Key Concepts
The central venous pressure catheter’s tip should not be allowed to migrate into the heart chambers.
Although the pulmonary artery catheter can be used to guide goal-directed hemodynamic therapy to ensure organ perfusion in shock states, other less invasive methods to determine hemodynamic performance are available, including transpulmonary thermodilution cardiac output measurements and pulse contour analyses of the arterial pressure waveform.
Relative contraindications to pulmonary artery catheterization include left bundle-branch block (because of the concern about complete heart block) and conditions associated with greatly increased risk of arrhythmias, such as Wolff-Parkinson-White syndrome.
Pulmonary artery pressure should be continuously monitored to detect an overwedged position indicative of catheter migration.
Accurate measurements of cardiac output depend on rapid and smooth injection, precisely known injectant temperature and volume, correct entry of the calibration factors for the specific type of pulmonary artery catheter into the cardiac output computer, and avoidance of measurements during electrocautery.
Cardiovascular Monitoring: Introduction
Arterial Blood Pressure
The rhythmic contraction of the left ventricle, ejecting blood into the vascular system, results in pulsatile arterial pressures. The peak pressure generated during systolic contraction (in the absence of aortic valve stenosis) approximates the systolic arterial blood pressure (SBP); the lowest arterial pressure during diastolic relaxation is the diastolic blood pressure (DBP). Pulse pressure is the difference between the systolic and diastolic pressures. The time-weighted average of arterial pressures during a pulse cycle is the mean arterial pressure (MAP). MAP can be estimated by application of the following formula:
Arterial blood pressure is greatly affected by where the pressure is measured. As a pulse moves peripherally through the arterial tree, wave reflection distorts the pressure waveform, leading to an exaggeration of systolic and pulse pressures (Figure 5-1). For example, radial artery systolic pressure is usually greater than aortic systolic pressure because of its more distal location. In contrast, radial artery systolic pressures often underestimate more “central” pressures following hypothermic cardiopulmonary bypass because of changes in hand vascular resistance. Vasodilating drugs may accentuate this discrepancy. The level of the sampling site relative to the heart affects the measurement of blood pressure because of the effect of gravity (Figure 5-2). In patients with severe peripheral vascular disease, there may be a significant difference in blood pressure measurements among the extremities. The higher value should be used in these patients.
Figure 5-1
Changes in configuration as a waveform moves peripherally. (Reproduced, with permission, from Shah N, Bedford RF: Invasive and noninvasive blood pressuring monitoring. In: Clinical Monitoring: Practical Applications in Anesthesia and Critical Care Medicine. Lake CL, Hines RL, Blitt CD [editors]. WB Saunders, Philadelphia, 2001.)
Because noninvasive (palpation, Doppler, auscultation, oscillometry, plethysmography) and invasive (arterial cannulation) methods of blood pressure determination differ greatly, they are discussed separately.
The use of any anesthetic, no matter how “trivial,” is an indication for arterial blood pressure measurement. The techniques and frequency of pressure determination depend on the patient’s condition and the type of surgical procedure. An oscillometric blood pressure measurement every 3-5 min is adequate in most cases.
Although some method of blood pressure measurement is mandatory, techniques that rely on a blood pressure cuff are best avoided in extremities with vascular abnormalities (eg, dialysis shunts) or with intravenous lines. Rarely, it may prove impossible to monitor blood pressure in cases (eg, burns) in which there may be no accessible site from which the blood pressure can be safely recorded.
SBP can be determined by (1) locating a palpable peripheral pulse, (2) inflating a blood pressure cuff proximal to the pulse until flow is occluded, (3) releasing cuff pressure by 2 or 3 mm Hg per heartbeat, and (4) measuring the cuff pressure at which pulsations are again palpable. This method tends to underestimate systolic pressure, however, because of the insensitivity of touch and the delay between flow under the cuff and distal pulsations. Palpation does not provide a diastolic pressure or MAP. The equipment required is simple and inexpensive.
