Hemodynamic and Physiologic Effects of Intra-aortic Balloon Pump Therapy
Intra-aortic Balloon Pump Inflation
At the beginning of diastole, the balloon is inflated with helium gas, which efficiently displaces the aortic blood volume and pushes blood toward the aortic root. The increase in aortic root pressure increases flow into the coronary arteries. As a result, myocardial oxygen supply is increased. Distal perfusion pressure is also enhanced as balloon inflation improves renal and mesenteric arterial blood flow. These are two vascular beds that are frequently underperfused with myocardial dysfunction and advanced heart failure.
Intra-aortic Balloon Pump Deflation
Deflation is timed to occur just prior to the next systolic ejection of the heart. The displacement of blood from the aorta reduces impedance to left ventricular (LV) ejection resulting in a larger stroke volume with lower ventricular wall tension (Figure 15-2). The clinical outcome is decreased afterload and reduced myocardial oxygen demand. Indirectly, this will improve contractility. Greater stroke volume per beat results in decreased preload and decreased pulmonary congestion, which are two prevalent features of myocardial dysfunction and advanced heart failure.
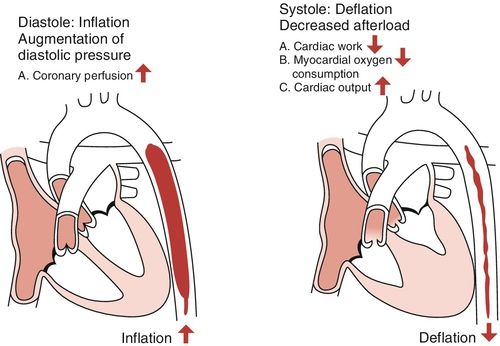
The hemodynamic and physiologic effects of IABP counterpulsation therapy are summarized in Table 15-2. The degree to which the desired hemodynamic effects are achieved will, in part, depend on the size or volume of the balloon, the degree to which the balloon occludes the aorta during diastole, correct positioning of the balloon in the aorta, and how well timed the balloon inflation and deflation are relative to the patient’s cardiac cycle.2
Table 15-2
Physiologic and Hemodynamic Effects of IABP Inflation and Deflation
Clinical or Hemodynamic Indicator | Inflation | Deflation |
↑ Coronary blood flow | +++ | None |
↓ Symptoms and signs of ischemia Angina ST segment changes Arrhythmias | +++ | +++ |
↓ Afterload | None | +++ |
↓ Preload (PAOP) | + – ++ | +++ |
↓ Heart rate (indirect effect) | ++ | ++ |
↑ Cardiac index | + – + | +++ |
↑ Urine output (indirect effect) | + – ++ | +++ |
↓ MVO2 | + – ++ | +++ |
+ = mild
++ = moderate
+++ = major
IABP, Intra-aortic balloon pump; MVO2, myocardial oxygen demand; PAOP, pulmonary artery occlusion pressure (wedge).
To achieve ideal counterpulsation, the balloon must have adequate volume relative to the size of the patient. Ideally, the inflated balloon should occlude 95% of the aorta to increase coronary blood flow during inflation and achieve optimal afterload reduction during deflation. The degree to which this is achieved depends on how well the balloon size fits the diameter of the aorta. Balloon sizes range from 30 to 50 mL in volume in adult patients. A person with a height less than 5 feet and 2 inches would most likely require the 30-mL size because the larger balloon will extend too far down into the abdominal aorta potentially inflating against a calcified aorta, thus increasing the risk of balloon rupture. A lower position may also interfere with renal artery blood flow. A person taller than 6 feet and 2 inches would most likely require a 50-mL balloon to ensure adequate blood volume displacement during balloon inflation. For all other individuals, the 40-mL balloon is adequate.
The correct position of the balloon in the aorta is just below the left subclavian artery and above the renal arteries. This position can be visualized on the chest radiograph. The catheter tip should be located 2 centimeters (cm) below the origin of the left subclavian artery at the second to third intercostal space. A position too high in the aorta may interfere with blood flow to the left subclavian artery. A position too low in the aorta may interfere with renal artery blood flow. Routine assessment of the patient with an IABP includes assessment for the presence of the left radial pulse. A change in radial pulse character might be an indication that the balloon is too high in the aorta and limiting left subclavian arterial blood flow. Any unexpected decrease in urine output in the patient should be investigated, as it may result from a low balloon position limiting renal artery blood flow.
