The RV architecture includes the intraventricular septum. The septum divides the two ventricles of the heart and is essential in the motion of ventricular contraction. Both the RV free wall and the intraventricular septum are equally important for normal function of the right ventricle.2
Right Ventricular Blood Supply
The RV free wall receives its blood supply from the right coronary artery (RCA). Uniquely, the proximal RCA is perfused in both systole and diastole. Two different arteries supply the septum: (1) the left anterior descending (LAD) artery, which provides blood flow to the anterior two thirds; and (2) the posterior descending artery (PDA), which supplies the inferior–posterior third.2
Pericardium
Anatomically, the pericardium plays an important role in the function of the right ventricle. The pericardial sac surrounds the complete heart and restricts excessive ballooning of the compliant right ventricle.
Ventricular Interdependence
The term ventricular interdependence describes the situation wherein the size, shape, and compliance of one ventricle may affect the size, shape, and pressure–volume relationship of the other ventricle through mechanical interaction.6 Ventricular contractile synchrony ensures that a change in one ventricle will be reflected by a change in the other.8 The constraining effect of the pericardium limits the size of each ventricle and augments the impact of interdependence.
Right Ventricular Volume Overload
The right ventricle, as a high capacitance chamber, can tolerate acute volume overload better than it can tolerate acute pressure overload. Conditions leading to RV volume overload include tricuspid regurgitation and atrial septal defect with a left-to-right shunt. In right heart failure, as the right ventricle progressively dilates, the ventricular cavity changes from the normal crescent shape to an oblong or more rounded shape. This geometric change impacts both the left ventricle and the pericardial sac via ventricular interdependence, as demonstrated in Figure 18-2.
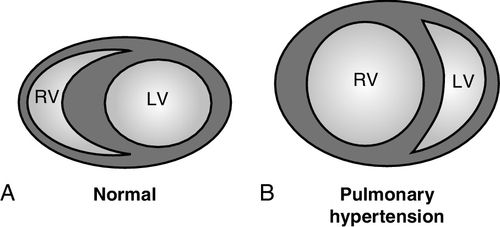
The intraventricular septum bows into the left ventricle as increased RV pressure or volume forces the RV chamber to dilate. This change in RV chamber shape, and increase in RV pressure, affects the ability of the left ventricle to fill and eject. The progressive RV dilation also increases pressure against the constraining effects of the pericardial sac. With these geometric imbalances, the left ventricle is challenged to accommodate a normal preload volume and generate a normal stroke volume.6,8 The result is a decreased left ventricular (LV) stroke volume and decreased cardiac output.
Right Ventricular Dilation
RV dilation results in distortion of the tricuspid annulus, causing tricuspid regurgitation. Tricuspid regurgitation increases RV preload and decreases cardiac output. Thinning of the walls as occurs in myocardial infarction, leads to higher local wall tension and increased risk of further RV free wall dysfunction.9
Right Ventricular Pressure Overload
Pressure overload on the ventricular myocytes, as seen with pulmonary valve stenosis, pulmonary embolism, pulmonary hypertension, and left heart failure, leads to cellular replacement by connective tissue. Pressure overload decreases RV contractility and decreases forward movement of blood into the pulmonary vascular bed. With progressive hypertrophy, RV function continues to deteriorate. Ejection becomes less complete, and wall tension remains elevated throughout the cardiac cycle. Coronary arterial perfusion is impaired and contributes to RV ischemia.10 The patient demonstrates increasing symptomatology and decreasing functional reserve.
Acute Pulmonary Embolism
Massive pulmonary embolism may lead to acute right heart failure and is considered the most frequent cause of acute RV pressure overload.11 Major emboli are usually bilateral, widespread, and involve large territories of the pulmonary vasculature.12 With pulmonary embolism, the sudden increase in afterload pressure for the right ventricle leads to initial myocardial hypertrophy and contractile dysfunction. This obstructive pressure is further increased with the release of pulmonary vasoconstrictors and hypoxemia.12 The RV chamber dilates to maintain stroke volume with rising filling pressures. This leads to diastolic dysfunction, decreased cardiac output, and tricuspid regurgitation.2 Other signs of acute pulmonary embolism include hypotension, as described in the case study at the end of Chapter 3, and a decrease in the partial pressure of end tidal carbon dioxide (PetCO2) as described in Chapter 8 (see Figure 8-7).