When a Doppler probe is substituted for the anesthesiologist’s finger, arterial blood pressure measurement becomes sensitive enough to be useful in obese patients, pediatric patients, and patients in shock (Figure 5-3). The Doppler effect is the shift in the frequency of sound waves when their source moves relative to the observer. For example, the pitch of a train’s whistle increases as a train approaches and decreases as it departs. Similarly, the reflection of sound waves off of a moving object causes a frequency shift. A Doppler probe transmits an ultrasonic signal that is reflected by underlying tissue. As red blood cells move through an artery, a Doppler frequency shift will be detected by the probe. The difference between transmitted and received frequency causes the characteristic swishing sound, which indicates blood flow. Because air reflects ultrasound, a coupling gel (but not corrosive electrode jelly) is applied between the probe and the skin. Positioning the probe directly above an artery is crucial, since the beam must pass through the vessel wall. Interference from probe movement or electrocautery is an annoying distraction. Note that only systolic pressures can be reliably determined with the Doppler technique.
A variation of Doppler technology uses a piezoelectric crystal to detect lateral arterial wall movement to the intermittent opening and closing of vessels between systolic and diastolic pressure. This instrument thus detects both systolic and diastolic pressures. The Doppler effect is routinely employed by perioperative echocardiographers to discern both the directionality and velocity of both blood flow within the heart and the movement of the heart’s muscle tissue (tissue Doppler).
Inflation of a blood pressure cuff to a pressure between systolic and diastolic pressures will partially collapse an underlying artery, producing turbulent flow and the characteristic Korotkoff sounds. These sounds are audible through a stethoscope placed under—or just distal to—the distal third of the blood pressure cuff. The clinician measures pressure with an aneroid or mercury manometer.
Occasionally, Korotkoff sounds cannot be heard through part of the range from systolic to diastolic pressure. This auscultatory gap is most common in hypertensive patients and can lead to an inaccurate diastolic pressure measurement. Korotkoff sounds are often difficult to auscultate during episodes of hypotension or marked peripheral vasoconstriction. In these situations, the subsonic frequencies associated with the sounds can be detected by a microphone and amplified to indicate systolic and diastolic pressures. Motion artifact and electrocautery interference limit the usefulness of this method.
Arterial pulsations cause oscillations in cuff pressure. These oscillations are small if the cuff is inflated above systolic pressure. When the cuff pressure decreases to systolic pressure, the pulsations are transmitted to the entire cuff, and the oscillations markedly increase. Maximal oscillation occurs at the MAP, after which oscillations decrease. Because some oscillations are present above and below arterial blood pressure, a mercury or aneroid manometer provides an inaccurate and unreliable measurement. Automated blood pressure monitors electronically measure the pressures at which the oscillation amplitudes change (Figure 5-4). A microprocessor derives systolic, mean, and diastolic pressures using an algorithm. Machines that require identical consecutive pulse waves for measurement confirmation may be unreliable during arrhythmias (eg, atrial fibrillation). Oscillometric monitors should not be used on patients on cardiopulmonary bypass. Nonetheless, the speed, accuracy, and versatility of oscillometric devices have greatly improved, and they have become the preferred noninvasive blood pressure monitors in the United States and worldwide.
Arterial tonometry measures beat-to-beat arterial blood pressure by sensing the pressure required to partially flatten a superficial artery that is supported by a bony structure (eg, radial artery). A tonometer consisting of several independent pressure transducers is applied to the skin overlying the artery (Figure 5-5). The contact stress between the transducer directly over the artery and the skin reflects intraluminal pressure. Continuous pulse recordings produce a tracing very similar to an invasive arterial blood pressure waveform. Limitations to this technology include sensitivity to movement artifact and the need for frequent calibration.
Adequate oxygen delivery to vital organs must be maintained during anesthesia. Unfortunately, instruments to monitor specific organ perfusion and oxygenation are complex, expensive, and often unreliable, and, for that reason, an adequate arterial blood pressure is assumed to predict adequate organ blood flow. However, flow also depends on vascular resistance:
Even if the pressure is high, when the resistance is also high, flow can be low. Thus, arterial blood pressure should be viewed as an indicator—but not a measure—of organ perfusion.