Timing of balloon inflation and deflation to the patient’s cardiac cycle is critical for achieving optimal coronary perfusion and afterload reduction. The hemodynamic consequences of balloon mistiming are listed below:
The accurate timing of balloon inflation and deflation is illustrated in Figure 15-3. Present-day IABP consoles use computer or automatic timing. The most accurate IABP timing is achieved with newer fiberoptic catheters.1
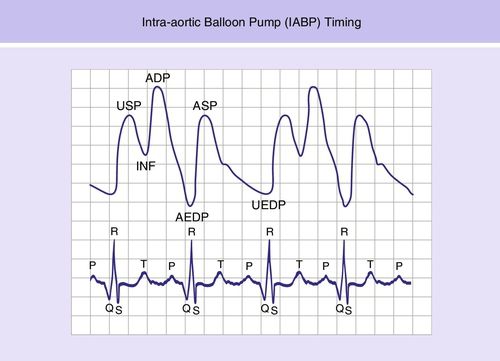
Indications and Contraindications of Intra-aortic Balloon Pump Therapy
IABP therapy is indicated and effective for a variety of cardiac conditions. A frequent application is for the support of low cardiac output following cardiopulmonary bypass. The duration of support is rarely more than 72 hours, by which time the myocardium has recovered from any stunning, edema, or other transient effects of the surgery. Common medical indications include cardiogenic shock from LV failure or following myocardial infarction. IABP therapy can be helpful in supporting patients who have incurred acute mitral regurgitation from papillary muscle injury, or ventricular septal rupture with left-to-right shunting following myocardial infarction. The decreased afterload and increased stroke volume helps decrease the amount of regurgitation and left-to-right shunt from these two mechanical problems until surgical repair. Other indications are intractable angina or myocardial ischemia, refractory heart failure, intractable arrhythmias, and as a bridge to further therapy such as LVAD, heart transplantation, or cardiac surgery.3 IABP therapy is used in some centers as adjunctive therapy for high-risk patients undergoing interventional procedures in the cardiac catheterization laboratory.
Contraindications to IABP therapy are few. Severe peripheral vascular disease is a contraindication because of the risk of the catheter compromising blood flow distal to the cannulated femoral artery. This condition could potentially lead to limb ischemia or compartment syndrome. Other contraindications include the presence of an aortic aneurysm, as well as aortic dissection, since dissection may further increase with higher pressures during diastole. Aortic insufficiency is also a contraindication because the increased pressure during diastole would serve to create greater valve regurgitation. Because patients must be anticoagulated while receiving IABP therapy, any uncontrolled bleeding disorder may be a contraindication for insertion.
Potential Complications of Intra-aortic Balloon Pump Therapy
Complications associated with IABP therapy are well established and primarily vascular in nature, especially in the presence of peripheral arterial disease.2 Common complications are listed in Box 15-1.4–6 In keeping with the theme of this chapter, only those complications that affect hemodynamic effectiveness are discussed. The clinical issues that might impair IABP effectiveness other than what has previously been discussed include hypovolemia and right heart failure. In the cardiothoracic surgery patient, bleeding from extensive surgical dissection, trauma to platelets from cardiopulmonary bypass, and residual effects from anticoagulation may cause hypovolemia, which must be treated with volume replacement before the benefits of improved cardiac index from IABP therapy can be appreciated. A second issue that may preclude hemodynamic improvement from IABP therapy is the presence of significant right heart failure. Increased coronary perfusion may help to a certain extent, but if the clinical problem is primary right heart failure, improved cardiac index cannot be achieved. Left heart afterload reduction might be attained but inadequate filling of the left heart will still result in low output and cardiac index.
Hemodynamic Effectiveness and Limitations in Intra-aortic Balloon Pump Therapy
For decades, IABP counterpulsation therapy has been the first-line MCS for patients with acute myocardial infarction undergoing percutaneous revascularization intervention because of its widespread availability, ease of use, low cost, and relatively low rates of serious complications. However, the utility of IABP therapy has recently been challenged.7 The results of two large randomized controlled trials have called into question the effectiveness of IABP therapy in acute myocardial infarction.
The Counterpulsation to Reduce Infarct Size Pre-PCI Acute Myocardial Infarction (CRISP-AMI) trial compared IABP support before percutaneous coronary intervention (PCI), with standard PCI care in 337 patients with acute myocardial infarction without cardiogenic shock. No difference was observed in infarct size reduction between the two groups.8
The Intra-aortic Balloon Pump in Cardiogenic Shock II (IABP- SHOCK II) trial compared 30-day mortality rates in 595 patients between those with acute myocardial infarction and cardiogenic shock undergoing early PCI revascularization and those with primary PCI alone.9 Pre-PCI insertion of the IABP did not lower mortality at 30 days9 or at 12 months.10 These results can be interpreted in several ways. Perhaps IABP does not have added benefit in acute myocardial infarction without cardiogenic shock as long as the time to coronary revascularization is short;11 or it may be that the SHOCK II trial results indicate that more substantial MCS support is required for myocardial infarction patients with cardiogenic shock. The mortality risk of acute myocardial infarction with cardiogenic shock is about 40%.9 Therefore, it is important to escalate MCS support in patients with refractory cardiogenic shock, as IABP therapy may not provide adequate support.12
Impella Ventricular Assist Device
Microaxial continuous flow pumps provide the next level of temporary MCS support. An example of this type of pump is the Impella device. The two commonly used sizes are the Impella 2.5, and the Impella 5.0. The pump is inserted through the femoral artery and passed in a retrograde manner up the aorta, across the aortic valve, and into the left ventricle under fluoroscopic or echocardiographic guidance. The pump directly off-loads the left ventricle by pulling blood from the LV inflow cannula and propelling it through the outflow into the ascending aorta (Figure 15-4). The pump is mounted on a 9-French (Fr) catheter, which houses a motor driveline and purge line system.
