NOSOCOMIAL INFECTIONS
In order to help the clinician make decisions about empiric antibiotic selection, the classification of infections has typically been divided into the site of infection (i.e., lung, skin and soft tissue, urinary tract, and intraabdominal) and the location of the patient at the time the infection occurs.1–3 Historically, location has been divided into community and hospital-onset, with hospital-acquired infections (HAIs) occurring after 72 hours of hospitalization, but patient demographics, risk factors, and pathogens have changed substantially over the last two decades, making this classification schema no longer informative. Bacterial resistance to antibiotics after exposure to the health care setting, and the potential for infection due to cross-contamination from the environment or from transmission from other patients, must now be considered in the initial choice of antibiotics. Because of these changes in epidemiology, infections are now classified as health care-associated, hospital-acquired, or community-acquired.1
HAIs remain a leading cause of morbidity and mortality, occurring in as many as 10% of hospitalized patients. Hospitals vary substantially in rates and types of HAIs, which may relate to coding, referrals, patient demographics, use of devices and procedures, as well as actual quality of patient care.1,4 From January 2006 to October 2007, 28,502 HAIs were reported to the National Hospital Surveillance Network (NHSN): 10,064 (35.3%) were cases of central line-associated blood stream infection (CLABSI), 8,579 (30.1%) were cases of catheter-associated urinary tract infection (CAUTI), 4,524 (15.9%) were cases of ventilator-associated pneumonia (VAP), 5,291 (18.6%) were cases of surgical site infection (SSI), and 44 (0.2%) were cases of postprocedure pneumonia.1
Patients in intensive care units (ICUs) suffer a disproportionally high percentage of HAI when compared with patients in non–critical care areas.1,5–9 For example, ICU beds account for 5%–10% of all hospital beds, yet 45% of CLABSIs occur in ICUs. Thus, the clinician must consider not only the individual patient risk factors but also the location of the patient in determining the probability and source of infection.5
This chapter discusses some of the common HAIs encountered in surgical patients, including pneumonia, CLABSIs, and UTIs, and to a lesser extent intraabdominal infections (IAIs), emphasizing epidemiology, pathogenesis, prevention, and treatment. SSIs are discussed in detail elsewhere (see Chapter__).
PNEUMONIA
Pneumonia is one of the most common nosocomial infections of hospitalized patients.1,6 Health care-associated pneumonia (HCAP) occurs among patients hospitalized for 90 days; who live in nursing facilities; who have received antibiotics, chemotherapy, or wound care recently; or who undergo hemodialysis.3 These patients are at risk for the same pathogens as patients who have hospital-acquired pneumonia (HAP), which by definition occurs after 48 hours of inpatient care in previously healthy patients. Distinguishing HAP from community-acquired pneumonia (CAP) is important, as patients with HAP are susceptible to pneumonia from different, potentially more virulent pathogens. VAP is a subset of HAP that occurs among patients after more than 48–72 hours of mechanical ventilation. Each day the patient remains on mechanical ventilation, the risk for development of VAP increases.
Because the cost (increased length of stay) of VAP and HAP is substantial, prevention has become an important focus for quality improvement and pay-for-performance reporting activities.10,11 Clear recognition of the risk factors and understanding of the probable factors in disease development can lead to rational preventive strategies. Moreover, knowledge of varying diagnostic and treatment regimens may lead to improvements in patient care and outcomes.
Epidemiology
HAP is one of the most common nosocomial infections. At a rate of 3–10 cases per 1,000 hospital admissions, HAP may increase a patient’s hospital stay by more than 1 week, resulting in up to $40,000 in additional cost.1,9–12 A large and important subset of HAP, the risk of VAP is estimated at 3% per day for the first 5 days of mechanical ventilation, 2% per day for days 6–10, and 1% per day thereafter, with each day of mechanical ventilation adding incremental risk.12
Pathophysiology
The pathogenesis of HAP is multifactorial; several patient and treatment risk factors have been identified (Table 54.1). Most cases of HAP are secondary to microaspiration of infected material into the normally sterile lung parenchyma.14 Thus, patient risk factors, the amount of pathogens delivered into the respiratory tree, and the virulence of infecting pathogens influence the probability of developing HAP and potentially the severity of the disease.
