ACUTE RESPIRATORY DYSFUNCTION
The primary functions of the respiratory system are to adequately deliver oxygen from the external environment to meet the metabolic demands of tissues and eliminate the buildup of carbon dioxide. The acute care surgeon is faced with a number of injuries and acute illnesses that impair pulmonary function. This chapter focuses on acute respiratory dysfunction and the common conditions and treatment strategies important for the acute care surgeon.
PHYSIOLOGY OF RESPIRATION
The two main functions of the respiratory system are ventilation to eliminate carbon dioxide and oxygenation to deliver oxygen to the tissues. Efficiency of ventilation is achieved by matching ventilation to perfusion in the gas-exchange regions of the lung. Minute ventilation is calculated by multiplying the tidal volume by the respiratory rate and can be directly measured by collecting exhaled gas over a measured time. Higher minute ventilation represents greater carbon dioxide release. Normal minute ventilation volume is 5–8 L/minute for a 70 kg man. Increased cellular metabolism in response to injury results in increased CO2 production, which must be matched by increased release from the lungs. Normally, a resting adult must eliminate 200 mL/kg/minute of CO2. In the hypermetabolic state, CO2 production increases to the range of 425 mL/kg/minute.1 The minute ventilation required to maintain eucapnia rises concurrently from approximately 5 L/minute (resting rate) to 10 L/minute. This represents a 100% increase in the work of breathing required to meet metabolic demands under these circumstances.
Oxygenation is primarily dependent on binding to hemoglobin (Hb). Hb is composed of four polypeptide chains including two alpha and two beta chains totaling 146 amino acids. Each polypeptide contains an iron atom on the heme group that is capable of binding oxygen. For each gram of Hb, 1.39 mL of O2 can be stored. Arterial O2 content (CaO2) is related to Hb concentration and Hb saturation (SaO2) by the following equation2:
The oxygen dissociation curve seen (Fig. 47.1) shows the percent saturation of Hb at various partial pressures of oxygen. As erythrocytes travel to areas with low oxygen saturation, the partial pressure of oxygen decreases leading to delivery of oxygen to tissue. Temperature, organic phosphates such as DPG and pH directly affect Hb affinity for oxygen and oxygen delivery.
FIGURE 47.1. Oxygen dissociation curve.
Obstructive airway disease such as chronic obstructive pulmonary disease (COPD), asthma, chronic bronchitis, and emphysema decrease gas flow and therefore gas exchange. As a consequence, arterial partial pressure of O2 drops (hypoxemia) and arterial partial pressure of CO2 increases (hypercapnia). Interventions to improve the match between ventilation and pulmonary perfusion may be required. In the setting of acute respiratory failure secondary to acute respiratory distress syndrome (ARDS), pulmonary contusion, or severe pneumonia, hypoxia is the primary factor due to consolidation of the pulmonary parenchyma. In the setting of acute pulmonary embolism (PE), ventilation/perfusion (V/Q) mismatch is also enhanced secondary to disruption of perfusion to segments of lung tissue.
Basic features of respiratory dysfunction include dyspnea, hypoxia, and hypercarbia. There are a variety of medicine conditions leading to respiratory dysfunction, which is defined as a drop in PO2 <60 mm Hg or a rise in PCO2 >50 mm Hg. Table 47.1 is an overview of the four main mechanisms of respiratory dysfunction.
TABLE 47.1
MECHANISM OF RESPIRATORY DYSFUNCTION
Hypoxemia
The rate and severity of hypoxia and hypercarbia affect the clinical and neurologic presentation of the patient. Patients may experience mild confusion and headache to loss of consciousness. Hypoxemia should always be considered a cause of altered mental status. Prolonged central nervous system hypoxia has the potential to lead to hypoxic–ischemic encephalopathy through neuronal death. Hypoxia may be secondary to airflow obstruction in conditions such as asthma or airspace flooding as seen in pneumonia, aspiration, or hemorrhage. Acute airflow obstruction can cause hypoxemia that is typically treatable with supplemental oxygen. However, conditions that result in airspace flooding typically require adjuvant therapies and are not reversible by additional oxygen alone. Pulmonary shunting occurs when the alveoli of the lung is perfused normally; however, there is ventilation failure to the perfused regions leading a change in the ventilation/perfusion ratio. In a healthy individual, the physiologic shunt is rarely over 4%.3 Shunting is minimized by the reflexive constriction of pulmonary vasculature to hypoxia. In conditions such as pulmonary contusion, the shunt increases considerably and mechanical ventilation may be required. Therapy should always focus on treatment for the underlying cause of hypoxemia.
