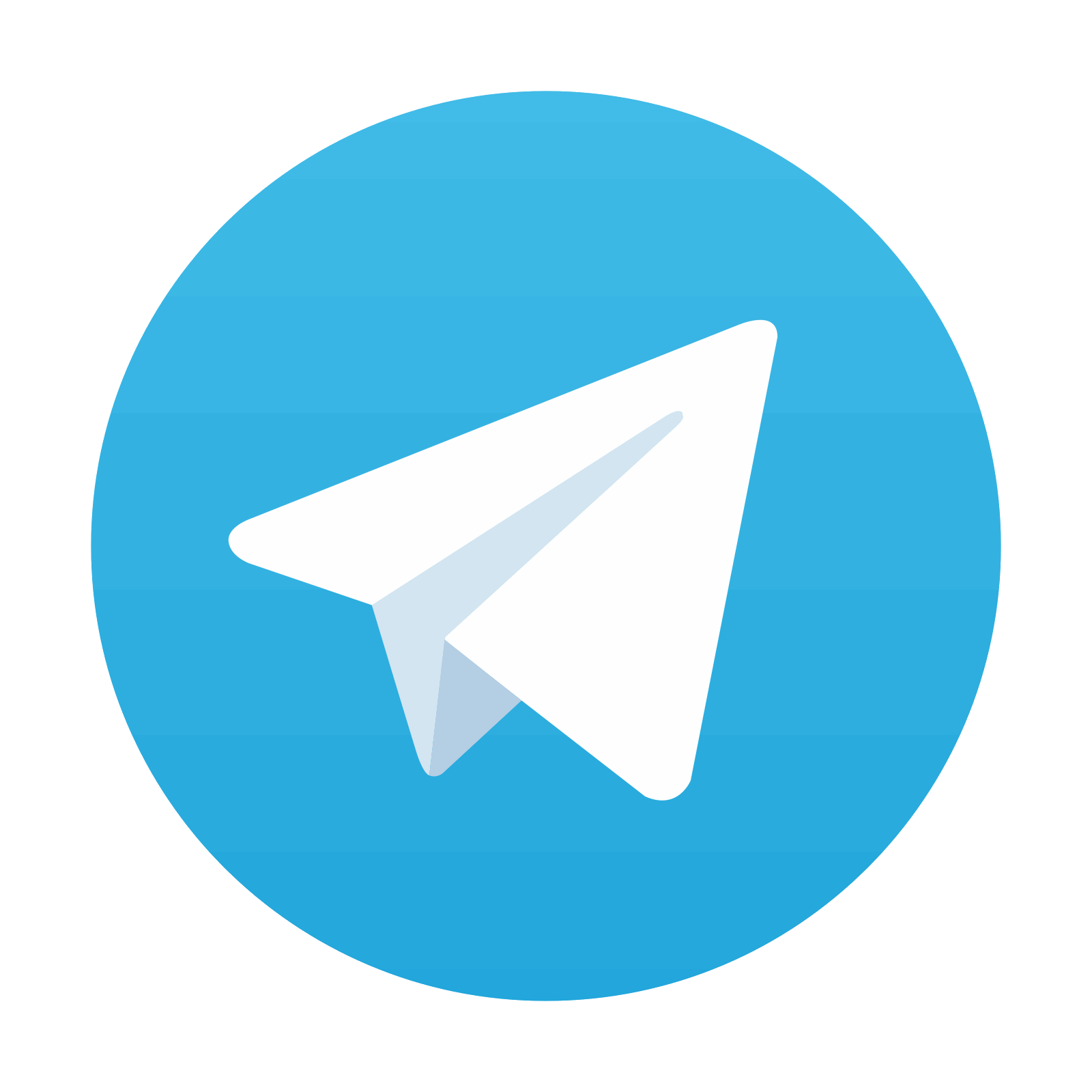
Stay updated, free articles. Join our Telegram channel

Full access? Get Clinical Tree
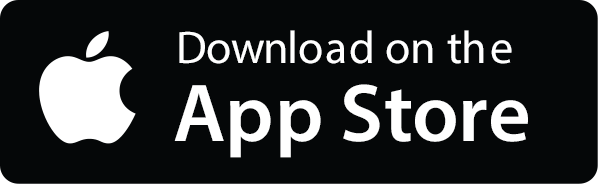
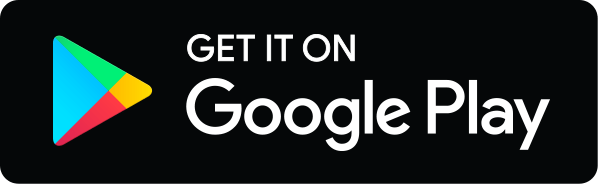