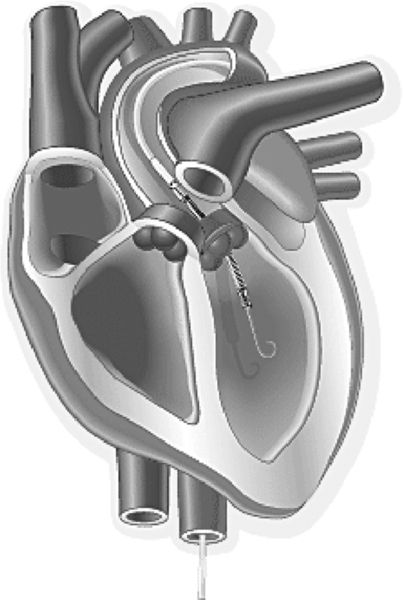
The Impella 2.5 has a pump diameter of 12 Fr and can usually be placed without a surgical cut down. The Impella 5.0 has a pump diameter of 21 Fr that necessitates a femoral arterial cut-down for placement.13 The Impella pumps can also be placed directly into the aorta in patients with cardiogenic shock or low cardiac output syndrome following cardiothoracic surgery. This direct approach utilizes a vascular graft that is sewn directly to the ascending aorta. The pump-mounted catheter is then passed through the graft and directed in a retrograde manner across the aortic valve into the left ventricle. With this approach, only the skin of the chest wound is closed until the pump is removed. The rationale for this chest approach is the rapid initiation of circulatory support in an emergency situation with catastrophic hemodynamic deterioration.14 A newer pump design created for transaortic placement is the Impella LD. It is shorter than the 2.5 and 5.0 pumps and does not have a pigtail at the tip. The catheter provides flows up to 5 L/min. The Impella 2.5 pump is capable of generating up to 2.5 L/min blood flow, and the Impella 5.0 is capable of 5.0 L/min. Another new pump is the Impella CP, which is built on the Impella 2.5 platform but with a larger diameter (14 Fr versus 12 Fr) so that it can provide flows of 3.5 to 4.0 L/min.
Each of these pumps has been used to support patients for periods up to 7 or more days allowing time to develop a more definitive treatment strategy or for recovery to occur.14,15 Despite the large size of these pumps, the incidence of limb ischemia or vascular repair has been similar to that associated with IABP therapy.16,17 The patient must receive anticoagulation therapy. Intraoperative activated clotting times are typically maintained between 160 and 180 seconds to prevent clot formation in the motor.13,15 Postoperatively, partial thromboplastin times are maintained between 40 to 50 seconds with a continuous infusion of heparin.14,15
Hemodynamic and Physiologic Effects of Impella Device Therapy
The propulsion of blood ejected from the left ventricle results in an increase in mean arterial pressure (MAP) and an increase in the cardiac index. Coronary artery perfusion pressure should improve, thus increasing myocardial oxygen supply. Improved forward flow will lower the pulmonary artery occlusion pressure (PAOP). This mechanical unloading of the ventricle reduces LV workload and myocardial oxygen demand.13 As with IABP therapy, improved distal flow and a higher cardiac index should enhance renal and mesenteric arterial blood flow.
Indications and Contraindications of Impella Device Therapy
Current indications for use of the Impella pump include hemodynamic support for high-risk percutaneous interventions, acute ST-elevation myocardial infarction with cardiogenic shock and low output syndrome, or cardiogenic shock following cardiothoracic surgery. Contraindications include severe peripheral vascular disease that would make peripheral insertion difficult and compromise blood flow distal to the insertion site with great risk of limb ischemia. The presence of LV thrombus precludes intraventricular placement of the pump because of the high risk of embolization to the cerebrovascular circulation causing a stroke. The presence of a mechanical aortic valve, moderate to severe aortic valve insufficiency, or aortic valve stenosis are also contraindications.
Potential Complications of Impella Device Therapy
The vascular injury potential of this pump is similar to IABP therapy because of the peripheral cannulation of the femoral artery. Limb ischemia and bleeding complications also have a reported incidence that is similar to IABP therapy.17 Mild hemolysis may occur with the use of any mechanical pump and has been reported in up to 20% of patients.18 Patients receiving higher flows with faster motor revolutions may be at greater risk for hemolysis.