Right Ventricular Hypertrophy
Hypertrophy of the right ventricle occurs in response to increased resistance to ejection. The law of Laplace is used to describe the relationship of the tension (T) in the walls of a container with its radius (R) and the pressure (P) of its contents.9 Tension is proportional to the product: Pressure × Radius as described in Chapter 1 (Table 1-2). The thickening of ventricular walls tends to reduce the tension. Injury or conditions that generate increased pulmonary vascular resistance force the right ventricle to generate higher pressures to eject blood into the pulmonary vasculature. Hypertrophy ultimately limits RV diastolic filling ability and restricts cardiac output.8 Initially, this lowers wall tension. However, as RV failure progresses, ventricular diameter increases, causing increased stress on the RV wall.
Neurohormonal reactions to the decreased flow include activation of the sympathetic system that increases both heart rate and contractility. Continued decrease in cardiac output activates the renin-angiotensin-aldosterone system (RAAS) resulting in sodium and fluid retention.13 RAAS activation leads to a vicious cycle of volume overload and increased work for the failing right ventricle (see Figure 1-17 in Chapter 1).
Right Ventricular Infarction
Infarction of the right ventricular impacts its contractility. Right ventricular myocardial infarction (RVMI) is identified with increasing frequency with reports of RV involvement in 40% to 50% of acute myocardial infarctions.14 Classic signs of RVMI include hypotension, with elevated jugular venous pressure, and clear lung fields.13 Studies identify increased risk of mortality, cardiogenic shock, malignant ventricular arrhythmias, and atrioventricular block in the patient with an acute RVMI. Occlusion of the RCA proximal to the marginal branches leads to hypokinesis in the lateral and inferior right ventricle, whereas ischemia of the left anterior descending artery (LAD) usually results in anterior RV wall hypokinesis.1 The right ventricle has been known to exhibit spontaneous recovery with aggressive support. This recovery post ischemic injury is theorized to be secondary to the thinner muscular wall and dual arterial perfusion.6
Right Ventricular Failure
RV function is dependent on the classic factors that govern cardiac output: preload or the amount of volume in the right ventricle at the end of diastole; afterload or the resistance the right ventricle is ejecting against, contractility, and heart rate.15 Failure of the right ventricle occurs as a result of structural or functional disorders that alter the ventricle’s ability to fill or eject blood.6,10 The causes of RV failure are categorized under three areas that affect right ventricular function: preload, afterload, and contractility, and listed in Table 18-1.
Table 18-1
Causes of Right Heart Failure
Physiologic Impact on Right Ventricle | Clinical Condition |
Increased preload | Atrial septal defect Acute tricuspid regurgitation Volume overload |
Decreased contractility | Myocardial infarction Myocarditis Cardiomyopathy Congenital heart disease (some conditions impact preload and afterload) |
Increased afterload | Pulmonary hypertension Pulmonary embolism Interstitial lung disease Acute respiratory distress syndrome Left heart failure Acute mitral valve regurgitation Ventricular septal defect or rupture |
Most or all of these conditions result in increased volume or pressure in the right ventricle. Initially, the right ventricle tolerates increased pressure or volume with increased myocardial stretch and increased contractility. When these adaptive mechanisms are overwhelmed, the ventricle compensates in one of two ways: (1) by hypertrophy in response to increased pressure, and (2) by dilation in response to increased volume. Either of these conditions decreases ventricular function, as described earlier.