Ventilatory Failure
The etiology of hypocapnia and hypoxia are frequently different. With neurologic depression, the decrease in the drive to breathe reduces minute ventilation leading to increase PaCO2. In patients who require a high minute ventilation to maintain a normal PaCO2, there is often elevated CO2, increased dead space, or a high degree of ventilated but poorly perfused alveoli.4 This is a common etiology in our trauma and perioperative patients in combination with neurologic impairment.
Perioperative and Neurologic Impairment
The perioperative period is high risk for progression of respiratory dysfunction. Patients have a significant risk for atelectasis, which is the primary mechanism of perioperative respiratory dysfunction. Abnormal abdominal mechanics reduce the end-expired lung volume leading to progressive lung collapse in the dependent portions of the lung. The addition of narcotics or benzodiazepines can lead to a combination of ventilatory dysfunction leading to respiratory failure. Treatment of patients in the perioperative patients should focus on excellent bronchopulmonary hygiene to minimize atelectasis and clear secretions. This includes incentive spirometry, early ambulation, maintenance of euvolemia, and patient positioning. Attention to abdominal/thoracic pain and incisional pain is necessary and epidural anesthesia or transcutaneous electrical nerve stimulation should be considered for those individuals at high risk for pulmonary dysfunction including the obese, elderly, and patients with thoracic trauma. Lung collapse can be detected radiographically and on routine exam and should be treated aggressively with techniques for lung recruitment.
Shock
Patients with hypotension secondary to cardiogenic, hypovolemic, or septic shock often present with respiratory dysfunction. While there is no primary pulmonary mechanism, mechanical ventilation is often necessary. This allows for stabilization of gas exchange and minimizes cardiac steal until the underlying cause is identified and treated.
The mainstay of treating acute respiratory dysfunction is mechanical ventilatory support. Identification and treatment of the underlying cause is paramount. Prompt initiation of supportive ventilation during this time is key to preserving neurologic function and preventing permanent sequelae.
Special Populations
Children
The unique anatomy of children is important for both diagnosis and treatment of acute respiratory dysfunction. Children have a large head and tongue in proportion to the size of the oral cavity as well as prominent tonsils and adenoid tissue. Increased neck flexion from the prominent occipital bulge can lead to airway obstruction. Focus on proper head positioning including chin-lift and jaw-thrust is especially important in this population.5 Equipment sizing is also imperative. Broselow tape and equipment of various sizes should be immediately available.
Neonates and infants are obligate nose breathers. Nasal obstruction can cause both increased work of breathing and respiratory distress. Nasal suctioning can help to improve respiratory status in these patients.6 The larynx is well protected behind the mandibular arch and due to the relatively short neck; only a small portion of the trachea is unprotected above the manubrium. There is increased elasticity of the tracheobronchial tree. Laryngeal injuries are rare but often present with vague symptoms including hoarseness and subcutaneous emphysema, whereas tracheobronchial injuries often present with pneumothorax with or without a persistent airleak.7
Due to the increased plasticity of the chest wall in children, blunt injury to the chest is transmitted to deeper structures. Thirty-four percent of pediatric patients with pulmonary contusions do not show radiographic evidence of rib fractures.8 Injuries such as pulmonary contusion, pneumothorax, hemothorax, blunt cardiac injury, and great vessel trauma can be found without rib fractures and a high index of suspicion is required for quick diagnosis.6
Elderly
A broad range of physiologic changes compromise the anatomic and functional integrity of the respiratory system in the elderly. This includes impared elastic recoil of the lung and decreased dynamic compliance, both which increase airflow resistance leading to progressive airflow trapping. Decreased size and number of muscle fibers impairs the strength of intercostal and diaphram contraction. Individual response to hypoxemia and hypercapnia also wanes with age.9–11 Reduced plasticity of the chest wall also increases the risk of the elderly for rib fractures and the associated complications.