Table 54.1
RISK FACTORS FOR THE DEVELOPMENT OF VENTILATOR-ASSOCIATED PNEUMONIA
Classifying pneumonia into CAP and HAP recognizes that the causative pathogens differ and even change during hospitalization.12 Because expected pathogens differ and successful outcome depends on appropriate initial treatment, general recommendations for empiric treatment depend on whether the patient has CAP or HAP (or HCAP), and the duration of the current hospitalization.1,14–16 Distinguishing HCAP and CAP, broad-spectrum initial treatment of HCAP followed by de-escalation, has not led to measurable changes in mortality in prospective studies. Early HAP (<5 days into the hospital course) is associated with a better prognosis than late-onset HAP (arising ≥5 days into a hospital course).1 These two categories can then be subdivided further into patients with and without prior antibiotic exposure.
Early-onset HAP in patients with no prior antibiotic exposure tends to mirror CAP, and patients generally have susceptible pathogens. The most common pathogens include Enterobacteriaceae, Haemophilus influenzae , Streptococcus pneumoniae , and methicillin-sensitive Staphylococcus aureus (MSSA).14,15 Patients with no prior antibiotic exposure who develop late-onset HAP are infected by similar bacteria; however, occasionally these patients present with gram-negative bacilli (GNB) resistant to first-generation cephalosporins. Late-onset HAP in patients with prior antibiotic exposure presents a greater problem in both the prediction and empiric treatment of likely pathogens. As many as 40% of such patients present with potentially multi–drug-resistant (MDR) pathogens, including Pseudomonas aeruginosa, Acinetobacter baumannii , and methicillin-resistant Staphylococcus aureus (MRSA).15
Gram-positive Bacteria. The common gram-positive cocci causing pneumonia in hospitalized patients are S. pneumoniae and S. aureus.14–16 Patients with traumatic brain injury and early HAP may be infected with S. aureus. Whereas these pathogens are often seen in early-onset pneumonia, S. aureus can cause pneumonia at any point of the hospitalization, and MRSA is typically observed later in the hospital course. Risk factors for MRSA pneumonia include chronic obstructive pulmonary disease (COPD), longer duration of mechanical ventilation, prior antibiotic exposure, prior use of corticosteroids, prior bronchoscopy, colonization with MRSA, and adjacency to a patient identified to harbor MRSA.16
Gram-negative Bacteria. Gram-negative bacteria associated with early-onset HAP include H. influenzae and lactose-fermenting GNB such as Enterobacteriaceae, including Escherichia coli , Klebsiella species, and Enterobacter species.14–16 Overgrowth of these organisms can be associated with prior antibiotic therapy, and their virulence may increase in critical illness. Enterobacteriaceae are increasingly demonstrating extended-spectrum beta-lactamase (ESBL) activity.17,18 Whereas these organisms were treated frequently with broad-spectrum beta-lactam antimicrobials, plasmid-mediated resistance to these agents is increasing, and major outbreaks of VAP secondary to ESBL-producing pathogens have been identified.17,18 Stains that produce ESBL are resistant to all beta-lactam agents and exhibit high-level resistance to fluoroquinolones, making carbapenems the recommended first-line agents for ESBL-producing strains.17,18
P. aeruginosa is the most common MDR GNB causing HAP/VAP,1,16 and the most frequent VAP isolate in patients on mechanical ventilation for more than 4 days.19 Resistance to one or more antibiotics is common and patients with MDR P. aeruginosa are at increased risk of severe sepsis and death.20 A. baumannii , an aerobic, non–lactose-fermenting GNB that has intrinsic resistance to many antibiotics, can cause nosocomial infections that may spread rapidly among hospitalized patients16,21–23 Due to its ability to acquire resistance rapidly to many drugs, prior antibiotic exposure is a major risk factor for resistance.24
Prevention
Patients scheduled for elective surgery may be identified to be at increased risk for postoperative pneumonia, and may be candidates for risk modification.25,26 The respiratory risk index is a scoring system that categorizes patients as low-, medium-, or high risk for postoperative respiratory failure, based on factors such as emergency and complex operations, as per American Society of Anesthesiologists (ASA) score (see Chapter 10), and patient comorbidities (e.g., COPD, ascites, chronic kidney disease).26 Potentially modifiable risk factors are indicated in Table 54.2.27–73 The use of “ventilator bundles,” such as those promulgated by the Institute for Healthcare Improvement (IHI), and similar quality improvement efforts have decreased the incidence of VAP by more than 50%.27,28 However, the IHI paradigm contains two measures not likely to decrease the incidence of VAP (prophylaxis of stress-related gastric mucosal hemorrhage and venous thromboembolic disease, whereas other measures such as the effect of topical chlorhexidine used for oral hygiene, and recommendations about specific endotracheal tubes (e.g., silver-coated tubes) are not included in current bundles.