Impaired Hemodynamic Effectiveness and Limitations of Impella Device Therapy
Hypovolemia following cardiothoracic surgery presents a threat to the optimal performance of the pump and can easily be addressed with volume replacement. A greater threat is the development of cardiac tamponade, which would impair LV filling (pump preload) and therefore impair pump output. Relief of cardiac tamponade is a surgical emergency. This event is more likely to occur with transaortic placement of the device.
As with all devices that support the left ventricle, the coexistence of right heart failure will inhibit the ability of the pump to provide optimal output. If the right ventricle has difficulty filling the left heart, there is less volume to support pump flow and a reduced contribution by the left ventricle to overall blood pressure. In this situation, inotropic support would be indicated to support right ventricular (RV) function. Care must be taken during insertion to position the pump away from the papillary muscles and the mitral subvalvular apparatus to avoid damage to those structures (see Figure 15-4). The suction effect generated by the pump might result in inflow obstruction from adjacent tissue. It is also important to maintain adequate anticoagulation to prevent thrombus formation within the pump that would impair function and might even necessitate removal or exchange.
TandemHeart
The TandemHeart is another example of a percutaneous continuous flow MCS system. TandemHeart support is indicated in patients with refractory cardiogenic shock following myocardial infarction, in decompensated heart failure as temporary support until there is ventricular recovery, as a bridge to a more permanent device, or as a bridge to heart transplantation. The TandemHeart circuit includes a venous cannula, a trans-septal left atrium to femoral artery cannula, and an external, nonpulsatile centrifugal pump (see Figure 15-5). Contained within the pump is a six-blade rotating impeller that spins at speeds between 3000 and 7500 revolutions per minute (RPM), generating flows of up to 5 L/min. Placement of the pump is performed in the cardiac catheterization laboratory under fluoroscopic guidance or by direct vision in the case of a cardiothoracic surgery patient.
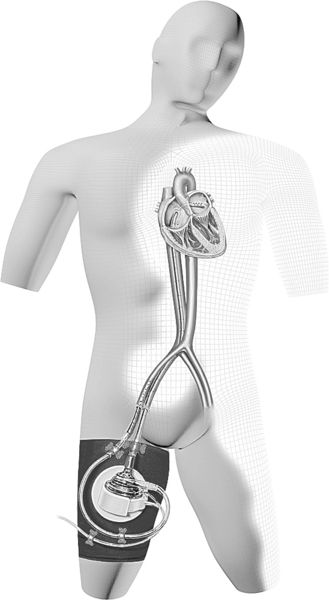
The inflow to the pump is via a 21-Fr femoral venous cannula that is advanced up the inferior vena cava to the right atrium. The cannula is then advanced across the atrial septum into the left atrium via septotomy. The end of the cannula has 14 side holes that allow oxygenated blood to be pulled from the left atrium to the extracorporeal centrifugal pump.13 It is important to maintain the cannula in the correct position with 3 cm traversing the septum. The pump returns blood to the femoral or iliac arterial systemic circulation. A 14-Fr to 19-Fr cannula is used for this purpose. The position of the pump and the transseptal cannulation of the left atrium is shown in Figure 15-5. It is critical not to jeopardize the position of the left atrial cannula. For that reason, patients are relatively immobile during TandemHeart support.
Patient positioning concerns with regard to this pump include recommendations that patients be tilted rather than turned, that the cannulated extremity not be flexed, and that the head of the bed not be elevated more than 20 degrees. Cooperative patients require only light sedation, but some patients may require extended sedation to limit mobility for their safety. These are reasons this therapy has a limited duration of only several days. Anticoagulation is required when this pump is in use. Activated clotting times are maintained between 180 and 220 seconds with anticoagulation. Anticoagulation is titrated to maintain partial thromboplastin times between 65 and 85 seconds in the post-insertion period.
Hemodynamic and Physiologic Effects of TandemHeart Therapy
The TandemHeart is capable of significant pressure and volume unloading of the left ventricle. It can relieve LV distention and decrease ventricular wall strain, which are important components of myocardial oxygen demand. The LV unloading will also unload the right ventricle, improving right heart function, as evidenced by a decrease in the central venous pressure. The improved cardiac index will enhance the support of end-organ function with increased tissue perfusion. These effects have been reported in patients with cardiogenic shock, high-risk interventional cardiology patients, and cardiothoracic surgery patients.19–21
As stated, it is critical to prevent dislodgement of the left atrial cannula. A sudden drop in peripheral oxygen saturation is a first indication of cannula malposition, which can be confirmed with an arterial blood gas (ABG) measurement. This event is an emergency. If the catheter has slipped back into the right atrium, deoxygenated blood will be pulled from the right atrium and returned to the systemic circulation. In addition, the acute left-to-right shunt from the left atrium to the right atrium will volume-overload the right ventricle. Resuscitation with chest compressions may dislodge the left atrial cannula and also pose a risk of left atrial perforation; if needed, such resuscitation should be performed under the supervision of a physician.