Obesity
Excess adipose tissue in obese individuals reduces chest wall compliance, increases airway and pulmonary resistence, and increases work of breathing.12 Obesity-hypoventilation syndrome (pickwickian syndrome) is found in one-third of individuals with a BMI of >35 (morbidly obese).13 Nearly all have obstructive sleep apnea and >50% develop pulmonary hypertension.14 Care must be taken to maintain adequate oxygen saturation (of >88%), especially in the setting of narcotics and/or benzodiazepines in trauma or postoperative patients. Continuous airway monitoring should be considered as diminished neuromuscular response to hypercapnia may quickly lead to hypoventilation, hypercapnia, acidemia, and respiratory distress.14,15 The risk and rate of deterioration will be accelerated by intoxication, rib fractures, spine immobilization, and limited mobility in all patients, but perhaps to a greater degree in the obese and elderly population.
While the management of respriatory distress of obese patients is similar to normal weight individuals, bilevel positive airway pressure (BIPAP) should be considered in stable patients. However, BIPAP should never delay or be used instead of endotracheal intubation.14
Pregnancy
One of the principal causes of morbidity and mortality among pregnant women is acute respiratory dysfunction. Studies suggest that up to 30% of maternal deaths results from acute respiratory failure. Predominent causes include adult respiratory distress syndrome, thromboembolism, venous air embolism, beta-adrenergic tocolytic therapy, and amniotic fluid embolism.16 The etiology of respiratory failure is similar in nonpregnant patients but the physiologic changes of pregnancy complicate standard management. In pregnancy, minute ventilation increases by 50% due to increased respiratory rate and tidal volume required to compensate for changes in maternal, fetal, and placental oxygen requirements and CO2 production. There is a mild respiratory alkalosis in pregnancy with a reduction in PaCO2 to 27–34 mm Hg (normal 35–45 mm Hg) and a compensatory reduction in bicarbonate to 18–21 mL/Eq/L (normal 22–30 mL/Eq/L) to maintain arterial blood pH of 7.4–7.45.17,18 Oxygen consumption increases by up to 20%–30% in the third trimester and maternal cardiac output increases by 50% during the first two trimesters due to both an increased stroke volume and heart rate and a decrease in systemic vascular resistance. Fetal oxygenation depends on maternal cardiac output. Therefore, diuresis, positive end-expiratory pressure (PEEP), and vasopressor treatments, which may change the maternal blood flow and shunt blood away from the uterus, may put the fetus at risk.19 As long as the maternal PaO2 is maintained above 60 mm Hg and both cardiac output and fetal blood flow remains stable, the fetus will not be affected adversely.17
Standard treatment for respiratory dysfunction includes ventilatory, hemodynamic, and nutritional support. However, in pregnancy, maternal–fetal physiology and drug safety require additional consideration. Respiratory dysfunction in pregnancy results from hypoxic and hypercapnic–hypoxic physiologic causes. Hypoxic respiratory dysfunction is the predominant cause of pregnancy-related respiratory failure that includes ARDS, PE, venous air embolism and amniotic fluid embolism pneumonia, cardiogenic pulmonary edema, and pneumothorax. Hypercapnic–hypoxic respiratory dysfunction results from narcotics or sedatives, asthma, myasthenia gravis, and Guillain Barre.17
ARDS is a common final pathway of pulmonary injury and has a similar presentation and mortality rate for both gravid and nongravid patients. Outcomes are related to comorbidities, age, injury, and etiology and range in the literature from 30% to 70%.16,20 Causes of ARDS unique to pregnancy include tocolytic-induced pulmonary edema, eclampsia, aspiration chorioamnionitis, amniotic fluid embolism, trophoblastic embolism, placental abruption, obstetric hemorrhage, endometritis, retained placental products, and septic abortion.17 Pregnancy brings the additional challenge of evaluating for the presence and etiology of pulmonary edema and fetal monitoring. The use of a pulmonary artery catheter may aid in diagnosis and tailoring therapeutic decisions.16 Fetal assessment is best with continuous fetal heart monitoring and sonographic biophysical profile done in consultation with obstetrics colleagues.21 Hypovolemia of pregnancy, particularly during the 30th to 32nd week gestation, may also contribute to progression and poor outcomes of ARDS in this population.16
