Key Concepts
Diabetic autonomic neuropathy may limit the patient’s ability to compensate (with tachycardia and increased peripheral resistance) for intravascular volume changes and may predispose the patient to cardiovascular instability (eg, postinduction hypotension) and even sudden cardiac death.
Temporomandibular joint and cervical spine mobility should be assessed preoperatively in diabetic patients to reduce the likelihood of unanticipated difficult intubation. Difficult intubation has been reported in as many as 30% of persons with type 1 diabetes.
Sulfonylureas and metformin have long half-lives and many clinicians will discontinue them 24-48 h before surgery. They can be started postoperatively when the patient resumes oral intake.
Incompletely treated hyperthyroid patients can be chronically hypovolemic and prone to an exaggerated hypotensive response during induction of anesthesia.
Clinically hypothyroid patients are more susceptible to the hypotensive effect of anesthetic agents because of their diminished cardiac output, blunted baroreceptor reflexes, and decreased intravascular volume.
Patients with glucocorticoid deficiency must receive adequate steroid replacement therapy during the perioperative period.
In patients with a pheochromocytoma, drugs or techniques that indirectly stimulate or promote the release of catecholamines (eg, ephedrine, hypoventilation, or bolus doses of ketamine), potentiate the arrhythmic effects of catecholamines (classically halothane), or consistently release histamine (eg, large doses of atracurium or morphine sulfate) may precipitate hypertension and are best avoided.
Obese patients may be difficult to intubate as a result of limited mobility of the temporomandibular and atlantooccipital joints, a narrowed upper airway, and a shortened distance between the mandible and sternal fat pads.
The key to anesthetic management of patients with carcinoid syndrome is to avoid anesthetic and surgical techniques or agents that could cause the tumor to release vasoactive substances.
Anesthesia for Patients with Endocrine Disease: Introduction
The underproduction or overproduction of hormones can have dramatic physiological and pharmacological consequences. Therefore, it is not surprising that endocrinopathies affect anesthetic management. This chapter briefly reviews normal physiology and pathophysiology of four endocrine organs: the pancreas, the thyroid, the parathyroids, and the adrenal gland. It also considers obesity and carcinoid syndrome.
The Pancreas
Adults normally secrete approximately 50 units of insulin each day from the β cells of the islets of Langerhans in the pancreas. The rate of insulin secretion is primarily determined by the plasma glucose concentration. Insulin, the most important anabolic hormone, has multiple metabolic effects, including facilitating glucose and potassium entry into adipose and muscle cells; increasing glycogen, protein, and fatty acid synthesis; and decreasing glycogenolysis, gluconeogenesis, ketogenesis, lipolysis, and protein catabolism.
In general, insulin stimulates anabolism, whereas lack of insulin is associated with catabolism and a negative nitrogen balance (Table 34-1).
|
Effects on muscle
|
Effects on fat
|
Diabetes mellitus is characterized by impairment of carbohydrate metabolism caused by an absolute or relative deficiency of insulin or of insulin responsiveness, which leads to hyperglycemia and glycosuria. The diagnosis is based on an elevated fasting plasma glucose greater than 126 mg/dL or glycated hemoglobin (HbA1c) of 6.5% or greater. Values are sometimes reported for blood glucose, which runs 12-15% lower than plasma glucose. Even when testing whole blood, newer glucose meters calculate and display plasma glucose.
Diabetes is classified in multiple ways (Table 34-2). Type 1 (insulin-requiring due to endogenous insulin deficiency) and type 2 (insulin-resistant) diabetes are the most common and well known. Diabetic ketoacidosis (DKA) is associated with type 1 diabetes mellitus, but rarely individuals with DKA appear phenotypically to have type 2 diabetes mellitus. Long-term complications of diabetes include retinopathy, kidney disease, hypertension, coronary artery disease, peripheral and cerebral vascular disease, and peripheral and autonomic neuropathies.