Indications and Contraindications for the TandemHeart
Indications for the use of the TandemHeart include refractory cardiogenic shock in ST-segment elevation myocardial infarction, as a bridge to recovery, or a bridge to decision, allowing time to determine whether to offer a more durable form of support for longer-term therapy.
Contraindications to the use of this pump include severe peripheral vascular disease, as is the case for the other peripherally inserted therapies previously discussed. Left atrial thrombosis can be a source of systemic emboli. The degree of anticoagulation required for this pump is greater than the Impella or the IABP, so any coagulopathy might be a contraindication. Since the pump primarily addresses LV function, severe right heart failure is a contraindication if the right ventricle is not capable of adequately filling the left side of the heart.
Potential Complications of TandemHeart Therapy
The placement of a large cannula in the femoral artery creates a risk of lower limb ischemia. Cannulation of the femoral artery brings a risk of artery dissection. Other complications reported in the TandemHeart Registry include groin hematomas, bleeding around the cannula sites, sepsis, gastrointestinal bleeding, coagulopathy, and stroke.22 Given the larger bore of the TandemHeart catheters, infection at the insertion site is a much greater risk than with the previously described devices. These patients are also much more likely to require blood transfusions compared with patients receiving IABP or Impella support.
Impaired Hemodynamic Effectiveness and Limitations of TandemHeart Therapy
As with other devices, hypovolemia will impair pump performance, so it is important to assess the patient’s fluid volume status regularly. This assessment is critical to all assist devices. Tamponade may occur if the left atrial wall is perforated. Randomized controlled trials of the TandemHeart with mortality as an outcome are not available, possibly because the TandemHeart is rarely inserted as an elective procedure.12
CentriMag
Like the TandemHeart, the CentriMag device is an extracorporeal, electrically driven, continuous-flow, centrifugal pump designed for short-term use in patients in cardiogenic shock. The CentriMag can be used in patients with acute cardiogenic shock of any etiology, but its initial reported use was frequently in postcardiotomy patients because a median sternotomy is required for placement.23,24 No specific cannulas are required, so cardiopulmonary bypass cannulas can easily be used with this pump, which makes it relatively quick and easy to place a patient on support in situations where rapid deterioration occurred following attempts to wean from cardiopulmonary bypass.25,26 A typical placement of cannulas would be inflow from the right atrium with return to the pulmonary artery for an RVAD and left atrial inflow with return to the ascending aorta for an LVAD.23,24,27 The unique feature of this device is an impeller that floats and rotates in a magnetic field, so there is no mechanical contact with any other part of the pump (see Figure 15-6).
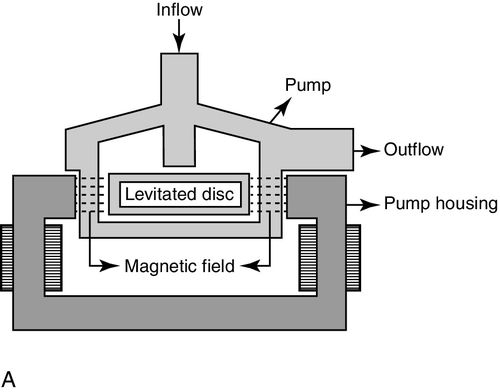
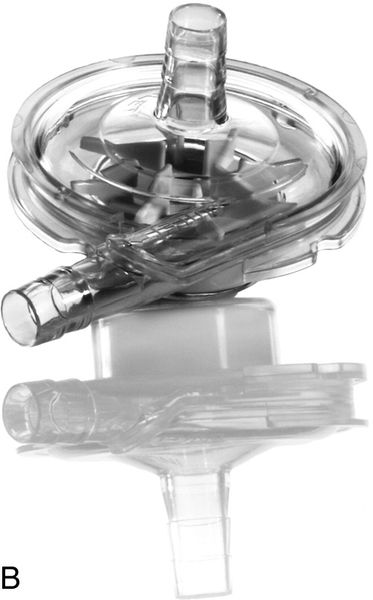
Put simply, the magnetic field is created by the electrical energy powering the pump. The impeller is uniformly washed by blood flow to minimize areas of blood stagnation and turbulence that can be sources of thrombus formation.28 This feature also minimizes hemolysis. Unlike the TandemHeart, patients with CentriMag can become mobile once their condition has stabilized because of the central cannulation. The pump battery system can provide up to 120 minutes of untethered support at a speed of 3500 RPM. The CentriMag device has been used for periods of up to 30 days to support patients as a bridge to decision, bridge to recovery, or bridge to transplantation.23,24,28 It can be used as an LVAD, RVAD, or biventricular assist device (BI-VAD). If needed, the CentriMag pump can be combined with an oxygenator to deliver what is traditionally thought of as ECMO support.26 The device is capable of providing flows of up to 10 L/min. Usual pump flows are between 4 and 5 L/min at speeds of 3000 to 4000 RPM.