TABLE 54.2
Risk Factor Reduction and Prevention for Ventilator-Associated Pneumonia
Diagnosis
Clinical Evaluation. The method of establishing the diagnosis of HAP remains controversial, undoubtedly due to difficulty establishing the differences among colonization, tracheobronchitis, and HAP. Neither radiological nor microbiological features in isolation can establish the diagnosis of HAP. The U.S. Centers for Disease Control and Prevention (CDC) and the NHSN have developed criteria for the diagnosis of nosocomial pneumonia, taking into account clinical factors, such as fever and leukocytosis, as well as radiological criteria, including persistent new findings on chest radiography2,74 (Table 54.3). The controversy about whether to use clinical, noninvasive, or invasive measures to establish a diagnosis continues today.75–91 An invasive diagnostic strategy does not alter mortality, but if antibiotic use is protocolized, the total duration of antibiotics may be lowered.77,87,89,90
TABLE 54.3
U.S. CENTERS FOR DISEASE CONTROL AND PREVENTION. SURVEILLANCE DEFINITION OF PNEUMONIA FOR A NON–IMMUNO-COMPROMISED ADULT PATIENTa
WBC; white blood cell count.
From http://www.cdc.gov/nhsn/PDFs/pscManual/17pscNosInfDef_current.pdf. Accessed December 29, 2010.
The Clinical Pulmonary Infection Score (CPIS)78 includes both clinical and radiological factors that indicate an increased likelihood of the presence of pneumonia. Point values are assigned to each criterion and the total is summed. Traditionally, a threshold score of >6 points has been considered diagnostic of pneumonia79 (Table 54.4). The clinical utility of the CPIS has been evaluated extensively, with both proponents and detractors.78–81 In the original dataset, when the CPIS was ≤6 points, no patient satisfied microbiologic criteria for pneumonia.79 Other investigators suggest that the CPIS, while sensitive, lacks specificity and leads to unnecessary antimicrobial treatment. Among 201 patients who underwent an invasive diagnosis of pneumonia, there was no difference in the CPIS score of patients with and without VAP. Importantly, therapy based on resolution of the CPIS would have led to 840 days of empiric antibiotics as compared to 424 days based on invasive specimen collection.79
TABLE 54.4
MODIFIED CLINICAL PULMONARY INFECTION SCORE
ARDS, acute respiratory distress syndrome; CHF, congestive heart failure; WBC, white blood cell count.
Adapted from Swoboda et al. Can the clinical pulmonary infection score impact ICU antibiotic days? Surg Infect. 2006;7:331–339.
Whereas most studies indicate that clinical evaluation is sensitive in identifying VAP, the specificity is low. Clinical diagnosis combined with short-course antibiotic therapy may be reasonable especially if a low [≤6 points] CPIS score is achieved after day three of treatment.81 In a 2000 study, Singh et al.81 examined short-course empiric therapy for patients in the ICU with suspected VAP. The rate of antimicrobial resistance or superinfection was significantly higher in patients receiving standard therapy. The duration of therapy was significantly lower in the experimental group, with no difference in mortality.
Bacteriologic Evaluation. The diagnosis of pneumonia can be established by a number of methods, including noninvasive and invasive means for sputum specimen collection and qualitative or quantitative microbiology.82–91 Blind tracheobronchial aspiration (TBAS) is a noninvasive technique accomplished by inserting a flexible suction catheter into the distal trachea via the endotracheal tube to obtain sputum for quantitative culture.86 The typical threshold for diagnosis of pneumonia is growth of >105 cfu/mL. This technique is simple but does not allow specific lung segments to be sampled, and the catheter is contaminated easily.