Diagnosis (based on blood glucose level) | |
Fasting | 126 mg/dL (7.0 mmol/L) |
Glucose tolerance test | 200 mg/dL (11.1 mmol/L) |
Classification | |
Type 1 (juvenile) | Absolute insulin deficiency secondary to immune-mediated or idiopathic causes |
Type 2 | Onset in childhood or adulthood secondary to insulin resistance (relative insulin insensitivity) |
Gestational | Onset of disease during pregnancy; may or may not persist postpartum |
There are three life-threatening acute complications of diabetes and its treatment—DKA, hyperosmolar nonketotic coma, and hypoglycemia—in addition to other acute medical problems (such as sepsis) in which the presence of diabetes makes treatment more difficult. Decreased insulin activity allows the catabolism of free fatty acids into ketone bodies (acetoacetate and β-hydroxybutyrate), some of which are weak acids (see Chapter 50). Accumulation of these organic acids results in DKA, an anion-gap metabolic acidosis. DKA can easily be distinguished from lactic acidosis, with which it can coexist; lactic acidosis is identified by elevated plasma lactate (>6 mmol/L) and the absence of urine and plasma ketones (although they can occur concurrently and starvation ketosis may occur with lactic acidosis). Alcoholic ketoacidosis can follow heavy alcohol consumption (binge drinking) in a nondiabetic patient and may include a normal or slightly elevated blood glucose level. Such patients may also have a disproportionate increase in β-hydroxybutyrate compared with acetoacetate, in contrast to those with DKA.
Infection is a common precipitating cause of DKA in a known diabetic patient, and DKA may be the reason that a previously undiagnosed person with type 1 diabetes presents for medical treatment. Clinical manifestations of DKA include tachypnea (respiratory compensation for the metabolic acidosis), abdominal pain, nausea and vomiting, and changes in sensorium. The treatment of DKA should include correcting the often substantial hypovolemia, the hyperglycemia, and the total body potassium deficit. This is typically accomplished with a continuous infusion of isotonic fluids and potassium and an insulin infusion.
The goal for decreasing blood glucose in ketoacidosis should be 75-100 mg/dL/h or 10%/h. Therapy generally begins with an intravenous insulin infusion at 0.1 units/kg/h. DKA patients may be resistant to insulin, and the insulin infusion rate may need to be increased if glucose concentrations do not decrease. As glucose moves intracellularly, so does potassium. Although this can quickly lead to a critical level of hypokalemia if not corrected, overaggressive potassium replacement can lead to an equally life-threatening hyperkalemia. Potassium and blood glucose should be monitored frequently during treatment of DKA.
Several liters of 0.9% saline (1-2 L the first hour, followed by 200-500 mL/h) may be required to correct dehydration in adult patients. When plasma glucose decreases to 250 mg/dL, an infusion of D5W should be added to the insulin infusion to decrease the possibility of hypoglycemia and to provide a continuous source of glucose (with the infused insulin) for eventual normalization of intracellular metabolism. Patients may benefit from precise monitoring of urinary output during initial treatment of DKA.
Bicarbonate is rarely needed to correct severe acidosis (pH < 7.1) as the acidosis corrects with volume expansion and with normalization of the plasma glucose concentration.
Ketoacidosis is not a feature of hyperosmolar nonketotic coma possibly because enough insulin is available to prevent ketone body formation. Instead, a hyperglycemia-induced diuresis leads to dehydration and hyperosmolality. Severe dehydration may eventually lead to kidney failure, lactic acidosis, and a predisposition to form intravascular thromboses. Hyperosmolality (frequently exceeding 360 mOsm/L) induces dehydration of neurons, causing changes in mental status and seizures. Severe hyperglycemia causes a factitious hyponatremia: each 100 mg/dL increase in plasma glucose lowers plasma sodium concentration by 1.6 mEq/L. Treatment includes fluid resuscitation with normal saline, relatively small doses of insulin, and potassium supplementation.
