SPINAL COLUMN AND SPINAL CORD INJURY
Injuries to the spine, including injuries to the vertebral column, ligaments, and cord, are common. Spine injuries occurred in 106,762 patients in 2008, representing 17% of the population of patients reported to the National Trauma Data Bank.1 According to the Foundation for Spinal Cord Injury Prevention, Care and Cure, the incidence of spinal cord injury, excluding those who die at the scene, is approximately 40 cases per million population in the United States, or approximately 12,000 new cases per year.2
Spinal cord injury is a disease of young male adults. The average age at injury during the 1970s was approximately 28 years with most injuries ranging between the ages of 16 and 30. However, as the median age of the population has increased, the average age at injury has also steadily increased to approximately 42 years in 2005. Spinal cord injury affects males more commonly than females, with a ratio of 4 to 1. The most common etiology of spinal cord injury in the United States is motor vehicle crashes (42.1%) followed by falls and acts of violence (primarily gunshot wounds). The proportion of injuries due to sports has decreased over time.2
Injuries to the spinal cord are devastating: 30% of patients have incomplete tetraplegia, 25% have complete paraplegia, 20% have complete tetraplegia, and 18.5% have incomplete paraplegia. Less than 1% of patients experience functional neurologic recovery by the time of hospital discharge. The median hospital length of stay in an acute care hospital has declined over the last three decades, averaging 12 days in the years 2005-2008. Similar downtrends are noted for days in rehabilitation, averaging 37 days in the years 2005-2008. Overall median hospitalized days including acute care and rehabilitation were greater for neurologically complete injuries.2
Spinal cord injuries represent a major societal economic burden. Although more than half of patients with spinal cord injury (57.5%) report being employed at the time of their injury, this drops significantly to 11.5% at 1 year postinjury. Additionally, in 2008 dollars, the average first year postinjury costs range from $236,109 to $801,161 with each subsequent year’s cost ranging from $16,547 for incomplete motor function at any level to $143,507 for a patient with high tetraplegia (C1-C4). These costs noted are only direct costs and do not acknowledge the indirect costs, including loss of future wages, fringe benefits, and productivity. As >95% of patients survive their initial hospitalization, the lifetime costs of injury in a young spinal cord injury patient with a high cervical spine injury (C1-C4) are in excess of $3,160,000. Indirect costs have been estimated at approximately $64,443 per year in December of 2008.2
Spine injuries occur in mobile spinal segments: the cervical spine and the thoracolumbar spine. In the cervical spine, 25% of all injuries occur in the upper cervical spine, while 75% occur between C3 and C7. Multiple-level spine injuries have been estimated to occur in 4%-20% of all cases. In the thoracolumbar spine, the anatomic distribution of injury is most common around the thoracolumbar junction.
The most common spine fracture is a vertebral body compression fracture. Anterior column wedge fractures are the most common type of compression fractures and are the typical compression fractures seen in osteoporotic patients. Fractures occurring from major flexion and distraction forces often disrupt the posterior ligamentous complex causing these fractures to be relatively unstable. These fractures are often caused by lap belts in motor vehicle accidents. High-energy axial loads to the spine may cause compression fractures with retropulsion of bone fragments into the canal. These fractures are called burst fractures, which have a higher risk of causing neurologic deficit and instability. A combination of compression, tension, rotation, and/or shear forces causes fracture dislocation injuries that disrupt all three columns of the spine. Fracture dislocations are the most unstable type of spinal fracture.
Cervical spine injuries occur in a bimodal fashion, involving either the young (age 15-45 years) or elderly (age 65-85 years).3 While cervical spine injury is more common in younger patients, the elderly (age 50-80) are more likely to sustain thoracolumbar fractures.4
The main tenet of prehospital care for spinal cord injury is effective spinal immobilization to prevent further injury. It is estimated that 3%-25% of spinal cord injuries occur after the initial trauma, either during transport or in the initial phases of evaluation and treatment. Effective spine immobilization includes the use of a rigid cervical collar and a backboard, with the head of the patient secured to the backboard with head blocks and straps. The practice of immobilization with sandbags and tape is not recommended.5,6
BONY AND LIGAMENTOUS ANATOMY OF THE VERTEBRAL COLUMN
The vertebral column, which along with the skull and rib cage comprises the axial skeleton, provides support and protects neural elements. It consists of 33 vertebrae, 24 of which are mobile and 9 immobile. The mobile vertebrae include 7 cervical, 12 thoracic, and 5 lumbar vertebrae. The immobile vertebrae include 5 sacral and 4 coccygeal vertebrae, which are fused to form the sacrum and coccyx, the caudalmost portion of the vertebral column.