Bronchoalveolar lavage (BAL) allows sampling of specific lung segments suspected to be involved with pneumonia, and supports quantitative microbiology. Bacterial growth of >104 cfu/mL is typically accepted as being most accurate for diagnosis of VAP, although some authors1,89 argue that the use of 105 cfu/mL provides fewer false-positives and a lower likelihood of inappropriate antibiotic therapy.90
Specimen collection with a protected specimen brush (PSB) involves a telescoping catheter brush that is advanced blindly or through a bronchoscope, into the suspected distal airway. A diagnostic cutoff of >103 cfu/mL is typically accepted as being consistent with HAP.1,91 Complications such as bleeding or pneumothorax may be more frequent with PSB.
Proponents of invasive diagnostic means for pneumonia suggest that fewer and more selective antibiotics be used when BAL or PSB is used to collect quantitative cultures.89,90 Direct examination of BAL fluid for intracellular organisms (>5 cells) has overall sensitivity and specificity of 93.6% and 91.5%, respectively, for the diagnosis of VAP, with only 12% of patients receiving incorrect empiric therapy.85 With early appropriate antibiotic therapy being an important predictor of mortality, a technique that facilitates early guidance of therapy may be useful clinically. In a randomized trial comparing PSB versus TBAS and thus quantitative versus qualitative techniques, Ruiz et al.86 were unable to demonstrate any differences in ICU length of stay, length of mechanical ventilation, 30-day mortality, or attributable mortality between groups. However, the cost difference between groups was $368 versus $29.86 Similarly, the Canadian Critical Care Trials Group randomized 740 patients with a clinical suspicion of pneumonia to BAL on mechanical ventilation for >4 days to quantitative culture versus TBAS, with qualitative culture controlling for the timing of empiric antibiotics.87 The authors reported no difference in 28-day mortality. Additionally, there were no differences in the secondary outcomes of hospital and ICU length of stay, duration of mechanical ventilation, and ICU and hospital mortality.
On the other hand, Fagon et al.88 randomized 413 patients to invasive versus noninvasive diagnosis of VAP, and found decreased 14-day mortality, improved organ function scores, and fewer days of antibiotic therapy among patients undergoing the invasive diagnosis. Interestingly, they also identified alternative infectious diseases that required intervention when pneumonia was not verified. The greatest support for the use of invasive techniques may be reflected by reducing antibiotic utilization or by more accurate use.89,91 Shorr et al.91 evaluated four randomized trials of diagnostic techniques, and found that invasive strategies do not affect the mortality of VAP, likely because empiric antibiotic choices must be made before results of quantitative cultures become available.
Treatment
Empiric Therapy and De-escalation of Treatment. The most important factor influencing the mortality of HAP is prompt and adequate empiric antibiotic therapy.1,93,94 Treatment should be instituted immediately after specimen collection and should be directed against likely pathogens. The choice of antimicrobial agent(s) should account for risk factors such as current length of hospital stay, prior exposure to antibiotics, duration of mechanical ventilation, previous culture results, known antibiotic resistance locally among patient, contacts, and unit-specific microbiota, and host immunosuppression.94,95 Several guidelines have been published suggesting agents that could be utilized for empiric antibiotics for CAP, HAP, VAP, and HCAP.1,26,92,96,97–102 If the patient is considered low-risk by all criteria, single-agent therapy is a reasonable choice (Table 54.5).1,26 On the other hand, patients who have risk factors as noted above should be considered for double or triple-drug initial empiric therapy for VAP (Table 54.6).1,26,99,100
TABLE 54.5
EMPIRIC ANTIBIOTIC CHOICES FOR LOW-RISK PATIENTS WITH VENTILATOR-ASSOCIATED PNEUMONIA
TABLE 54.6
EMPIRIC ANTIBIOTIC SELECTION IN HIGH-RISK PATIENTS WITH SUSPECTED VAP
MRSA, methicillin-resistant S. aureus.