Hypoglycemia in the diabetic patient is the result of an absolute or relative excess of insulin relative to carbohydrate intake and exercise. Furthermore, diabetic patients are incompletely able to counter hypoglycemia despite secreting glucagon or epinephrine (counterregulatory failure). The dependence of the brain on glucose as an energy source makes it the organ most susceptible to episodes of hypoglycemia. If hypoglycemia is not treated, mental status changes can progress from anxiety, lightheadedness, or confusion to convulsions and coma. Systemic manifestations of hypoglycemia result from catecholamine discharge and include diaphoresis, tachycardia, and nervousness. Most of the signs and symptoms of hypoglycemia will be masked by general anesthesia. Although the lower boundary of normal plasma glucose levels is ill-defined, medically important hypoglycemia is present when plasma glucose is less than 50 mg/dL. The treatment of hypoglycemia in anesthetized or critically ill patients consists of intravenous administration of 50% glucose (each milliliter of 50% glucose will raise the blood glucose of a 70-kg patient by approximately 2 mg/dL). Awake patients can be treated orally with fluids containing glucose or sucrose.
Abnormally elevated hemoglobin A1c concentrations identify patients who have maintained poor control of blood glucose over time. These patients may be at greater risk for perioperative hyperglycemia, perioperative complications, and adverse outcomes. The perioperative morbidity of diabetic patients is related to their preexisting end-organ damage. Unfortunately, one third to one half of patients with type 2 diabetes mellitus may be unaware of their condition.
A preoperative chest radiograph in a diabetic patient is more likely to uncover cardiac enlargement, pulmonary vascular congestion, or pleural effusion, but is not routinely indicated. Diabetic patients also have an increased incidence of ST-segment and T-wave-segment abnormalities on preoperative electrocardiograms (ECGs). Myocardial ischemia or old infarction may be evident on an ECG despite a negative history. Diabetic patients with hypertension have a 50% likelihood of coexisting diabetic autonomic neuropathy (Table 34-3). Reflex dysfunction of the autonomic nervous system may be increased by old age, diabetes of longer than 10 years’ duration, coronary artery disease, or β-adrenergic blockade. Diabetic autonomic neuropathy may limit the patient’s ability to compensate (with tachycardia and increased peripheral resistance) for intravascular volume changes and may predispose the patient to cardiovascular instability (eg, postinduction hypotension) and even sudden cardiac death. The incidence of perioperative cardiovascular instability appears increased by the concomitant use of angiotensin-converting enzyme inhibitors or angiotensin receptor blockers. Autonomic dysfunction contributes to delayed gastric emptying (diabetic gastroparesis). Premedication with a nonparticulate antacid and metoclopramide is often used in an obese diabetic patient with signs of cardiac autonomic dysfunction. However, autonomic dysfunction can affect the gastrointestinal tract without any signs of cardiac involvement.
|
Diabetic renal dysfunction is manifested first by proteinuria and later by elevated serum creatinine. By these criteria, most patients with type 1 diabetes have evidence of kidney disease by 30 years of age. Because of an increased incidence of infections related to a compromised immune system, strict attention to aseptic technique, important for all patients, is especially important in those with diabetes.
Chronic hyperglycemia can lead to glycosylation of tissue proteins and limited mobility of joints. Temporomandibular joint and cervical spine mobility should be assessed preoperatively in diabetic patients to reduce the likelihood of unanticipated difficult intubations. Difficult intubation has been reported in as many as 30% of persons with type 1 diabetes.
The goal of intraoperative blood glucose management is to avoid hypoglycemia while maintaining blood glucose below 180 mg/dL. Attempting to maintain strict euglycemia is imprudent; “loose” blood glucose control (>180 mg/dL) also carries risk. The exact range over which blood glucose should be maintained in critical illness has been the subject of several much-discussed clinical trials. Hyperglycemia has been associated with hyperosmolarity, infection, poor wound healing, and increased mortality. Severe hyperglycemia may worsen neurological outcome following an episode of cerebral ischemia and may compromise outcome following cardiac surgery or after an acute myocardial infarction. Unless severe hyperglycemia is treated aggressively in type 1 diabetic patients, metabolic control may be lost, particularly in association with major surgery or critical illness. Maintaining blood glucose control (<180 mg/dL) in patients undergoing cardiopulmonary bypass decreases infectious complications. A benefit of true “tight” control (<150 mg/dL) during surgery or critical illness has not yet been demonstrated convincingly and in some studies has been associated with worse outcome than “looser” control (<180 mg/dL).