Hemodynamic and Physiologic Effects of CentriMag Therapy
The hemodynamic and physiologic effects of the CentriMag device are similar to what one expects to see with the TandemHeart. Those are pressure and volume unloading of the left ventricle and, in the case of the CentriMag, pressure and volume unloading of the right ventricle if used as an RVAD or BI-VAD. The resultant decrease in myocardial oxygen demand will support the heart and allow time for potential recovery or for a bridge to re-evaluating the plan of care for the patient. Early initiation of support should have the effect of minimizing the damage to end-organ function and enhance organ recovery from cardiogenic shock.
Contraindications to Use of the CentriMag Device
The primary contraindication to the use of this device is any contraindication to anticoagulation. This contraindication would primarily apply to the evaluation of medical patients because surgical patients have already been screened and anticoagulated for cardiopulmonary bypass. If heparin-induced thrombocytopenia (HIT) should occur, patients can be converted to a direct thrombin inhibitor such as argatroban.
Potential Complications of CentriMag Therapy
As would be expected with a surgically implanted device, bleeding is the most commonly reported complication of device placement. The incidence of bleeding has been reported to be between 21% and 44%.23,24,28 The presence of cardiac tamponade should always be investigated when low flows occur in the presence of optimized volume loading. Thrombus may be less likely to occur within the pump but can develop in the atria, in the ventricle, or at the cannula connector sites, so a stroke as a result of thromboembolism is still possible.24 Low flows will increase the risk of thrombus. Low flows should also prompt an evaluation of right heart function in a patient with an LVAD. Right heart failure is a common source of poor LV filling and low preload for the pump. Hemolysis incidence has been reported to be low (5%) and not clinically relevant.23,28 When hemolysis does occur, it is likely related to the speed of the pump. The pump should not be run at higher speeds than necessary to achieve adequate flows. For example, flows of greater than 5 L/min may not be necessary and may only serve to increase the risk of hemolysis.
Impaired Hemodynamic Effectiveness and Limitations of CentriMag Therapy
The common theme for all devices is maintaining adequate pump filling, decreasing resistance to pump flow by active management of any systemic or pulmonary hypertension, and inotropic support of right heart function as required in patients with an LVAD. If these issues are not addressed, pump outflow can be affected, and this will impair effectiveness. A disadvantage of the CentriMag device is the requirement of a median sternotomy for cannula placement often in a hemodynamically unstable patient. Placement using a mini-thoracotomy has been reported and may be preferred in patients who have had a previous median sternotomy because of the greater risk of bleeding and the need for more complicated surgery.29
Peripheral Extracorporeal Membrane Oxygenation System
ECMO has been successfully used to treat refractory cardiogenic shock and failure to wean patients from cardiopulmonary bypass. Survival rates have been reported to be over 60% for non-postcardiotomy patients and 45% for postcardiotomy patients.30,31 The survival rate of over 60% in medical patients is similar to that achieved for acute respiratory failure indications. Acute respiratory failure is a longstanding indication for the use of this technology.32 Survival is about half that in patients resuscitated from cardiac arrest.33
The advent of centrifugal pumps has made the idea of ECMO following cardiopulmonary bypass more appealing. It is actually an extension of cardiopulmonary bypass with or without oxygenation support. Centrifugal pumps cause much less trauma to blood than the traditional roller pumps associated with cardiopulmonary bypass and can provide support to patients for days. Advantages of ECMO are that it can provide both cardiac and pulmonary support and it can be inserted percutaneously using the femoral artery and vein. Insertion is simple and rapid and can be achieved during cardiopulmonary resuscitation. The speed of application is the major appeal, as ECMO can be accomplished at the bedside.31 It is also one of the least costly forms of MCS therapy. As mentioned, the system consists of venous-to-arterial cannulation for the support of cardiac failure. In case of failure to wean from cardiopulmonary bypass, the previously opened chest allows for direct right atrial cannulation with return to the ascending aorta. In this application, the chest is left open until the cannulas are removed. Many companies have centrifugal pumps that can be set up with membrane oxygenators. Figure 15-7 is a schematic illustration of a venous-to-arterial central and peripheral ECMO circuit.