Whereas vancomycin is the agent used most commonly to treat MRSA in hospitalized patients, considerable debate has emerged about whether vancomycin should be used empirically as agent for suspected MRSA infection. The minimum inhibitory concentration (MIC) for vancomycin has been increasing among clinical isolates, and in a recent study, 73% of MRSA isolates had a MIC > 1.5 ĉg/mL.103 Use of vancomycin as the primary agent for empiric use to treat high-risk patients with suspected VAP is also problematic because vancomycin has poor penetration of lung tissue.104,105 Based on these trends, it is unclear whether vancomycin should remain the drug of choice for treating MRSA pneumonia, or whether linezolid should be considered as a first-line agent.106–108 When compared to pneumonia caused by MSSA, MRSA is associated with prolonged ICU length of stay and increased hospital cost despite appropriate initial therapy.108
The decision to treat high-risk adult patients with suspected HAP/VAP with three agents (one against MRSA; two against MDR GNB) is based on the concern that MDR pathogens may be etiologic and that longer time to initial appropriate antimicrobial therapy has been linked to mortality. However, extended, overly broad antimicrobial therapy will lead to the development of additional resistance.109,110 Some pathogens may be particularly difficult to treat; for example, as many as 50% of Acinetobacter isolates may be resistant to all antimicrobial agents except the polymyxins.111,112 There is some evidence that treatment with intravenous or inhaled polymyxin E (colistin) may be safe and effective for patients with MDR Acinetobacter pneumonia.111,113
In many institutions, P. aeruginosa is the most common isolated pathogen responsible for VAP or HAP. In addition, the percentage of isolates sensitive to single-agent antipseudomonal therapy can be <50%.114 This fact, along with the in vitro synergy observed with two-agent therapy (traditionally a beta-lactam and an aminoglycoside) has led to the suggestion that using two agents to cover possible MDR Pseudomonas is prudent,108 albeit with considerable debate on the point.115–121 Among trauma patients, Croce et al.118 found that patients treated with a combination of a third-generation cephalosporin and gentamicin actually had increased rates of treatment failure and superinfection compared to those treated with the cephalosporin alone. However, recent investigations suggest that patients treated with initial combination therapy are more likely to receive appropriate empiric therapy, with associated improved mortality.116,119–121 Heyland et al.120 randomized patients with suspected VAP to empiric monotherapy versus combination therapy, and found no reduction of mortality from combination therapy. However, the percentage of patients treated effectively by combination therapy was significantly higher. Moreover, patients with one or more MDR organisms were treated adequately 84% of the time by combination therapy, versus only 19% of the time by empiric monotherapy. For these reasons, current treatment guidelines recommend initial empiric double coverage for MDR GNB for critically ill patients with suspected HAP/VAP.1,99,100,121–123
De-escalation of therapy should be considered within 72 hours of initiating empiric therapy and is accomplished by: (1) changing to antibiotics with a narrower spectrum; (2) eliminating unnecessary antibiotics from the treatment regimen; or (3) changing to oral therapy.124,125 Importantly, de-escalation has not been associated with decreased response rates, higher mortality, or longer hospital or ICU lengths of stay.124,125
Duration of Therapy. Recommendations for duration of antimicrobial therapy for nosocomial pneumonia have evolved in recent years, with an overall trend to decrease duration of therapy. In the seminal paper by Chastre et al.,126 patients with VAP were randomized to 8 versus 15 days of antimicrobial therapy. All-cause mortality was similar between groups (18.8% for 8 days vs. 17.2% for 15 days, risk difference 1.6, 95% confidence interval [CI], -3.7 to 6.9). Additionally, there was no difference in recurrence rate (28.9% vs. 26.0%, risk difference 2.9 [CI -3.2 to 0.1]) of VAP. In a retrospective evaluation of the same population, pneumonia secondary to non–lactose-fermenting GNB and MRSA were both independently associated with recurrence.127 Whereas concern for recurrence exists when treating MDR infections, these results126,127 indicate that many patients may be treated safely and effectively with shortened courses of antibiotics.
The question remains: What is the shortest course of antimicrobial therapy appropriate for nosocomial pneumonia?131 Serial BAL has been used as a method of assessing response to therapy and allowing for a shorter duration of therapy.82 Discontinuation of appropriate therapy after 4 days in patients with decreased bacterial growth on repeat BAL decreases antibiotic duration and total antibiotic days, with no effect on mortality, length of stay, ventilator-free days, relapse rate, or rate of superinfection,82 indicating that shortening of antibiotic courses may be accomplished safely in appropriate patients.