The mainstay of therapy in respiratory failure in the pregnant patient is maternal stabilization, fetal monitoring, treatment of underlying cause, prevention of aspiration, and low-tidal lung protective mechanical ventilation with the application of PEEP when indicated. As PEEP exceeds 10 cm H2O, there is decreased venous return, decreased cardiac output, which worsens fetal oxygen delivery. The effect of hypercapnia on fetal blood flow is unknown.17 Fluid restriction in pregnancy is controversial. While it improves oxygenation, fluid restriction worsens cardiac output and organ perfusion.17
COMMON CAUSES OF ACUTE RESPIRATORY DYSFUNCTION
Acute Respiratory Distress Syndrome
ARDS is characterized by dysfunction in respiratory mechanics and gas exchange. The mortality rate ranges from 40% to 50%.22 The incidence in the United States is approximately 190,000 cases per year affecting a wide distribution of both medical and surgical patients and is associated with >74,000 deaths per year.23 In 1994, the American-European Consensus Committee recommended seperating patients with lung disease into two groups, those with acute lung injury (ALI) and those with more severe hypoxia, ARDS. Criteria include: acute onset, bilateral infiltrates on chest radiograph, pulmonary artery wedge pressure <18 mm Hg or the absence of left atrial hypertension. ALI is considered to be present if the PaO2:FiO2 ratio is <300, whereas ARDS requires a PaO2:FiO2 ratio to be <200.24
Several studies have sought to improve outcome following ARDS. The only study that has demonstrated a major improvement in outcome utilized a lung-protective ventilation strategy with lower than usual tidal volumes.20 The pathogenesis of ALI and ARDS in surgical patients is variable. These most commonly include sepsis, trauma, aspiration, near drowning, blood transfusion, pulmonary contusion, and inhalation injury.25 Typically, an initial inflammatory stage with neutrophil infiltration and massive capillary leak is then followed by a fibroproliferative phase, drastically changing the elasticity of the lung.24,25
The clinical course of ARDS is variable with patients recovering within weeks to months of prolonged ventilation. Rather than respiratory failure itself, it is prolonged ventilation with superimposed infection and multisystem organ failure that ultimately leads to death. Treatment strategies include ventilatory support to minimize iatrogenic injury with a goal to avoid progression of pulmonary damage and modulation of inflammatory response through pharmocologic options.25 Studies of treatment options have only shown a mortality benefit for patients with ARDS with low-tidal ventilation.26 The Acute Respiratory Distress Syndrome network (ARDSnet) trial randomized patients to receive ventilatory support with 6 mL/kg versus 12 mL/kg based on predicted body weight. Allowing for hypercapnia, there is a goal plateau pressure of <30 cm H2O. The low tidal volume group (6 mL/kg) had a significantly lower mortality rate (31% vs. 40%), and increased number of ventilator-free days.20
Pneumothoraces
A breach in the parietal or visceral pleural can result in air in the pleural space (pneumothorax). Diagnosis of small pneumothoraces is most commonly made by chest radiograph; however, the presence of a tension pneumothorax should be evident on physical exam. A small separation of space may translate into a large pneumothorax, particularly depending on the position of the patient during chest radiograph. A tension pneumothorax is created when a large amount of intrapleural air causes a shift in the mediastinum toward the contralateral lung. Stab or gunshot wounds may create a sucking chest wound. Ventilation and venous return of blood to the heart may be compromised resulting in hypotension that can lead to cardiovascular collapse. Open and tension pneumothoraces should be diagnosed clinically based on physical exam including decreased breath sounds and hypotension and treated as a surgical emergency.