Acute decompensation, in which normal mechanisms are overwhelmed, occurs with an increase in cardiac demand such as sepsis or with an increase in afterload, as seen with arrhythmias, pulmonary embolism, or pulmonary hypertension.15
Right Ventricular Dysfunction in Left Heart Failure
Many studies on heart failure focus on impairment of the left ventricle as the main pump of the body.16 Chapter 17 discusses heart failure that alters left ventricular function in detail. In left heart failure, right heart work increases from afterload transmitted in a retrograde fashion through the pulmonary venous system with an increased transpulmonary gradient. Ischemia generated injury may affect both ventricles, depending on the culprit lesion, as the right heart is perfused by both RCA and LAD vessels. Left heart failure results in decreased cardiac output with concomitant decreased perfusion of the RCA. The RCA fills during systole and diastole and is sensitive to any fall in cardiac output. This impairment of RCA perfusion is compounded by the restricted pericardial compartment.2 The other component of left heart failure that impacts the right heart is related to the dilation of the LV chamber. This causes compression of the right ventricle in the limited pericardial space and results in decreased RV filling.
End-Stage Right Ventricular Failure and Pulmonary Hypertension
Pulmonary hypertension generates increased afterload in the right ventricle as explained in the next part of this chapter. The increased resistance causes RV hypertrophy, which results in decreased RV filling. This results in decreased RV stroke volume that results in decreased LV filling with concomitant hypotension and reduced cardiac output.
Diagnosis
Right heart failure was historically difficult to evaluate given the right ventricle’s geometry, interrelationship with the left ventricle, and sensitivity to alterations in pulmonary pressure.1 Right heart failure is diagnosed on the basis of signs and symptoms, laboratory tests, imaging techniques, and right heart catheterization, as described below.
Signs and Symptoms
Signs of right heart failure include fluid retention, peripheral edema, ascites, anasarca, exercise intolerance, fatigue, dyspnea, and atrial and ventricular arrhythmias.11 As RV failure worsens, the liver becomes engorged with a positive hepatojugular reflex. If severe, the patient may have discomfort or pain in right upper quadrant or epigastrium with a pulsatile enlarged liver.17 Auscultation may reveal an RV S3 gallop, and the murmur of tricuspid regurgitation. A parasternal RV lift may be observed. An RV “heave” may be felt when a clinician places the palm of the hand on the patient’s chest, over the area of the right ventricle. When present, this signifies RV hypertrophy.
Advanced right heart failure results in hypotension resulting from elevated filling pressures and low cardiac output. As the systolic reserve or the ability to maintain adequate forward flow with ejection decreases, patient symptoms progression can be tracked using the New York Heart Association (NYHA) functional classification system, as described in Chapter 17 (see Table 17-3).
Laboratory Tests for Right Heart Failure
Laboratory tests used in the diagnosis of heart failure are similar for both left-sided and right-sided heart failure. B-type natriuretic peptide, abbreviated as BNP, is a useful diagnostic test as levels increase as heart failure-related volume overload progresses. Natriuretic peptides correlate with right atrial and RV volume increase, and excessive stretching of the cardiac muscle. Serial measurements may help with diagnosis and treatment evaluation as discussed in Chapter 17 (see Table 17-7). Other laboratory abnormalities in right heart failure include hematology, chemistry, and anticoagulation measures.
With increased antidiuretic hormone (ADH) release, fluid retention and hyponatremia occur.17 Sodium levels less than 130 milliequivalents per liter (mEq/L) indicate significant fluid retention, which causes some patients to present with a decreased level of consciousness. Potassium is also an important electrolyte to monitor in right heart failure.
Creatinine levels, which are used to monitor for kidney insult, may be misinterpreted in this population. Although most would consider increased creatinine levels a sign of progressive kidney impairment, in right heart failure, it may be related to venous congestion rather than decreased perfusion from impaired cardiac output.10 Decrease in central venous pressure (CVP) may improve renal artery perfusion and clear the rising creatinine. Other dilutional effects include decreased hemoglobin and hematocrit levels.