Outcomes
HAP has a substantial impact, both medically and economically, with incremental cost attributed to a single episode of HAP of up to $50,000.2,5,10 Specifically, ICU patients with VAP experience longer ICU and hospital lengths of stay, which is a major driver of cost.2,5,10 Prevention of HAP is therefore the focus of quality improvement efforts, and is, in part, why use of ventilator “bundles” for both prevention and therapy has blossomed.106,128–130 However, the effect of VAP on mortality is unclear. In trauma and in patients with acute respiratory distress syndrome (ARDS), VAP does not appear to increase mortality, thus mortality is not a valid endpoint in studies of prevention of VAP.132
CATHETER-RELATED (-ASSOCIATED) BLOOD STREAM INFECTION
Definitions, Epidemiology, and Risk Factors
Central venous catheters are used commonly in patients for hemodynamic monitoring and administration of fluids and drugs, total parenteral nutrition, and blood products, and renal replacement therapy. The main complication of catheter use is infection, which can occur at the site of insertion, within the blood stream, or result in metastatic infection.133 Central line-associated BSI is defined from a surveillance perspective if a catheter is present within 48 hours of the culture and bacteremia occurs, irrespective of whether the catheter itself has been identified as a causative vector.134 A laboratory-confirmed BSI (LCBSI) is defined when a patient has one of two possible conditions: (1) a recognized pathogen cultured from one or more blood cultures that is not related to infection at another site; and (2) signs and symptoms of infection and positive laboratory results not related to infection at another site; common skin contaminants must be cultured in blood from two or more sites, cultured on separate occasions.134 These surveillance definitions overestimate the true incidence of infection because they do not account for a bacteremia from an undocumented source, such as an IAI. On the other hand, a catheter-related BSI (CRBSI) is confirmed when the catheter (cultured by the semi-quantitative “roll-plate” technique; >15 cfu—see below) and blood have identical pathogens. In the United States it is estimated that 250,000 BSIs occur annually, with rates ranging between 1.3 and 5.5 infections per 1,000 catheter-days.135
Catheters can become colonized or infected by two primary routes: (1) endogenous contamination from the skin alongside the catheter, causing catheter colonization and blood stream infection; or (2) endoluminal contamination from biofilm, a contaminated hub, or injection of infected fluid.136 Catheter design and logistics must be understood to assess the associated risk.136 For example, the catheter can be designated by the type of vessel it occupies (e.g., peripheral venous, central venous, or arterial); its intended life span (e.g., temporary or short-term vs. permanent or long-term); the insertion site (e.g., subclavian, femoral, internal jugular, or peripheral vein, peripherally inserted central catheter [PICC]); the pathway from skin to vessel (e.g., tunneled vs. nontunneled); its length; or some special characteristic of the catheter (e.g., cuffed or uncuffed, impregnation with heparin, antibiotics, or antiseptics, and the number of lumens).
Traditionally, the diagnosis of a CRBSI is established when both the catheter and blood stream yield the same pathogen.136 A catheter may be cultured using the semi-quantitative “roll-plate” technique, whereby a 5-cm segment of catheter is rolled onto an agar plate; growth of >15 cfu is considered meaningful.137 Pooled data suggest a sensitivity and specificity of 85% and 82%, respectively.138 A more labor-intensive method intended to account for endoluminal pathogens subjects the catheter segment to sonication before plating. Growth of >103 cfu/mL is then considered diagnostic, with sensitivity and specificity of 83% and 87%, respectively.138 However, both methods require catheter removal, thus alternatives have been considered. Simultaneous quantitative blood cultures can be drawn peripherally and through the catheter. A diagnosis of catheter-related infection is considered if the growth from the catheter is fivefold greater than the periphery. This method has sensitivity and specificity of 93% and 97% for long-term catheters.138 Furthermore, if blood drawn from catheter turns positive more than 2 hours before blood drawn simultaneously from a peripheral site, CLASBI is confirmed with sensitivity and specificity of 90% and 72%.138
Data from the NHSN indicate that the most common pathogens isolated from CLABSIs were coagulase-negative staphylococci (34.1%), Enterococcus spp. (15.0%), Candida spp. (11.8%) and S. aureus (9.9%). Depending on local patterns, the frequency of resistant pathogens can vary widely, with more than one-half of S. aureus isolates being MRSA, 79% of E. faecium isolates being vancomycin-resistant (VRE), and up to 30% of Pseudomonas spp. resistant to more than one antimicrobial agent. More recently, K. pneumoniae and Enterobacter spp. may be resistant to cephalosporins via production of an ESBL.139 Moreover, A. baumannii and Klebsiella spp. may be carbapenem-resistant, which can be transmitted rapidly to other GNB.