The accuracy of any method of blood pressure measurement that involves a blood pressure cuff depends on proper cuff size (Figure 5-6). The cuff’s bladder should extend at least halfway around the extremity, and the width of the cuff should be 20% to 50% greater than the diameter of the extremity.
Figure 5-6
Blood pressure cuff width influences the pressure readings. Three cuffs, all inflated to the same pressure, are shown. The narrowest cuff (A) will require more pressure, and the widest cuff (C) less pressure, to occlude the brachial artery for determination of systolic pressure. Too narrow a cuff may produce a large overestimation of systolic pressure. Whereas the wider cuff may underestimate the systolic pressure, the error with a cuff 20% too wide is not as significant as the error with a cuff 20% too narrow. (Reproduced, with permission, from Gravenstein JS, Paulus DA: Clinical Monitoring Practice, 2nd ed. Lippincott, Philadelphia, 1987.)
Automated blood pressure monitors, using one or a combination of the methods described above, are frequently used in anesthesiology. A self-contained air pump inflates the cuff at set intervals. Incorrect or too frequent use of these automated devices has resulted in nerve palsies and extensive extravasation of intravenously administered fluids. In case of equipment failure, an alternative method of blood pressure determination must be immediately available.
Indications for invasive arterial blood pressure monitoring by catheterization of an artery include induced current or anticipated hypotension or wide blood pressure deviations, end-organ disease necessitating precise beat-to-beat blood pressure regulation, and the need for multiple arterial blood gas measurements.
If possible, catheterization should be avoided in smaller end arteries with inadequate collateral blood flow or in extremities where there is a suspicion of preexisting vascular insufficiency.
Several arteries are available for percutaneous catheterization.
The radial artery is commonly cannulated because of its superficial location and substantial collateral flow (in most patients the ulnar artery is larger than the radial and there are connections between the two via the palmar arches). Five percent of patients have incomplete palmar arches and lack adequate collateral blood flow. Allen’s test is a simple, but not reliable, method for assessing the safety of radial artery cannulation. In this test, the patient exsanguinates his or her hand by making a fist. While the operator occludes the radial and ulnar arteries with fingertip pressure, the patient relaxes the blanched hand. Collateral flow through the palmar arterial arch is confirmed by flushing of the thumb within 5 sec after pressure on the ulnar artery is released. Delayed return of normal color (5-10 s) indicates an equivocal test or insufficient collateral circulation (>10 s). The Allen’s test is of such questionable utility that many practitioners routinely avoid it. Alternatively, blood flow distal to the radial artery occlusion can be detected by palpation, Doppler probe, plethysmography, or pulse oximetry. Unlike Allen’s test, these methods of determining the adequacy of collateral circulation do not require patient cooperation.
Ulnar artery catheterization is usually more difficult than radial catheterization because of the ulnar artery’s deeper and more tortuous course. Because of the risk of compromising blood flow to the hand, ulnar catheterization would not normally be considered if the ipsilateral radial artery has been punctured but unsuccessfully cannulated.
The brachial artery is large and easily identifiable in the antecubital fossa. Its proximity to the aorta provides less waveform distortion. However, being near the elbow predisposes brachial artery catheters to kinking.
The femoral artery is prone to atheroma formation and pseudoaneurysm, but often provides an excellent access. The femoral site has been associated with an increased incidence of infectious complications and arterial thrombosis. Aseptic necrosis of the head of the femur is a rare, but tragic, complication of femoral artery cannulation in children.
The dorsalis pedis and posterior tibial arteries are some distance from the aorta and therefore have the most distorted waveforms.
The axillary artery is surrounded by the axillary plexus, and nerve damage can result from a hematoma or traumatic cannulation. Air or thrombi can quickly gain access to the cerebral circulation during vigorous retrograde flushing of axillary artery catheters.