With significant hepatic engorgement, impaired liver function may be detected from elevated liver biomarkers; impaired coagulation function is detected from elevated prothrombin time, international normalized ratio (INR), and activated partial thromboplastin time (aPTT).
Intracardiac Pressure Measurements
An estimation of CVP can be obtained from inspection of the internal jugular veins as described in Chapter 2 (Figure 2-3). This technique may be challenging and difficult to achieve in obese patients or with the presence of fluid or pressure lines in these vessels.10 Direct measurement of CVP or right atrial pressure (RAP) with invasive pressure lines assist with baseline and ongoing measurement of treatment effectiveness (see Chapter 4 for more details about CVP monitoring).
As mentioned later in this chapter, diagnostic right heart catheterization in the cardiac catheterization laboratory is considered the gold standard for the diagnosis of pulmonary hypertension. In the critical care unit, continuous monitoring with a pulmonary artery catheter (PAC) measures changes in pulmonary pressures in response to treatments. PAC measurements include pulmonary artery occlusion pressures (PAOP) or ‘wedge’ pressure, as an indirect measure of the LV end-diastolic pressure. However, whenever possible, noninvasive assessment of RV function is recommended.
Right Ventricular Diagnostic Tests
Chest Radiography
In the right heart failure picture, chest radiography may reflect an enlarged heart with increased major vessels. Because the right heart is positioned mostly behind the sternum, the enlargement may only be seen as LV displacement.18
Echocardiography and Doppler Imaging
In clinical practice, echocardiography is the most frequent evaluation method of the right ventricle. Transthoracic echocardiography is challenging because the retrosternal position of the right ventricle limits direct views.6 The increasing use of more advanced echocardiography modalities has helped improve recognition and evaluation of right ventricular function and failure.
Vena Cava Collapsibility
Because inferior vena cava collapsibility is a surrogate measure for filling volumes, findings related to inferior vena cava collapsibility may assist with assessment and treatment. The mechanics of breathing significantly influence superior vena cava flow, hepatic vein flow, and RV inflow velocities. Inspiration increases venous return, whereas exhalation decreases venous flow by up to 20%. With normal breathing, an inferior vena cava decrease in size greater than 50% suggests the RAP is less than 10 millimeters of mercury (mm Hg).1 A small inferior vena cava with spontaneous collapse indicates intravenous volume depletion, whereas no collapse suggests that the RAP is greater than 15 mm Hg.1 See Chapter 11 for a more detailed discussion on the use of bedside ultrasound.
2D Doppler Imaging
Two-dimensional transthoracic echocardiography (TTE) is a noninvasive measure that is easy to perform at the bedside. Advances in portable devices, including the newer hand-held models, permit rapid assessment of ventricular fill and function.
Echocardiography and Doppler imaging has evolved with combination methods and use of computer models that can recreate the geometric complexities of the right ventricle. These various modalities can all be performed from a single echocardiography assessment and are described below.
Tissue Doppler Imaging
Tissue Doppler imaging is a quantitative assessment of RV systolic and diastolic function, which measures velocities of flow across the atrioventricular (mitral and tricuspid) valves. This measure helps detect low velocity and high amplitudes, determine systolic and diastolic time intervals, and estimate cardiac output.1 Velocity is the speed of flow into each chamber over time and is used to estimate the cardiac output. A low systolic annular velocity through the pulmonic valve may predict right ventricular dysfunction.
Speckle Tracking Imaging
Speckle tracking imaging is an update of two-dimensional echocardiography, which uses a pattern-matching technology to combine ultrasound and cine data from cardiac catheterization. D’Andrea et al. found that this approach could quantify regional myocardial function and predict response to cardiac resynchronization using biventricular pacing.19
Three-Dimensional Echocardiography
Three-dimensional (3D) echocardiography with analysis eliminates reliance on geometric modeling.1 This method uses both short-axis and long-axis views to calculate RV volumes and ejection fraction.1
Transesophageal Echocardiography and Doppler
Transesophageal echocardiography (TEE), with the probe inserted in the esophagus, measures the size, function, contractility, and dilation of the right ventricle, as well as the flow through the valves.
