© Springer Science+Business Media New York 2015
Alan David Kaye, Adam M. Kaye and Richard D. Urman (eds.)Essentials of Pharmacology for Anesthesia, Pain Medicine, and Critical Care10.1007/978-1-4614-8948-1_3939. The Intensive Care Unit
(1)
Department of Anesthesia, Harvard Medical School, Boston, MA 02215, USA
(2)
Department of Anesthesia, Critical Care and Pain Medicine, Beth Israel Deaconess Medical Center, Boston, MA, USA
Introduction
Caring for patients in the intensive care unit demands constant, meticulous thought and consideration while implementing every aspect of their management. Among the many life-sustaining therapies provided for those in the intensive care unit (ICU), the use of an individually tailored pharmacologic regimen has the potential to dramatically improve the overall health of the patient as well as to do great harm. Severe derangements in the physiologic responses and homeostatic mechanisms of critically ill patients often times result in challenges in constructing a durable therapeutic regimen. At times, these alterations in function can be difficult to detect, and their effects on the intended action of pharmacologic substances are often impossible to quantify. Knowledge of these specific disease processes, their potential effects on pharmacodynamics and pharmacokinetics, as well as an intimate familiarity with the pharmacologic agents most often used in the intensive care unit can lead to an optimized approach to improving the health of the critically ill patient.
Therapeutic Index and Intended Effect
As is always the case when providing any modality of therapy, one must decide whether the benefit of the intervention outweighs the risk that it may impose upon the patient. In the care of the critically ill, at times, the boundary between an acceptable risk and an unacceptable one can be obscured by the desire to provide life-saving or sustaining measures and the potential to create serious iatrogenic harm. Ideal pharmacologic regimens for these patients would then include drugs with a high therapeutic index (TI), defined as the ratio of therapeutic plasma levels to toxic plasma levels. In general, drugs with a favorable TI would exhibit an intended effect at a range of plasma levels far separated from a range in which the drug could cause potential harm. Often times, the critical nature of the patient’s disease dictates the use of an agent with a more unfavorable risk profile, because of either a lack of alternatives or an unsustainable progression of disease without treatment. In these challenging cases, agents with lower therapeutic indices should be selected when their intended effect can either be readily observed with recognizable changes in the patient’s vital signs and/or physical exam characteristics or directly measured with plasma concentrations.
Mode of Administration
Frequently, an agent’s intended effect and therefore clinical benefit can be augmented by selecting a different mode of administration. For example, an agent may have a low therapeutic index or limited time periods of effective plasma concentrations when given via intermittent bolus. The same agent may display more stable and therefore easier to predict serum concentrations if given via continuous intravenous infusion. Ideal candidates for continuous infusion would be agents that have a short context-sensitive half-life and do not accumulate in tissues over time, which could lead to a more prolonged effect than is intended. Examples of medications in this category might include propofol, furosemide, and nitroglycerin. In certain cases, the intended plasma concentration may not be obtained rapidly via continuous infusion alone, and a loading dose may be necessary. Once the desired effect is observed, however, patients can be maintained on an appropriate dose without being subjected to the potentially harmful effects of labile plasma concentrations of agents with a low therapeutic index.
Bioavailability and Route of Administration
Bioavailability refers to the fraction of active drug that reaches the systemic circulation. Drugs given via an intravenous route are thought to be 100 % bioavailable. In general, drugs given via the enteral route are subjected to absorption via the intestinal tract into the portal venous system, where it then undergoes varying amounts of metabolism in the liver before it reaches the systemic circulation. This phenomenon is referred to as the first-pass effect. This effect may lead to decreased bioavailability of enterally administered agents, delays in achieving intended effects, as well as the production of possible harmful metabolites. In critically ill patients, the enteral route of administration may be inappropriate for these reasons as well as many others. Postsurgical or trauma patients may have severe alterations in either the continuity or function of their gastrointestinal tract. Many mechanically ventilated patients will be maintained on opioid infusions leading to ileus, prolonged intestinal transit time, and delayed entry of enterally absorbed medications into the portal system.
Many therapeutic agents can be given via alternative dosing routes. Due to predictable and sustained absorption when given via subcutaneous injection, this route has become the delivery method of choice for anticoagulants including both unfractionated and low molecular weight heparin. These medications are widely given to ICU patients to prevent venous thromboembolism, a common and often disastrous development in this patient group. Other delivery routes may be chosen to target a specific site of action. Inhaled nitric oxide and tobramycin are both incredibly selective for lung capillaries and distal alveoli, respectively, when given via the inhaled route. The same can be said for nebulized agents such as the beta-agonist albuterol and the anticholinergic ipratropium bromide. Finally, the sublingual or buccal route takes advantage of the rich capillary network seen within the oral cavity and has an additional advantage of its venous drainage bypassing the portal circulation via the superior vena cava. Substances available for this route of administration will then exhibit rapid uptake and near 100 % bioavailability. Examples include sublingual nitroglycerin, the fentanyl lollipop, and sublingual olanzapine.