One technique of radial artery cannulation is illustrated in Figure 5-7. Supination and extension of the wrist provide optimal exposure of the radial artery. The pressure-tubing-transducer system should be nearby and already flushed with saline to ensure easy and quick connection after cannulation. The radial pulse is palpated, and the artery’s course is determined by lightly pressing the tips of the index and middle fingers of the anesthesiologist’s nondominant hand over the area of maximal impulse or by use of ultrasound. Using aseptic technique, 1% lidocaine is infiltrated in the skin of awake patients, directly above the artery, with a small gauge needle. A larger gauge needle can then be used as a skin punch, facilitating entry of an 18-, 20-, or 22-gauge catheter over a needle through the skin at a 45° angle, directing it toward the point of palpation. Upon blood flashback, a guidewire may be advanced through the catheter into the artery and the catheter advanced over the guidewire. Alternatively, the needle is lowered to a 30° angle and advanced another 1-2 mm to make certain that the tip of the catheter is well into the vessel lumen. The catheter is advanced off the needle into the arterial lumen, after which the needle is withdrawn. Applying firm pressure over the artery proximal to the catheter tip, with the middle and ring fingertips, prevents blood from spurting from the catheter while the tubing is connected. Waterproof tape or suture can be used to hold the catheter in place.
Figure 5-7
Cannulation of the radial artery. A: Proper positioning and palpation of the artery are crucial. After skin preparation, local anesthetic is infiltrated with a 25-gauge needle. B: A 20- or 22-gauge catheter is advanced through the skin at a 45° angle. C: Flashback of blood signals entry into the artery, and the catheter-needle assembly is lowered to a 30° angle and advanced 1-2 mm to ensure an intraluminal catheter position. D: The catheter is advanced over the needle, which is withdrawn. E: Proximal pressure with middle and ring fingers prevents blood loss, while the arterial tubing Luer-lock connector is secured to the intraarterial catheter.
Complications of intraarterial monitoring include hematoma, bleeding (particularly with catheter tubing disconnections), vasospasm, arterial thrombosis, embolization of air bubbles or thrombi, pseudoaneurysm formation, necrosis of skin overlying the catheter, nerve damage, infection, necrosis of extremities or digits, and unintentional intraarterial drug injection. Factors associated with an increased rate of complications include prolonged cannulation, hyperlipidemia, repeated insertion attempts, female gender, extracorporeal circulation, the use of larger catheters in smaller vessels, and the use of vasopressors. The risks are minimized when the ratio of catheter to artery size is small, saline is continuously infused through the catheter at a rate of 2-3 mL/hr, flushing of the catheter is limited, and meticulous attention is paid to aseptic technique. Adequacy of perfusion can be continually monitored during radial artery cannulation by placing a pulse oximeter on an ipsilateral finger.
Because intraarterial cannulation allows continuous beat-to-beat blood pressure measurement, it is considered the optimal blood pressure monitoring technique. The quality of the transduced waveform, however, depends on the dynamic characteristics of the catheter-tubing-transducer system (Figure 5-8). False readings can lead to inappropriate therapeutic interventions.
A complex waveform, such as an arterial pulse wave, can be expressed as a summation of simple harmonic waves (according to the Fourier theorem). For accurate measurement of pressure, the catheter-tubing-transducer system must be capable of responding adequately to the highest frequency of the arterial waveform (Figure 5-9). Stated another way, the natural frequency of the measuring system must exceed the natural frequency of the arterial pulse (approximately 16-24 Hz).
Figure 5-9
An original waveform overlays a four-harmonic reconstruction (left) and an eight-harmonic reconstruction (right). Note that the higher harmonic plot more closely resembles the original waveform. (Reproduced, with permission, from Saidman LS, Smith WT: Monitoring in Anesthesia. Butterworth-Heinemann, 1985.)
Most transducers have frequencies of several hundred Hz (>200 Hz for disposable transducers). The addition of tubing, stopcocks, and air in the line all decrease the frequency of the system. If the frequency response is too low, the system will be overdamped and will not faithfully reproduce the arterial waveform, underestimating the systolic pressure. Underdamping is also a serious problem, leading to overshoot and a falsely high SBP.
Catheter-tubing-transducer systems must also prevent hyperresonance, an artifact caused by reverberation of pressure waves within the system. A damping coefficient (β) of 0.6-0.7 is optimal. The natural frequency and damping coefficient can be determined by examining tracing oscillations after a high-pressure flush (Figure 5-10).