Lack of consensus regarding the appropriate target for blood glucose has not prevented perioperative glucose management from becoming yet another indicator of so-called “quality” anesthetic care. Consequently, anesthesia staff should carefully review their current practices to ensure that their glucose management protocols are in line with institutional expectations.
Control of blood glucose in pregnant diabetic patients improves fetal outcome. Nonetheless, as noted earlier, the brain’s dependence on glucose as an energy supply makes it essential that hypoglycemia be avoided.
There are several common perioperative management regimens for insulin-dependent diabetic patients. In the most time-honored (but not terribly effective) approach, the patient receives a fraction—usually half—of the total morning insulin dose in the form of intermediate-acting insulin (Table 34-4). To decrease the risk of hypoglycemia, insulin is administered after intravenous access has been established and the morning blood glucose level is checked. For example, a patient who normally takes 30 units of NPH (neutral protamine Hagedorn; intermediate-acting) insulin and 10 units of regular or Lispro (short-acting) insulin or insulin analogue each morning and whose blood glucose is at least 150 mg/dL would receive 15 units (half the normal 30-unit morning dose) of NPH subcutaneously before surgery along with an infusion of 5% dextrose solution (1.5 mL/kg/h). Absorption of subcutaneous or intramuscular insulin depends on tissue blood flow, however, and can be unpredictable during surgery. Dedication of a small-gauge intravenous line for the dextrose infusion prevents interference with other intraoperative fluids and drugs. Supplemental dextrose can be administered if the patient becomes hypoglycemic (<100 mg/dL). However, intraoperative hyperglycemia (>150-180 mg/dL) is treated with intravenous regular insulin according to a sliding scale. One unit of regular insulin given to an adult usually lowers plasma glucose by 25-30 mg/dL. It must be stressed that these doses are approximations and do not apply to patients in catabolic states (eg, sepsis, hyperthermia).
Bolus Administration | Continuous Infusion | |
---|---|---|
Preoperative | D5W (1.5 mL/kg/h) NPH1 insulin (half usual am dose) | D5W (1 mL/kg/h) Regular insulin: |
Intraoperative | Regular insulin (as per sliding scale) | Same as preoperative |
Postoperative | Same as intraoperative | Same as preoperative |
An alternative method is to administer regular insulin as a continuous infusion. The advantage of this technique is more precise control of insulin delivery than can be achieved with a subcutaneous or intramuscular injection of NPH insulin, particularly in conditions associated with poor skin and muscle perfusion. Regular insulin can be added to normal saline in a concentration of 1 unit/mL and the infusion begun at 0.1 unit/kg/h. As blood glucose fluctuates, the regular insulin infusion can be adjusted up or down as required. The dose required may be approximated by the following formula:
A general target for the intraoperative maintenance of blood glucose is less than 180 mg/dL. The tighter control afforded by a continuous intravenous technique may be preferable in patients with type 1 diabetes.
When administering an intravenous insulin infusion to surgical patients, adding some (eg, 20 mEq) KCl to each liter of fluid may be useful, as insulin causes an intracellular potassium shift. Because individual insulin needs can vary dramatically, any formula should be considered as only a crude guideline.
If the patient is taking an oral hypoglycemic agent preoperatively rather than insulin, the drug can be continued until the day of surgery. However, sulfonylureas and metformin have long half-lives and many clinicians will discontinue them 24-48 h before surgery. They can be started postoperatively when the patient resumes oral intake. Metformin is restarted if renal and hepatic function remain adequate. The effects of oral hypoglycemic drugs with a short duration of action can be prolonged in the presence of kidney failure. Many patients maintained on oral antidiabetic agents will require insulin treatment during the intraoperative and postoperative periods. The stress of surgery causes elevations in counterregulatory hormones (eg, catecholamines, glucocorticoids, growth hormone) and inflammatory mediators such as tumor necrosis factor and interleukins. Each of these contributes to stress hyperglycemia, which increases insulin requirements. In general, type 2 diabetic patients tolerate minor, brief surgical procedures without any exogenous insulin. However, many ostensibly “nondiabetic” patients show pronounced hyperglycemia during critical illness and require a period of insulin therapy.