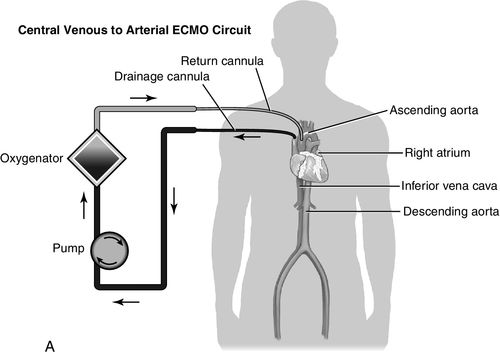
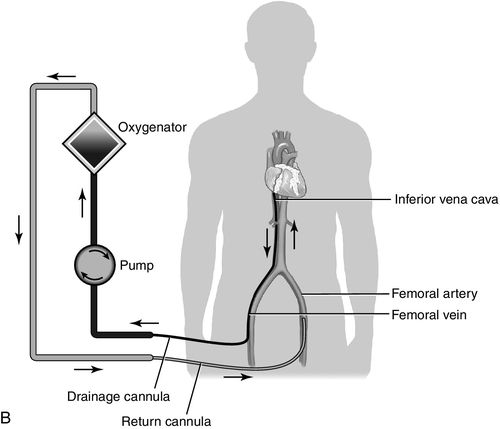
Indications and Contraindications of Extracorporeal Membrane Oxygenation Therapy
Virtually all situations where ECMO is considered are emergencies where time is of the essence. The patient may be too unstable for complex device insertion or too unstable to move to the cardiac catheterization laboratory or operating room. Indications include cardiogenic shock refractory to inotropic or IABP therapy, cardiac arrest, cardiogenic shock after cardiothoracic surgery with failure to wean from cardiopulmonary bypass, and post–heart transplantation graft failure. Patients may also be bridged to decision when the transplantation status is unknown, for example, following cardiac arrest when the patient’s neurologic status and comorbidities are unknown. For the majority of cardiothoracic surgery patients, the goal is bridge to recovery. Unfortunately, the duration of ECMO support is limited to usually no more than 7 to 9 days because of bleeding complications.30,31 In many areas of the United States, finding a heart donor in this short period is an unrealistic expectation, so, in this case, a longer-term device should be considered as a continued bridge-to-heart transplantation.
Absolute contraindications to ECMO include known neurologic dysfunction (e.g., dementia), pre-existing kidney failure, pre-existing liver failure, and significant aortic valve insufficiency. Prolonged shock with acidosis, oliguria, and prolonged hypotension will often be associated with poor outcomes, but consideration will be given to patient age and the degree to which kidney and liver functions have been affected. Relative contraindications include compromised pre-existing functional status and lack of social support if transplantation were to be considered an option. Advanced age is also a risk factor for poor outcomes.34 Given the emergent situation, it is left to individual physicians to make the best decisions about a patient’s likelihood for survival, as data to predict outcomes are limited.
Potential Complications of Extracorporeal Membrane Oxygenation Therapy
Bleeding is the most common complication with virtually all patients requiring transfusion after placement.30,31 Initial activated clotting time guidelines are initially targeted for 210 to 230 seconds, which might have to be reduced in a patient who is bleeding. Any bleeding is further complicated by the requirement for systemic anticoagulation to prevent the circuit from clotting off. Cerebral bleeding resulting in brain death is another devastating complication. Cardiac tamponade may be seen in postcardiotomy patients. Multi-organ failure occurs in approximately one third of patients.31 Other complications include sepsis, leg ischemia, pulmonary complications, and thrombus formation in the ECMO circuit. Large or mobile clots require an immediate change out of the pump circuit. It is important to have nurses or other experts who will continually monitor for the presence of clots and are capable of changing out the circuit. The greatest risk occurs when the activated clotting time is reduced in the presence of bleeding. Use of heparin carries a risk of heparin induced thrombocytopenia (HIT, which will require switching the patient to a direct thrombin inhibitor such as argatroban.
Impaired Hemodynamic Effectiveness and Limitations of Extracorporeal Membrane Oxygenation Therapy
Hemodynamic effectiveness of ECMO therapy will primarily be limited by problems with the pump circuit, for example, clotting and limitations of cannula size. Another consideration is not allowing blood returning from the bronchial circulation to distend the left ventricle. Afterload reduction for the left ventricle—through concomitant IABP therapy and optimal coronary perfusion—helps minimize this. Volume optimization will help with maintenance of optimal circuit flows, thus minimizing the risk of clotting. The ECMO circuit continuously monitors hemoglobin and hematocrit. Continuous monitoring of venous oxygen saturation (SvO2) is used to assess the adequacy of tissue perfusion. SvO2 assessment also serves as a marker for weaning tolerance from ECMO. Other monitoring indicators include esophageal echocardiographic determination of ejection fraction and right heart contractile performance.
Core temperature is monitored continuously. The long length of the cannulas, from the patient to the bedside ECMO pump, increases the risk of heat loss through the cannulas. Some circuits are capable of either warming or cooling. After cardiac arrest, patients are actively cooled to 32° C to 34° C for periods of up to 36 hours. Otherwise the patients are maintained at normothermia.31Figure 15-8 shows the ECMO monitor screen, where flow, pump RPM, temperature, and other parameters are continuously displayed.