The adult spine has natural curves in the sagittal plane that form an S-shape. This S-shape is formed by lordosis in the cervical and lumbar regions and kyphosis in the thoracic region. This natural curvature of the spine allows for shock absorption, maintenance of balance, and body movement. The basic motion segment is comprised of two adjacent vertebral bodies, intervening disc, facet joints, and the stabilizing ligaments. These spinal motion segments allow flexibility and motion while maintaining stability.
Because of anatomic and kinematic differences, the cervical spine is often differentiated into upper (C1-C2) and lower (C3-C7) regions. The pedicles of C2 are a transition zone between the upper and subaxial spine and are oblique in order to connect the anterior C1 facets to the posterior C3 facets. The occiput and the upper cervical spine form a complex and highly mobile unit. C1 articulates with the occipital condyles as well as the dens and facets of C2. The occiput–C1 articulation allows for flexion–extension and lateral bending. C2, also known as the axis, has a distinctive odontoid process that articulates with the anterior arch of C1 and is stabilized by the transverse ligament. The C1-C2 (atlantoaxial) articulation allows for 50% of head rotation. The vertebrae of C3-C7, also known as the subaxial cervical spine, are more uniform and, because of the shingle-like orientation of the facets, allow primarily flexion and extension in this region.
The 12 vertebrae of the thoracic spine form a long, rigid column with limited motion due to their attachments to the rib cage and sternum. The thoracolumbar junction is a transition zone from the rigid thoracic spine to the mobile lumbar spine. In this region, the facet joints gradually change from a more coronal orientation to a sagittal orientation. The five lumbar vertebrae are the largest vertebrae in the spine. The facets of the lumbar spine are oriented at 45 degrees of sagittal angulation allowing flexion–extension, rotation, and lateral bending. The lumbosacral junction is another transition zone between mobile and rigid segments that has significance when surgical stabilization procedures are being considered.
Spinal ligaments and intervening discs stabilize the mobile segments of the vertebral column. In the cervical spine, the intervertebral discs and the anterior and posterior longitudinal ligaments stabilize the anterior column. The posterior column is stabilized by the facet capsules, ligamentum flavum, interspinal and supraspinal ligaments, and the ligamentum nuchae. The odontoid process of C2 is stabilized by the alar, transverse, and apical ligaments.
First described by Denis, the spine can be divided into three longitudinally oriented columns.7 The anterior column includes the anterior longitudinal ligament, the anterior annulus, and the anterior half of the vertebral body. The middle column includes the posterior longitudinal ligament, posterior annulus, and the posterior half of the vertebral body. The posterior column is comprised of the pedicles, facets, laminae, and posterior ligamentous complex (Fig. 24.1).
FIGURE 24.1 A: Noncontrast axial CT and (B) schematic representation of a vertebral body highlighting the relevant anatomy (Reprinted with permission from Blumenfeld H. Neuroanatomy through Clinical Cases. Sinauer Associates; 2002).
ANATOMY AND PHYSIOLOGY OF THE SPINAL CORD
The spinal cord is an extension of the brainstem that begins at the foramen magnum and continues down through the vertebral canal to the first lumbar vertebra, where the spinal cord tapers to form the conus medullaris. A cluster of lumbosacral nerve roots below the conus medullaris forms the cauda equina. Along the length of the spinal cord, ventral and dorsal nerve roots emerge together bilaterally to form spinal nerve roots, exiting through the neural foramen at each intervertebral space.
The spinal cord is held in position at its inferior end by the filum terminale, an extension of the pia mater that attaches to the coccyx. Along its length, the spinal cord is held within the vertebral canal by denticulate ligaments that are lateral extensions of the pia mater attaching to the dural sheath. The subarachnoid space between the spinal cord and dura is an extension of, and communicates directly with, the subarachnoid space around the brain, containing cerebrospinal fluid (CSF) that acts as a shock absorber.
The spinal cord has variable diameters along its length. In the upper spinal cord, between C4 and T1, the spinal cord widens as the cervical enlargement, which is where spinal nerves innervating the upper extremities originate and terminate. The lumbar enlargement, between T9 and T12, is a widening in the lower part of the spinal cord where spinal nerves innervating the lower extremities originate and terminate.