System dynamics are improved by minimizing tubing length, eliminating unnecessary stopcocks, removing air bubbles, and using low-compliance tubing. Although smaller diameter catheters lower natural frequency, they improve underdampened systems and are less apt to result in vascular complications. If a large catheter totally occludes an artery, reflected waves can distort pressure measurements.
Pressure transducers have evolved from bulky, reusable instruments to miniaturized, disposable devices. Transducers contain a diaphragm that is distorted by an arterial pressure wave. The mechanical energy of a pressure wave is converted into an electric signal. Most transducers are resistance types that are based on the strain gauge principle: stretching a wire or silicone crystal changes its electrical resistance. The sensing elements are arranged as a “Wheatstone bridge” circuit so that the voltage output is proportionate to the pressure applied to the diaphragm (Figure 5-11).
Figure 5-11
In the original strain gauge pressure transducers, a deformable diaphragm was connected to a Wheatstone bridge. When pressure was applied to the diaphragm, strain on two of the resistors (No. 2 and No. 3) increased, whereas strain on the other two (No. 1 and No. 4) decreased. The change in total resistance across the bridge was proportional to the change in blood pressure, allowing direct accurate measurement of intravascular blood pressure for the first time.
Transducer accuracy depends on correct calibration and zeroing procedures. A stopcock at the level of the desired point of measurement—usually the midaxillary line—is opened, and the zero trigger on the monitor is activated. If the patient’s position is altered by raising or lowering the operating table, the transducer must either be moved in tandem or zeroed to the new level of the midaxillary line. In a seated patient, the arterial pressure in the brain differs significantly from left ventricular pressure. In this circumstance, cerebral pressure is determined by setting the transducer to zero at the level of the ear, which approximates the circle of Willis. The transducer’s zero should be checked regularly, as some transducer measurements can “drift” over time.
External calibration of a transducer compares the transducer’s reading with a manometer, but modern transducers rarely require external calibration.
Digital readouts of systolic and diastolic pressures are a running average of the highest and lowest measurements within a certain time interval. Because motion or cautery artifacts can result in some very misleading numbers, the arterial waveform should always be monitored. The shape of the arterial wave provides clues to several hemodynamic variables. The rate of upstroke indicates contractility, the rate of downstroke indicates peripheral vascular resistance, and exaggerated variations in size during the respiratory cycle suggest hypovolemia. MAP is calculated by integrating the area under the pressure curve.
Intraarterial catheters also provide access for intermittent arterial blood gas sampling and analysis. The development of fiberoptic sensors that can be inserted through a 20-gauge arterial catheter enables continuous blood gas monitoring. Unfortunately, these sensors are quite expensive and are often inaccurate, so they are rarely used. Analysis of the arterial pressure waveform allows for estimation of cardiac output (CO) and other hemodynamic parameters. These devices are discussed in the section on CO monitoring.
Electrocardiography
All patients should have intraoperative monitoring of their electrocardiogram (ECG). There are no contraindications.
Lead selection determines the diagnostic sensitivity of the ECG. ECG leads are positioned on the chest and extremities to provide different perspectives of the electrical potentials generated by the heart. At the end of diastole, the atria contract, which provides the atrial contribution to CO, generating the “P” wave. Following atrial contraction, the ventricle is loaded awaiting systole. The QRS complex begins the electrical activity of systole following the 120-200 msec atrioventricular (AV) nodal delay. Depolarization of the ventricle proceeds from the AV node through the interventricular system via the His-Purkinje fibers. The normal QRS lasts approximately 120 msec, which can be prolonged in patients with cardiomyopathies and heart failure. The T wave represents repolarization as the heart prepares to contract again. Prolongation of the QT interval secondary to electrolyte imbalances or drug effects can potentially lead to life-threatening arrhythmias (les torsade de pointes).