Volume of Distribution
An agent’s volume of distribution (Vd) can be thought of as the attempt to quantify the volume in the various compartments of the body that the agent can be found. This volume not only represents the circulating blood volume and the intended site of action but is also affected by many factors including protein binding, tissue binding, and lipid solubility of the drug in question. For these reasons, the Vd of most pharmacologic agents cannot be simply estimated by quantifying the extracellular fluid volume or lean body weight of the individual, and the resultant calculated volume tends to be a figure larger than the total body volume. The condition of critically ill patients tends to complicate matters even further. Patients may have dramatic alterations in their circulating volume, and the manner in which they were resuscitated can have implications for many pharmacologic agents. Patients receiving mainly crystalloid infusions will have a large volume of distribution for hydrophilic substances. Chronically ill patients will have conditions affecting their volume of distribution as well. Congestive heart failure may lead to fluid retention, increasing the Vd for hydrophilic agents. Chronic renal failure may lead to a similar increase in Vd via fluid retention, and conditions such as the nephrotic syndrome can lead to hypoalbuminemia, decreasing the Vd for highly protein-bound substances. Patients with hepatic failure and resultant productive protein deficits will also exhibit hypoalbuminemia as well as hypogammaglobulinemia, both essential binding sites for protein-bound drugs. Chronic or critical illness can have profound effects on the body’s normal homeostatic mechanisms, often times leading to a hypercatabolic state. This can lead to muscle wasting and loss of lean body mass. This can have implications for substances that exhibit both protein binding and lipophilic peripheral tissue binding.
Lastly, critically ill patients can exhibit violations in normally contiguous boundaries between various bodily compartments which can have implications on both an agent’s site of intended action and the possibility of acting upon an unintended compartment, which can lead to severe consequences. Examples of this include the diffuse capillary leak seen in sepsis, the localized pulmonary endothelial disruption seen in patients with the acute respiratory distress syndrome (ARDS), and disruptions of the blood-brain barrier by hemorrhage, tumor, or trauma. Prior knowledge of these disease states can have profound impact on an individualized pharmacologic regimen. For example, patients with elevated intracranial pressure may receive the osmotic diuretic mannitol in an attempt to draw interstitial fluid from the brain across the impermeable membrane of the blood-brain barrier. If this medication is given to a patient with elevated intracranial pressure with a disrupted blood-brain barrier, which can be the case with an intraparenchymal hemorrhage, the osmotic load will then be delivered to the brain parenchyma itself. This can lead to the disastrous consequence of localized tissue edema and an unintended increase in intracranial pressure.
Clearance
The body’s metabolism and disposal of a drug can be drastically altered by critical illness. Disruptions in the major metabolic and excretory organs such as the liver and kidneys as well as alterations in both regional and systemic circulation can all affect the efficiency in which a drug is ultimately cleared.
Renal insufficiency, whether chronic or acute, can have multiple implications on drug clearance. Substances which are eliminated unchanged in the urine will remain in circulation and therefore have a prolonged half-life. This can be said about digoxin, insulin, and the aminoglycoside antibiotics. Some drugs are not metabolized directly by the kidneys but may rely on them for excretion of active metabolites. These metabolites, as in the case of morphine and its active metabolite morphine-6-glucuronide, can contribute to a prolonged effect when they are not efficiently cleared by the failing kidneys. Since renal clearance is dependent on glomerular filtration, often times the extent of impairment in renal clearance can be estimated with reasonable accuracy by the decrease in calculated creatinine clearance, which can be used to estimate glomerular filtration rate (GFR). Dosing adjustments for patients with lower GFR can then be made that result in either a decreased dose or increased dosing interval. For drugs with low therapeutic indices, this would ideally be done in conjunction with measurement of plasma levels. A major determinant of how much drug within the intravascular compartment reaches the processing and filtration sites within the kidney is systemic blood flow, as the kidneys normally receive about 25 % of the cardiac output. Patients with heart failure may fail to produce enough cardiac output to support the systemic circulation, and septic patients may exhibit profound decreases in peripheral vascular resistance. Either of these scenarios may result in decreased glomerular filtration and renal clearance. In the case of patients with fulminant renal failure requiring renal replacement therapy, the question often arises whether or not an administered drug will be filtered out into the dialysate. In general, agents that are highly water soluble, of low molecular weight, and with low Vd that do not display significant protein binding are thought to be susceptible to either hemodialysis or peritoneal dialysis. Other important factors to consider include the area and porosity of the dialyzing membrane.