The cross-sectional anatomy of the spinal cord consists of white matter located on the periphery and gray matter located centrally. The white matter contains myelinated axons that form the ascending and descending spinal tracts. The gray matter contains primarily neurons and unmyelinated axons and is divided into three “horns”: anterior, posterior, and intermediate. The anterior horns contain lower motor neurons that innervate the muscles of the neck, trunk, and extremities. The upper motor neurons that synapse with these lower motor neurons originate in the contralateral cerebral cortex or brain stem and descend as the lateral and anterior corticospinal tracts prior to synapsing in the anterior horn. The posterior horns contain neurons that synapse with afferent sensory axons. The intermediate horns contain the preganglionic autonomic sympathetic neurons between T1 and L3 and the parasympathetic neurons between S2 and S4 (Fig. 24.2).
FIGURE 24.2 A: Noncontrast axial MRI and (B) schematic representation of the spinal cord highlighting the major anatomic tracts.
Sensory signals originate in somatic or visceral receptors and are transmitted via axons to the dorsal root ganglion. From there, sensory afferent axons enter the posterior horns of the gray matter and travel within varied regions of spinal cord white matter toward sensory regions of the brain. Pain and temperature signals cross immediately and ascend in the contralateral lateral spinothalamic tract. Touch also crosses immediately and ascends mainly in the anterior spinothalamic tract. Proprioception and vibration ascend in the ipsilateral dorsal column and cross in the brainstem. The dorsal columns are divided into the fasciculus gracilis and the fasciculus cuneatus. The fasciculus gracilis is located medially and carries sensory signals from the lower extremities, whereas the fasciculus cuneatus is located laterally and carries sensory signals from the upper extremities.
ACUTE VERTEBRAL COLUMN INJURIES
Spinal Instability
The determination of spinal instability is a complex and controversial subject, and spinal instability is often difficult to precisely ascertain. A widely accepted definition of clinical instability is the loss of the ability of the spine, under physiologic loads, to maintain its pattern of displacement so that there is no initial or additional neurologic deficit, no major deformity, or no incapacitating pain.8 Although many theories of spinal instability have been proposed, Denis’ previously described three-column theory is most widely accepted.7 The integrity of the middle column is of the utmost importance as an injury to two adjoining columns defines an unstable fracture. Punjabi and colleagues have validated the three-column theory of instability using high-speed trauma models of thoracolumbar spinal fractures and demonstrated that the integrity of the middle column correlated best with eight of nine flexibility parameters.9
Occipitoatlantal Dislocation
Among traumatic injuries to the cervical spine and causes for occipital–cervical instability, occipitoatlantal dislocation (OAD) is one of the most severe. A hyperflexion–distraction injury resulting in ligamentous disconnection of the skull from the cervical spine, OAD is often immediately fatal because of associated neurologic and vascular injuries. Any high-impact injury should arouse suspicion of OAD. Although plain radiographs of the cervical spine may reveal prevertebral soft tissue swelling and an increase in the basion–dens interval, which should measure 12 mm or less, more definitive diagnosis is made with reconstructed computed tomography (CT) images of the craniocervical junction. Magnetic resonance imaging (MRI) and CT are comparable in terms of diagnosing OAD, but MRI identifies the specific ligaments injured. OAD injuries can be defined in three broad categories. Type I injuries are characterized by anterior displacement of the occipital condyles on the C1 lateral masses; Type II injuries are characterized by displacement of the occiput and C1 in the vertical plane, and Type III injuries are marked by posterior displacement of occipital condyles compared to C1. These injuries are rare and usually fatal. If the patient survives and there are no contraindications, posterior occipital–cervical stabilization and fusion are required.
Occipital Condyle Fractures
The Anderson and Montesano scheme classifies occipital condyle fractures into three main types.10 These fractures are seen in 1%-3% of blunt trauma to the craniocervical region. Type 1 fractures are comminuted, and result from axial loading injuries. Type II fractures are linear fractures that originate in the squama of the occipital bone and extend into the condyle. Type III fractures are avulsion fractures of the condyles and are most prone to instability and OAD. While types I and II fractures are generally stable, type III fractures are potentially unstable. Stable fractures can be treated in a cervicothoracic brace; however, unstable fractures mandate stabilization and fusion.