The electrical axis of lead II is approximately 60° from the right arm to the left leg, which is parallel to the electrical axis of the atria, resulting in the largest P-wave voltages of any surface lead. This orientation enhances the diagnosis of arrhythmias and the detection of inferior wall ischemia. Lead V5 lies over the fifth intercostal space at the anterior axillary line; this position is a good compromise for detecting anterior and lateral wall ischemia. A true V5 lead is possible only on operating room ECGs with at least five lead wires, but a modified V5 can be monitored by rearranging the standard three-limb lead placement (Figure 5-12). Ideally, because each lead provides unique information, leads II and V5 should be monitored simultaneously. If only a single-channel machine is available, the preferred lead for monitoring depends on the location of any prior infarction or ischemia. Esophageal leads are even better than lead II for arrhythmia diagnosis, but have not yet gained general acceptance in the operating room.
Figure 5-12
Rearranged three-limb lead placement. Anterior and lateral ischemia can be detected by placing the left arm lead (LA) at the V5 position. When lead I is selected on the monitor, a modified V5 lead (CS5) is displayed. Lead II allows detection of arrhythmias and inferior wall ischemia. RA, right arm; LL, left leg.
Electrodes are placed on the patient’s body to monitor the ECG (Figure 5-13). Conductive gel lowers the skin’s electrical resistance, which can be further decreased by cleansing the site with alcohol. Needle electrodes are used only if the disks are unsuitable (eg, with an extensively burned patient).
The ECG is a recording of the electrical potentials generated by myocardial cells. Its routine use allows arrhythmias, myocardial ischemia, conduction abnormalities, pacemaker malfunction, and electrolyte disturbances to be detected (Figure 5-14). Because of the small voltage potentials being measured, artifacts remain a major problem. Patient or lead-wire movement, use of electrocautery, 60-cycle interference from nearby alternating current devices, and faulty electrodes can simulate arrhythmias. Monitoring filters incorporated into the amplifier to reduce “motion” artifacts will lead to distortion of the ST segment and may impede the diagnosis of ischemia. Digital readouts of the heart rate (HR) may be misleading because of monitor misinterpretation of artifacts or large T waves—often seen in pediatric patients—as QRS complexes.
Depending on equipment availability, a preinduction rhythm strip can be printed or frozen on the monitor’s screen to compare with intraoperative tracings. To interpret ST-segment changes properly, the ECG must be standardized so that a 1-mV signal results in a deflection of 10 mm on a standard strip monitor. Newer units continuously analyze ST segments for early detection of myocardial ischemia. Automated ST-segment analysis increases the sensitivity of ischemia detection, does not require additional physician skill or vigilance, and may help diagnose intraoperative myocardial ischemia.
Commonly accepted criteria for diagnosing myocardial ischemia require that the ECG be recorded in “diagnostic mode” and include a flat or downsloping ST-segment depression exceeding 1 mm, 80 msec after the J point (the end of the QRS complex), particularly in conjunction with T-wave inversion. ST-segment elevation with peaked T waves can also represent ischemia. Wolff-Parkinson-White syndrome, bundle-branch blocks, extrinsic pacemaker capture, and digoxin therapy may preclude the use of ST-segment information. The audible beep associated with each QRS complex should be loud enough to detect rate and rhythm changes when the anesthesiologist’s visual attention is directed elsewhere. Some ECGs are capable of storing aberrant QRS complexes for further analysis, and some can even interpret and diagnose arrhythmias. The interference caused by electrocautery units, however, has limited the usefulness of automated arrhythmia analysis in the operating room.