The key to any management regimen is to monitor plasma glucose levels frequently. Patients receiving insulin infusions intraoperatively may need to have their glucose measured hourly. Those with type 2 diabetes vary in their ability to produce and respond to endogenous insulin, and measurement every 2 or 3 h may be sufficient. Likewise, insulin requirements vary with the extensiveness of the surgical procedure. Bedside glucose meters are capable of determining the glucose concentration in a drop of blood obtained from a finger stick (or withdrawn from a central or arterial line) within a minute. These devices measure the color conversion of a glucose oxidase-impregnated strip. Their accuracy depends, to a large extent, on adherence to the device’s specific testing protocol. Monitoring urine glucose is of value only for detecting glycosuria.
Patients who take NPH or other protamine-containing insulin preparations have an increased risk of allergic reactions to protamine sulfate—including anaphylactoid reactions and death. Unfortunately, operations that require the use of heparin and subsequent reversal with protamine (eg, cardiopulmonary bypass) are more common in diabetic patients. The usefulness of a small protamine test dose of 1-5 mg over 5-10 min prior to the full reversal dose is unclear, although this is recommended by some clinicians.
Patients who use subcutaneous insulin infusion pumps for management of type 1 diabetes usually can leave the pump programmed to deliver “basal” amounts of regular insulin (or insulin glargine). This is the amount of insulin required during fasting. Such patients can safely undergo short outpatient surgery with the pump on the basal setting. If more extensive inpatient procedures are required, these patients will normally be managed with intravenous insulin infusions as described earlier.