BONY AND LIGAMENTOUS INJURIES TO THE CERVICAL SPINE
C1 Fractures and Transverse Ligament Injuries
Fractures of the atlas are usually defined in relation to the lateral masses and extent of arch involvement.11 They can involve any parts of the ring in isolation or in combination, ranging from single unilateral fractures to burst-type fractures involving all aspects, also known as a Jefferson fracture. Since isolated atlas fractures without ligamentous injury are stable and heal with simple immobilization, clinical decision making is based on the identification of injuries to the transverse ligament, the vertebral artery, and other associated spinal fractures. The most commonly cited radiographic criteria indicating unstable disruption of the transverse ligament include the rule of Spence (lateral displacement of C1 lateral masses over C2 > 6.9 mm) and the atlantodental interval > 3 mm.12,79 However, when feasible, this author prefers MRI evaluation of all atlas fractures to assess for concomitant ligamentous injury, which is an indication for surgical fixation.
Atlantoaxial Joint Injuries
Atlantoaxial subluxation occurs when the transverse ligament is disrupted. This is an extremely unstable injury with a high risk for neurologic deficit. Traction may be appropriate initially to achieve reduction. Definitive treatment mandates posterior cervical fusion of C1-C2.
C2 Fractures
Odontoid process fractures commonly affect the elderly. The most common classification scheme for fractures of C2, the Anderson and D’Alonzo scheme, relies on the location of the fracture line within the odontoid process or body of C2.13 In this scheme, type I fractures involve the tip of the dens, type II fractures run through the junction of the dens and the body of C2, and type III fractures course through the vertebral body of C2 (Fig. 24.3 and Fig. 24.4).
FIGURE 24.3. Schematic representation of the three types of odontoid fractures: (A) type 1, (B) type 2, and (C) type 3.
FIGURE 24.4. Noncontrast sagittal (A) and coronal (B) CTs demonstrate a type 2 odontoid fracture (arrows).
Management of C2 fractures is determined by type. Type I fractures are an avulsion of the alar ligament and are usually stable. Cervical collar immobilization for symptomatic management is usually sufficient. Type II fractures are the most common type of dens fracture and are more often subject to nonunion, especially in patients older than 50 years of age and those with displacement >5 mm. When choosing treatment strategies for type II fractures, the integrity of the transverse ligament, age and orientation of the fracture, displacement and/or angulation of the fractured process, and patient-specific factors such as medical comorbidities, and body habitus should be considered. Type III fractures extend into the C2 vertebral body. This fracture type can be mechanically unstable but generally heals well with immobilization. As such, treatment usually entails cervical immobilization in either a rigid cervical orthosis or a halo vest for 12 weeks.
Fractures of the C2 pedicles (also known as traumatic spondylolisthesis or hangman’s fractures) are often classified based on the mechanism of injury, where flexion (type III) and flexion–distraction (type IIa) are often unstable and require surgical fixation, especially type IIa injuries with >4 mm distraction and/or >11 degrees of angulation.15,76 Other fractures of the axis can include isolated fractures of the C2 vertebral body or fractures of the C2 spinous process or lamina, which are usually stable and can achieve good union with nonoperative immobilization.
Injuries of the Subaxial Cervical Spine (C3–C7)
Subaxial cervical spine injuries include fractures, dislocations, subluxations, and a combination of these injuries. Unilateral facet injuries can cause nerve root irritation and radiculopathy, whereas bilateral facet injuries frequently cause spinal cord compromise. Both unilateral and bilateral facet disruptions are treated initially with closed reduction with traction. If this reduction can be maintained, unilateral injuries can be definitively treated with halo immobilization. Bilateral facet injuries, however, are grossly unstable and require surgical fusion for definitive treatment.
Compression Fractures. Compression fractures are anterior column injuries with wedging of the anterior portion of vertebral body. The posterior portion of the vertebral body and the spinal canal remain intact. As a result, neurologic compromise is rare. These are generally stable injuries, but if angulation exceeds 11 degrees, wedge compression exceeds 25% of the anterior vertebral body, or translation exceeds 2.5 mm, brace immobilization may not be sufficient. If the anterior vertebral body wedge compression exceeds 50%, there is a greater likelihood that the posterior ligamentous complex has been disrupted. This unstable form of compression injury requires surgical stabilization.