Transesophageal Doppler probes calculate cardiac output by measuring stoke volume in the descending aorta. Esophageal probes are typically referred to as minimally invasive. See Chapter 10 for a more detailed discussion on the use of transesophageal Doppler.
Hemodynamic Values Derived from Echocardiography and Doppler
Unique hemodynamic measures are derived from the echocardiographic and Doppler modalities described above; these help with comprehensive RV evaluation, as described below:
Table 18-2
Hemodynamic Equations used in the Diagnosis of Pulmonary Hypertension
CHD, Congenital heart disease; ET, ejection time; IVCT, isovolumetric contraction time; IVRT, isovolumetric relaxation time; MI, myocardial infarction; MPI, myocardial performance index or tricuspid systolic excursion index; PVR, pulmonary vascular resistance; RHC, right heart catheterization; RVEF, right ventricular ejection fraction; RVFAC, right ventricular fractional area change; TAPSE, tricuspid annular plane excursion; TPG, transpulmonary gradient.
Cardiac Magnetic Resonance Imaging
Cardiac magnetic resonance imaging (cMRI) is being used more often to measure dimensions and function of the right ventricle. cMRI evaluates blood flow, stroke volume, cardiac output, and dimensions of the right ventricle noninvasively.
Exercise Testing
Cardiopulmonary exercise testing is useful in assessing RV function as maximum oxygen consumption (VO2 max) correlates with prognosis and quality of life. Exercise tolerance, which can be measured using the 6-minute walk distance (6MWD), is a simple measure to test exercise ability. The 6MWD test is easy to repeat over time to evaluate progression of right heart failure. With implementation of treatment, repeated measures indicate ventricular function progression or deterioration.
A functional class system for the right heart has been developed. Haddad et al. (2008) created a four-level continuum of right heart failure, with stages A and B denoting risk for chronic failure, and stages C and D demonstrating chronic right heart failure. Structural changes and increasing needs for management progress along this continuum.11
Treatment of Right Heart Failure
Goals of treatment for right heart failure are focused on optimizing preload, decreasing afterload, improving contractility, and stabilizing heart rates.
Optimizing Right Ventricular Preload
Decreasing volume and resistance to pumping are the key concepts in right heart failure management. Increased preload may be the initiating problem and is often the first focus of treatment. The aim of treating right heart failure is to optimize preload rather than initiate aggressive diuresis.7 Aggressive diuresis may result in hypovolemia and generate a vicious cycle of neurohormonal stimulation that results in further fluid and sodium retention. Removing excess volume load will decrease RV preload and pericardial constraint, optimize LV preload, and improve cardiac output. Progressive diuresis with doses of diuretics matched to a goal rate of diuresis is used for patients with marked volume overload. The goal is to generate diuresis while avoiding hypotension that may lead to RV ischemia. If this approach is not successful and the patient progresses to acute RV failure, more advanced fluid removal efforts include ultrafiltration and dialysis.7 For the patient with RV ischemia and hypotension, acute volume loading is started with a slow initial challenge of 500 milliliters (mL). If this careful fluid bolus does not improve blood pressure, further loading may escalate hemodynamic compromise.7 Continued judicious use of diuretics is designed to minimize fluid retention and limit preload. Other efforts include moderate sodium restriction (2 grams per day [g/day]) with daily weight monitoring to track progress of fluid removal over time.
Reducing Right Ventricular Afterload
Reducing afterload is the second goal in the treatment of right heart failure. Again, careful management of hemodynamics is essential to prevent worsening failure as a consequence of treatment. The two primary causes of increased afterload for the right ventricle are pulmonary hypertension and left heart failure, Understanding the interrelationship and interdependence of these systems is essential to understanding the hemodynamics of RV failure.20
Pulmonary vascular vasodilation is essential treatment to manage the pulmonary hypertension process. The three classes of medications used to treat pulmonary hypertension are (1) endothelin receptor antagonists, (2) phosphodiesterase inhibitors, and (3) prostanoids. These agents work to decrease the proliferation of endothelial cells and promote vasodilation of the pulmonary arteries.7 Oral and intravenous vasodilator medications are discussed in more detail later in this chapter.