Close monitoring of blood glucose must continue postoperatively. There is considerable patient-to-patient variation in onset and duration of action of insulin preparations (Table 34-5). For example, the onset of action of subcutaneous regular insulin is less than 1 h, but in rare patients its duration of action may continue for 6 h. NPH insulin typically has an onset of action within 2 h, but the action can last longer than 24 h. Another reason for close monitoring is the progression of stress hyperglycemia in the recovery period.
Insulin Type2 | Onset | Peak Action | Duration | |
---|---|---|---|---|
Short-acting |
|
|
|
|
Intermediate-acting | Lente, Lentard, NPH | 2-4 h | 8-10 h | 18-24 h |
Long-acting | Ultralente, Glargine, Insulatard | 4-5 h | 8-14 h | 25-36 h |
The Thyroid
Dietary iodine is absorbed by the gastrointestinal tract, converted to iodide ion, and actively transported into the thyroid gland. Once inside, iodide is oxidized back to iodine, which is bound to the amino acid tyrosine. The end result is two hormones—triiodothyronine (T3) and thyroxine (T4)—which are bound to proteins and stored within the thyroid. Although the gland releases more T4 than T3, the latter is more potent and less protein bound. Of all circulating T3, most is formed peripherally from partial deiodination of T4
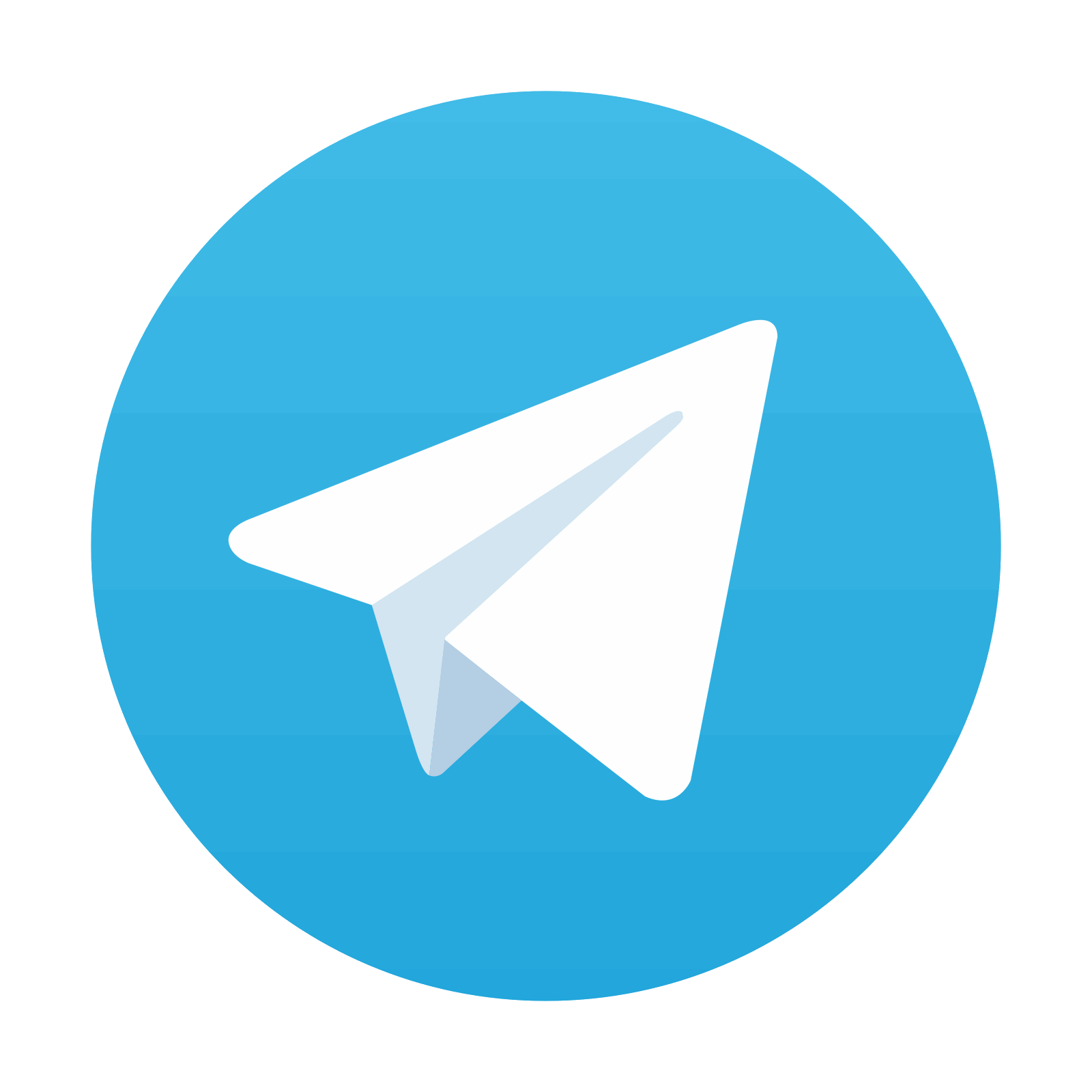
Stay updated, free articles. Join our Telegram channel

Full access? Get Clinical Tree
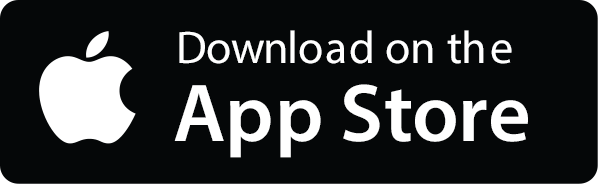
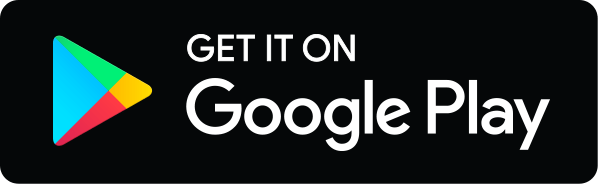