Burst Fractures. Burst fractures are characterized by disruption of both the anterior and middle columns, have some degree of spinal canal compromise, and are commonly associated with neurologic deficit and instability. These injuries are caused by a large axial load applied to a cervical spine while in a straight position. If there is no neurologic deficit or malalignment, halo immobilization may be an acceptable treatment. If, however, neurologic deficit is present and/or reduction cannot be achieved, anterior decompression and fusion is indicated. Anterior and posterior stabilization may be required if there is associated posterior ligamentous injury.
Other Cervical Fractures. Other common cervical injuries include lateral mass fractures, laminar fractures, spinous process fractures, flexion tear drop fractures (Fig. 24.5), extension tear drop fractures, and ligamentous injuries. These are generally stable injuries except for flexion tear drop fractures, which result from a flexion–compression mechanism. In this very unstable fracture, all three columns are involved, and there is a high incidence of neurologic compromise. In contrast, extension tear drop fractures are a stable avulsion injury involving only the anterior column.
FIGURE 24.5. Noncontrast sagittal CT (A) demonstrates a C5 tear drop fracture (arrow). Postoperative AP radiograph of the cervical spine (B) with anterior and posterior fixation hardware in place.
Injuries to the Thoracic Spine
Unlike the cervical and lumbar spine, the thoracic spine is inherently stable. As a result, thoracic spine fractures are usually caused by high-energy mechanisms, and associated injuries occur in about 75% of patients. Stable thoracic spine fractures without neurologic deficit can often be treated nonsurgically with bracing or vertebroplasty/kyphoplasty (Fig. 24.6). Unstable thoracic spine fractures are usually associated with neurologic deficit and malalignment and require surgical reduction and stabilization (Fig. 24.7).
FIGURE 24.6. Noncontrast sagittal CT demonstrates a chronic T10 compression fracture, status postkyphoplasty (double arrow), as well as acute compression fractures at T12 (single arrow) and L3 (dashed arrow).
FIGURE 24.7. Noncontrast axial (A) and sagittal (B) CTs demonstrate an L1 burst fracture with retropulsion of bone fragments into the spinal canal.
Injuries to the Thoracolumbar Spine
Thoracolumbar spine fractures are extremely common due to the relatively mobile characteristics of this region compared to the adjacent rigid thoracic spine and sacrum. Fractures in this location include compression fractures, burst fractures, fracture–distraction injuries, and fracture dislocation injuries. Flexion–distraction injuries consist of both bony and ligamentous disruption without loss of vertebral height. Most of these injuries are treated surgically, especially if there is substantial disc or ligamentous involvement. Fracture dislocations consist of varying degrees of rotation, flexion, and translation and are extremely unstable injuries. The majority of these injuries are associated with neurologic deficit, and require surgical decompression and stabilization (Fig. 24.8).
FIGURE 24.8. Lumbar AP radiograph (A) and noncontrast coronal (B) and axial (C) CTs demonstrate an L2–L3 fracture dislocation with malalignment of the vertebral column Reprinted with permission from: Loftus CM, Macias MY, Wolfla CE. Neurosurgical Emergencies. Thieme Medical Publishers; 2008).
ACUTE SPINAL CORD INJURY
Mechanism of Injury
Based on the mechanism of injury, spinal cord injury can be categorized as direct/penetrating or indirect/blunt injuries. The direct or penetrating injuries are caused by a space-occupying lesion as a result of a focal injury to the neural elements at the site of impact (Fig. 24.9 and Fig. 24.10). These are most often caused by a gunshot wound or a knife laceration and most often result in complete neurologic deficit. The indirect or blunt injuries are caused by stretching, shearing, or compressive forces on the spinal cord (Fig. 24.11). These often occur in the setting of a motor vehicle accident, fall, or sport-related activity and are the most frequent type of spinal cord injury encountered in clinical practice.
FIGURE 24.9. Gunshot wound with bullet in thoracic spinal canal at T4–T5 (Sagittal CT reconstruction).