Central Venous Catheterization
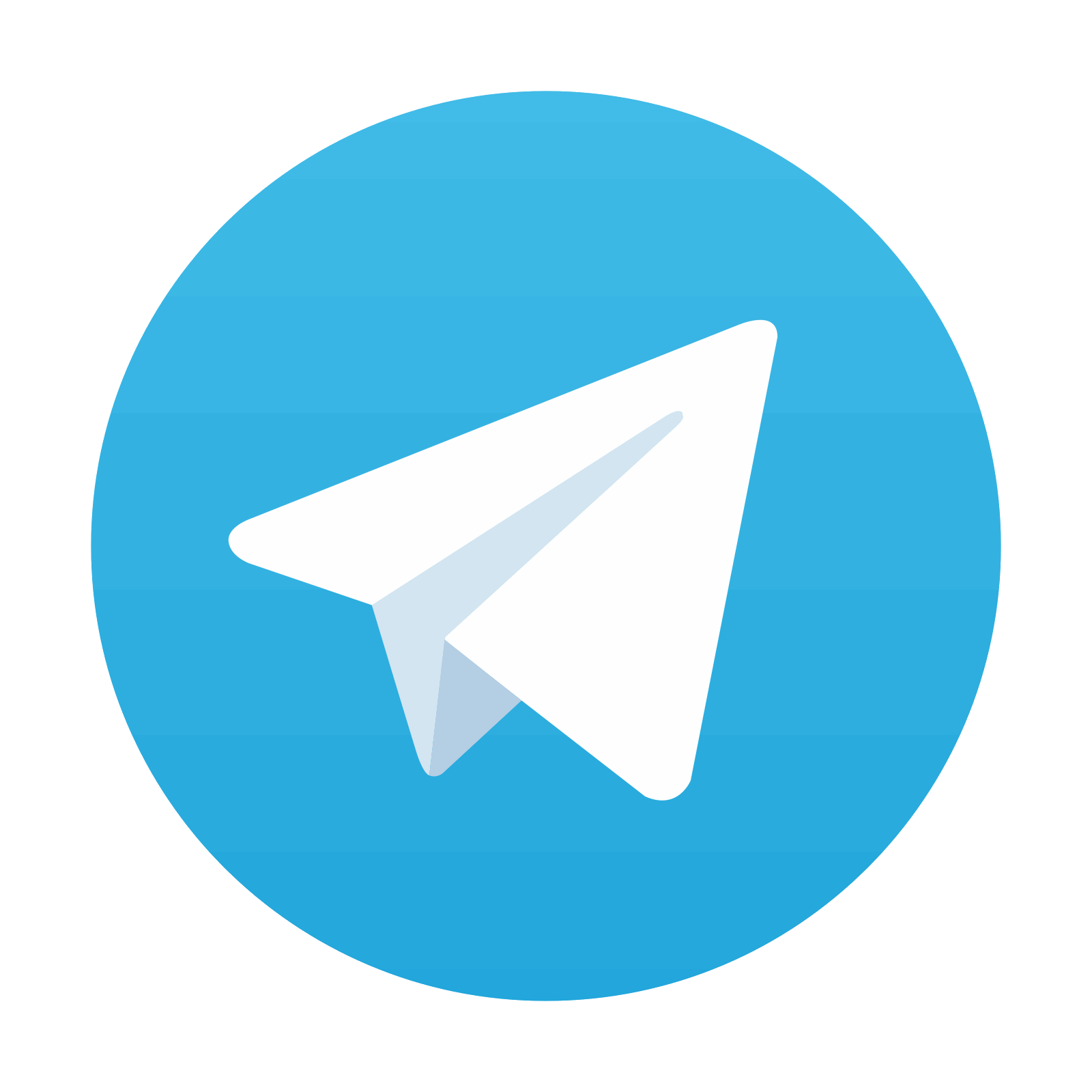
Stay updated, free articles. Join our Telegram channel

Full access? Get Clinical Tree
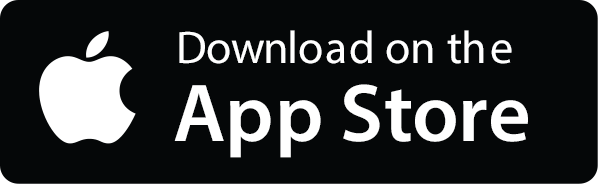
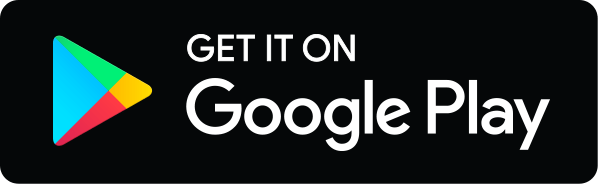