Prevention
Substantial progress has been made in preventing BSIs by implementing care bundles, including simple measures such as education, improved hand hygiene, use of full barrier precautions for catheter insertion, chlorhexidine skin antisepsis, avoidance of femoral sites, and prompt removal of unnecessary catheters.28,140–144 After these measures proved to reduce infection substantially at Johns Hopkins, deployment in State of Michigan ICUs decreased rates of CLABSI and CRBSI from 7.7 to 1.4/1,000 catheter-days at 16–18 months.144
In addition to processes of care and bundles designed to reduce infection, technological advances such as catheter coatings with antiseptics or antimicrobials reduce infections when the catheter is in place for an average of 5–12 days [0.40, 95% CI, 0.27–0.58], but not 13–20 days [0.69, 0.42–1.14].145 Ethanol lock solutions have been investigated for both prevention and treatment because resistance is unlikely and cost is modest. However, the concentration of ethanol, the dwell time, and the effect on the catheter all require elucidation.146,147
Antimicrobial locks have also been used in both clinical trials and in selected clinical conditions, such as in patients with tunneled hemodialysis catheters,148–151 where CLABSI and hospitalization were reduced by 52% and 69%, respectively, albeit with concern for possible increased resistance to gentamicin in coagulase-negative Staphylococcus isolates.148 Antimicrobial lock solutions are not recommended currently for routine use.136
The role of catheter dressings in reducing infections lacks clear recommendations as to the preferability of gauze or transparent dressings despite more than 20 years of study.152–156 More recently, chlorhexidine gluconate-impregnated sponges have been shown consistently to reduce catheter and skin colonization, albeit with an increase in local skin reactions, especially notably in low-birth-weight infants (<1,000 g).157–163
URINARY TRACT INFECTION
Definitions, Epidemiology, and Risk Factors
Urinary tract infection (UTI) is the single most common HAI, representing more than 30% of all HAIs reported by acute-care hospitals.1,2 The gold standard for diagnosis is the detection of a pathogen in the urine of a symptomatic patient. Traditionally, the microbiological quantification of a UTI has been the isolation of >105 cfu/mL from a mid-stream urine collection. However, this definition was proposed from healthy female outpatients, not from catheterized inpatients.
Several guidelines may assist clinicians in the prevention, identification, and management of UTIs.164–169 However, sufficient evidence is lacking to support a clear definition of CAUTI versus the far more common (and often inappropriately treated) asymptomatic catheter-associated bacteriuria. In the recent guidelines of the Infectious Diseases Society of America (IDSA),170 the term catheter-associated asymptomatic bacteriuria (CA-ASB) is retained, but it is omitted from the NSHN definitions (Table 54.7), and is considered by NHSN as a disease only when bacteremia ensues.164 According to the IDSA guidelines170:
TABLE 54.7
NHSN DEFINITION OF CAUTI
aFever is not diagnostic for UTI in the elderly (>65 y of age) and therefore fever in this age group does not disqualify from meeting the criteria of an ABUTI.
bUropathogen microorganisms are: GNB, Staphylococcus spp., yeasts, beta-hemolytic Streptococcus spp., Enterococcus spp., G. vaginalis , Aerococcus urinae , and Corynebacterium spp. (urease positive).