Pneumothoraces are classically classified as spontaneous or acquired. Typically, spontaneous pneumothoraces are secondary to rupture of small blebs due to increased transpulmonary pressure. In addition to connective tissue disorders, common causes of spontaneous pneumothorax are rupture of subpleural blebs after coughing, blunt chest trauma, rapid fall in atmospheric pressure, rapid decompression (seen in scuba diving), and high altitude with flying. Presentation ranges from asymptomatic to pleuritic chest pain, decreased breath sounds, and dyspnea, and may progress to hypotension, tachycardia, diaphoresis, and cyanosis, and deviation of trachea if a pneumothorax progresses to a tension pneumothorax.27
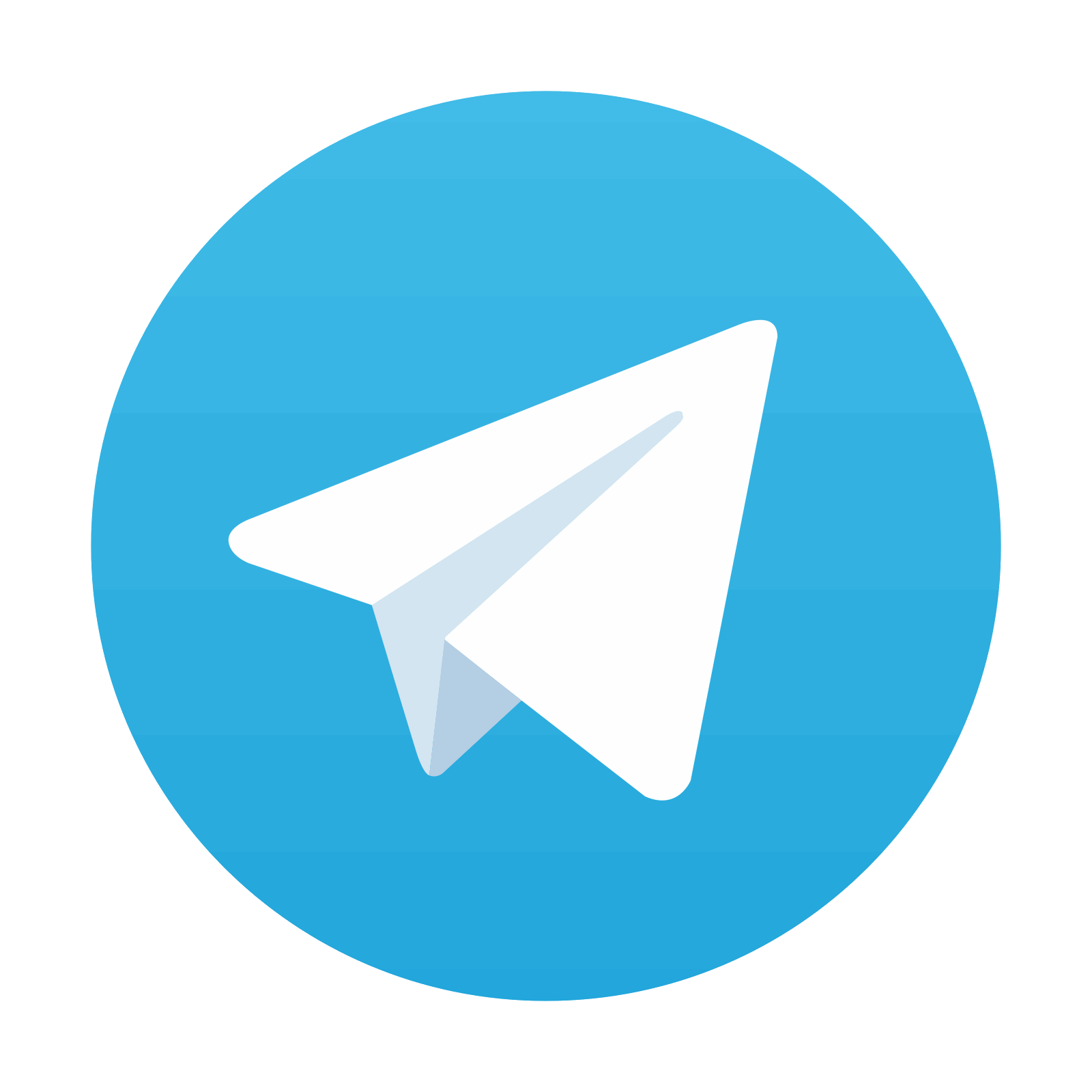
Stay updated, free articles. Join our Telegram channel

Full access? Get Clinical Tree
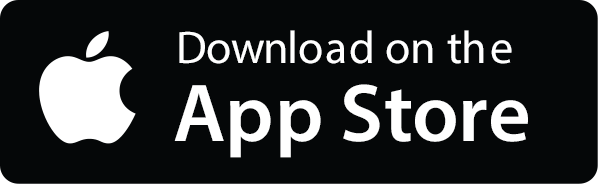
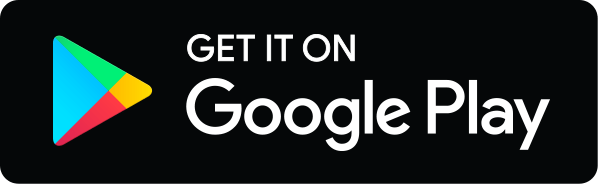