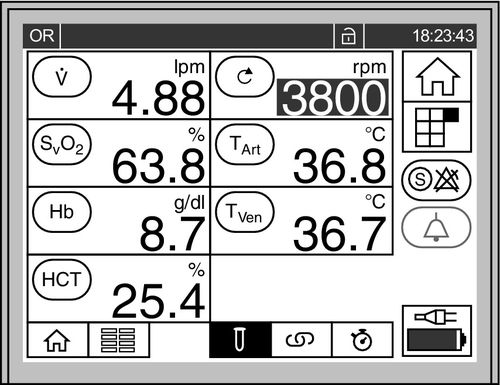
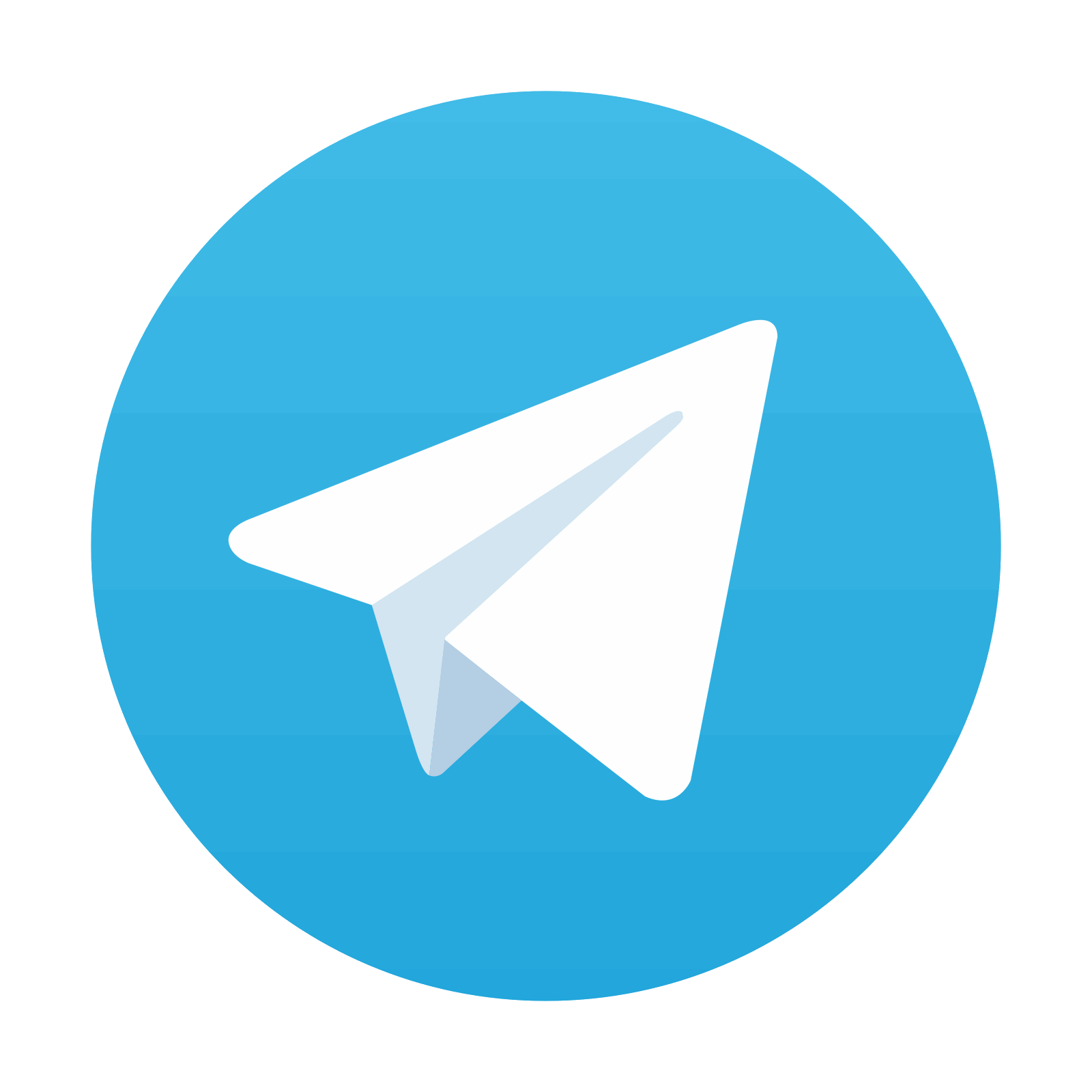
Stay updated, free articles. Join our Telegram channel

Full access? Get Clinical Tree
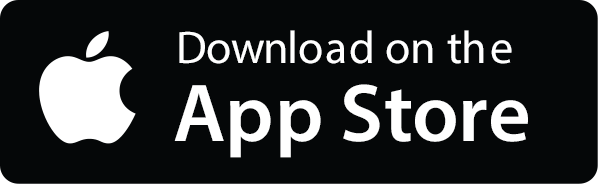
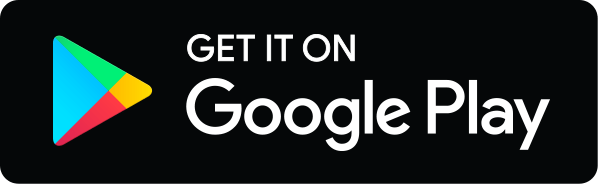