In contrast to the relative predictability of renal insufficiency’s effect on drug clearance, the patient with liver failure presents a challenge in the interpretation of to what extent their hepatic dysfunction may alter drug metabolism and elimination. The first level of complexity lies in the fact that for some substances, hepatic clearance is dependent not only on the inherent metabolic capability of the hepatocellular CYP450 system but on hepatic blood flow as well. Drugs with a high hepatic extraction ratio, meaning that they are efficiently metabolized and cleared by hepatocytes, are more affected by delivery via hepatic blood flow than metabolic capacity and are therefore classified as flow-limited. Critically ill patients may have multiple etiologies of decreased hepatic blood flow such as portal venous thrombosis, constriction of the splanchnic circulation from vasopressor therapy, or fibrosis of the liver from cirrhosis, therefore providing an intraparenchymal obstruction to hepatic blood flow. Substances which are less efficiently processed may rely more on the availability of hepatocytes for metabolism and are thought to be capacity limited.
Secondly, indices of hepatic dysfunction such as the Child score or MELD score do not readily correlate with the diseased liver’s ability to conjugate and biotransform substances. The myriad of effects seen in patients with hepatic disease including the decreased production of albumin and gamma globulin and therefore reductions in drug protein binding can also have effects on clearance, mainly for drugs that are capacity limited. Finally, cirrhosis has been shown to have varying effects on different enzymes within the CYP450 system. While some enzymes’ activity may be drastically reduced, others may not be affected or even show increased activity in cirrhosis.
Discussion of Commonly Used Agents
Sedatives, Analgesics, and Anxiolytics
Critical illness, as well as associated therapeutic measures, is often times characterized by severe discomfort, anxiety, or delirium. Each of these conditions has the potential to pose a significant barrier to both the intended outcome of a patient’s care and also its implementation. Therefore, agents within this particular class are some of the most commonly prescribed to patients in the ICU, and knowledge of their action and potential side effects is paramount to their successful use.
Midazolam
Midazolam is a short-acting benzodiazepine used most commonly in the ICU as a continuous infusion for patients who are mechanically ventilated. A selective GABAA agonist, midazolam’s intended effect is to produce sedation and anxiolysis, which may improve the patient’s ability to tolerate the often times uncomfortable sensations that occur with mechanical ventilation. Its short elimination half-life observed in healthy individuals made it an ideal candidate for sedation provided by continuous infusion. However, critically ill patients have been observed to exhibit much more prolonged durations of sedation than their healthy counterparts [4]. In fact, some studies showed that patients were still fully sedated more than 10 h after the cessation of a midazolam infusion [3]. Suggestions for the etiology of this prolonged effect include an increased Vd or decrease in renal clearance seen in critically ill patients, the latter of which may also lead to an accumulation of active metabolites [2]. Prolonged, uninterrupted periods of sedation in critically ill patients may lead to an increased risk of delirium, a major predictive risk factor of overall ICU-related mortality, and can cause confusion in the interpretation of neurologic exam findings. For these reasons, midazolam may be slowly falling out of favor in its utility as a first-line medication for providing sedation in critically ill patients.
Propofol
Propofol is a short-acting, lipophilic diisopropylphenol used mainly for the induction and maintenance of general anesthesia. While its exact mechanism of action is unknown, the prevailing theory is that it acts to enhance the activity of GABAA receptors in the central nervous system [1]. The rapid emergence shown in patients in whom propofol has been administered has proven favorable in the sedation of critically ill patients. It exhibits a very low context-sensitive half-life, which means that even patients receiving prolonged continuous infusions will still display rapid emergence. Its rapid clearance is performed mainly via the liver with flow-limited characteristics, and little change in its elimination profile has been reported in patients with hepatic or renal disease. Recently, a specific potential for harm in patients receiving prolonged infusions of propofol has been described involving severe bradycardia leading to asystole, metabolic acidosis, and rhabdomyolysis. This particular grouping of symptoms in relation to a propofol infusion has been labeled the propofol infusion syndrome (PRIS), and as many as 38 fatalities have been reported. PRIS has been found to be associated with infusions of more than 4 mg kg−1 h−1 for over 48 h and is thought to be potentially due to propofol-induced mitochondrial dysfunction [5, 6]. Predisposing factors include young age and severe critical illness of the neurologic or respiratory system [7]. These findings have led to a revaluation of sedation protocols for critically ill patients who may require high doses of sedatives over a prolonged period. An additional area of concern for intensivists regards propofol’s necessity for delivery in a lipid emulsion, which can contribute to a patient’s dietary intake. The lipid emulsion can be thought of as similar to a 10 % parenteral lipid emulsion, amounting to 1.1 kcal/ml of fat [8]. In patients requiring large volumes of propofol to achieve adequate sedation, this can theoretically lead to overfeeding if other means of nutrition are not properly adjusted.