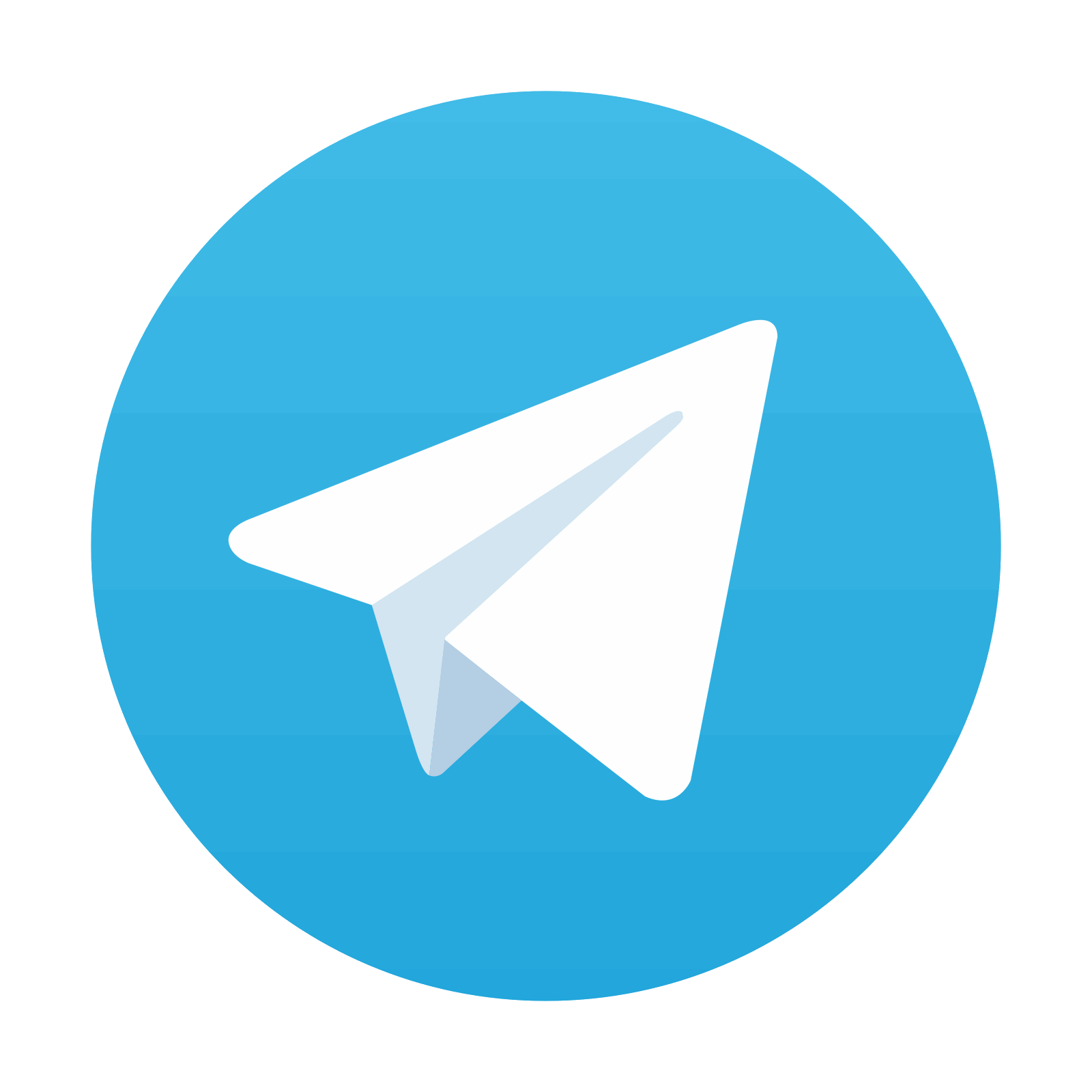
Stay updated, free articles. Join our Telegram channel

Full access? Get Clinical Tree
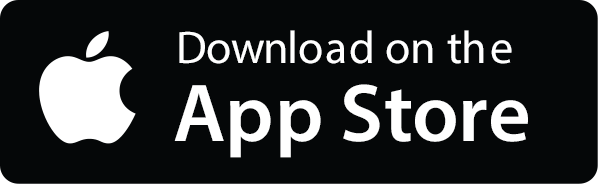
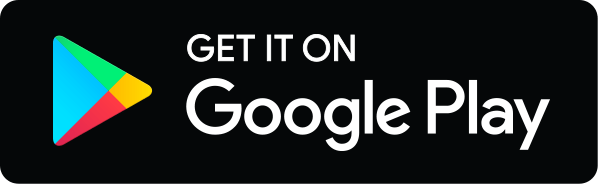