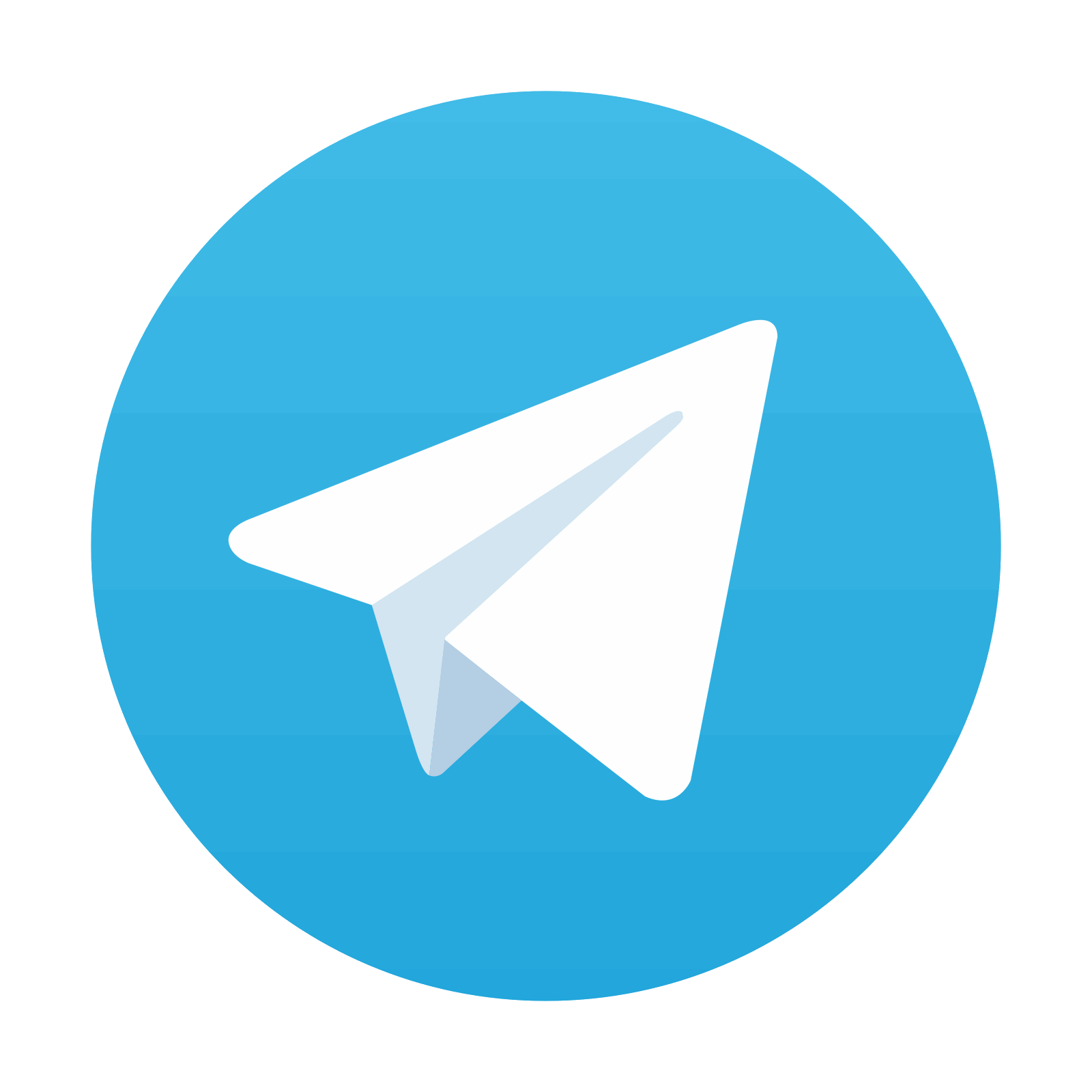
Stay updated, free articles. Join our Telegram channel

Full access? Get Clinical Tree
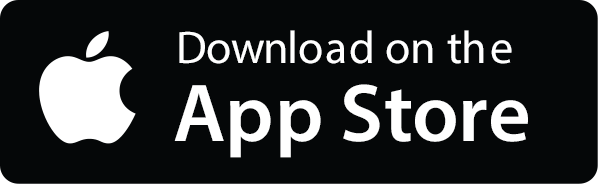
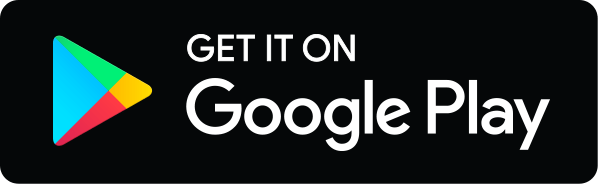