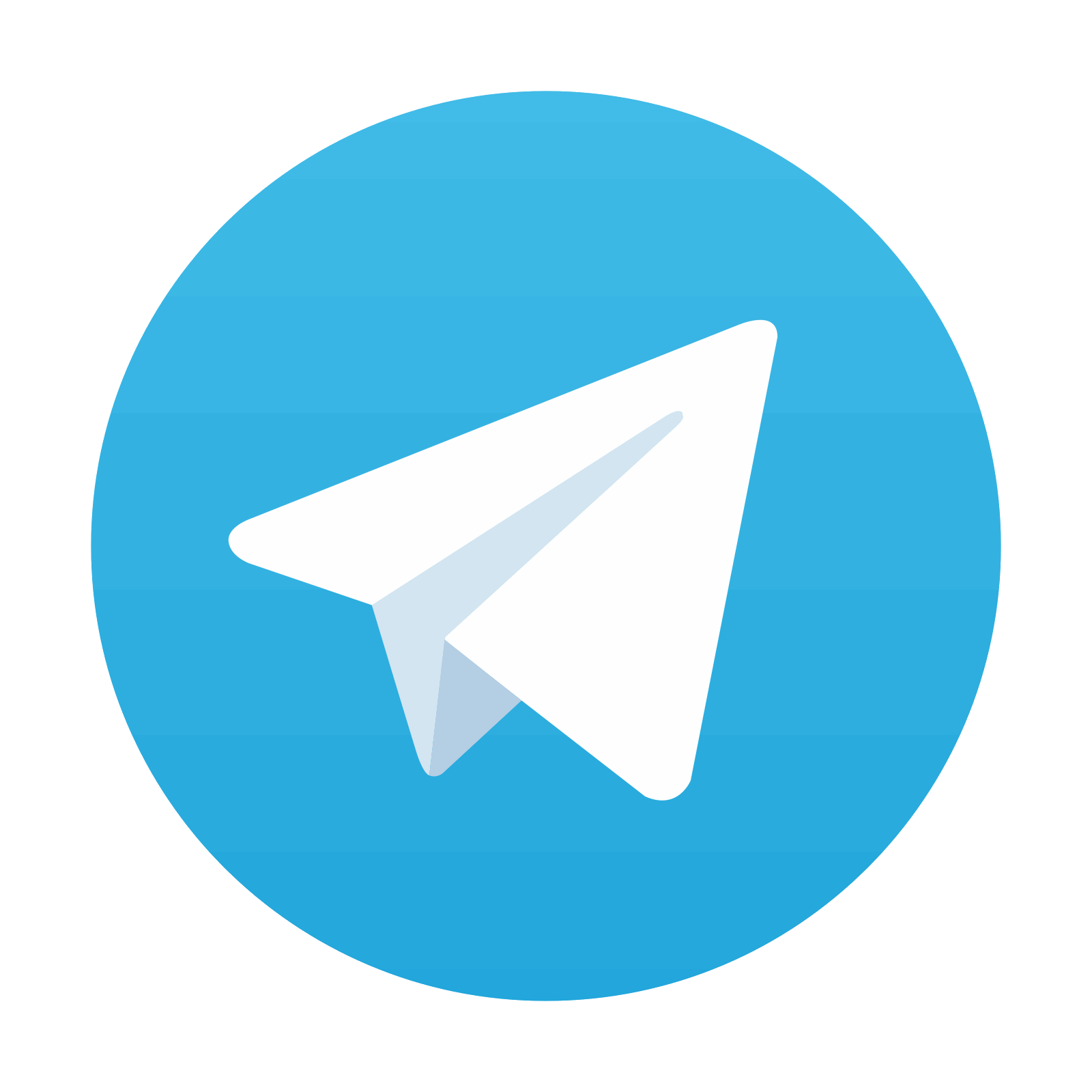
Stay updated, free articles. Join our Telegram channel

Full access? Get Clinical Tree
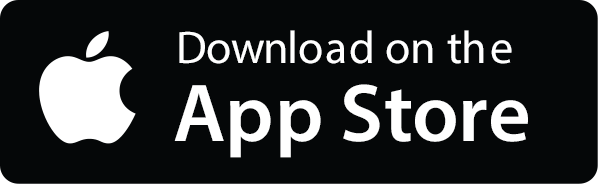
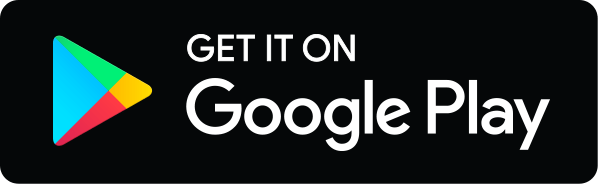