Inhaled vasodilators: Inhaled nitric oxide may alleviate RV ischemia associated with cardiogenic shock. Inhaled nitric oxide has potent selective pulmonary vasodilatory effects that promote reduction in RV afterload. It is used following cardiac surgery in patients with acute right heart failure to treat exacerbations of pulmonary hypertension. The cost and serious side effects, including the risk of rebound pulmonary hypertension and methemoglobinemia, are limiting factors for routine use of inhaled nitric oxide.7 More recently, nebulized epoprostenol is being trialed to treat acute right heart failure exacerbations with results similar to using inhaled nitric oxide.21,22 Limitations of inhaled epoprostenol include side effects of hypotension, bradycardia, headache, or flushing.20 Dopamine or epinephrine may be considered in patients with severe hypotension and used with caution, as the increase of arrhythmic side effects of these inotropic medications may result in worsening RV failure.
Improving Right Ventricular Contractility
The third focus for treating right heart failure is improving contractility. In the acute hemodynamically compromised right ventricle, the use of a combination of inotropes, vasopressors, and vasodilators is beneficial. Dobutamine and milrinone are the agents of preference with right heart failure.1,10,15 Both dobutamine and milrinone have vasodilatory effects that promote decreasing afterload while improving contractility. Dobutamine improves myocontractility while decreasing both systemic vascular resistance (SVR) and pulmonary vascular resistance (PVR).7 Dobutamine is less likely to cause arrhythmias than dopamine. If the patient is already experiencing tachyarrhythmias, the inodilator milrinone, a phosphodiesterase III inhibitor, is preferred to improve RV contractility.20 Milrinone vasodilates pulmonary and systemic vessels and increases inotropy by increasing cyclic adenosine monophosphate and decreasing afterload.15 The combination effects of inotropy and vasodilation promote increased cardiac output and stroke volume while maintaining preload.
Heart Rhythm Management
The fourth aspect of treatment for right heart failure focuses on heart rhythm management. High-degree atrioventricular block or atrial fibrillation will have profound hemodynamic effects on right heart function. Loss of AV synchrony may significantly decrease cardiac output in patients with right heart failure because the RV preload is essential for function.20 Treatments include sequential atrioventricular pacing and cardioversion of unstable tachyarrhythmias.11 Biventricular pacing, also known as cardiac resynchronization therapy, may improve symptoms and survival.10
Implantable cardioverter defibrillators are considered for patients with acute RVMI who are at risk of sudden cardiac death. This includes patients who survived a cardiac arrest, have a history of sustained ventricular tachycardia, or who have demonstrated inducible ventricular tachycardia in the electrophysiology laboratory. Campbell et al. (2013) reviewed data from the MADIT-CRT trial and reported that treatment with cardiac resynchronization therapy improved RV function and generated a reduction in tricuspid regurgitation velocity.23
For patients with inducible monomorphic ventricular tachycardia, catheter ablation that interrupts the ventricular tachycardia circuit may be an effective treatment.
Preventing Complications
Other pharmacologic treatments are focused on prevention of complications associated with right heart failure including use of anticoagulation to prevent clot formation. Supplemental oxygen therapy is used to reverse hypoxemia and its side effect of vasoconstriction. In acute respiratory failure with right heart failure, ventilator support is challenging, as discussed in Chapter 14. It is important to avoid high levels of positive end-expiratory pressure (PEEP), maintain plateau pressures less than 30 mm Hg, and avoid acidosis and alveolar hypoxia. These actions will minimize the positive pressure effects of mechanical ventilation on intrathoracic pressures, which increase the workload of the right heart.
Surgical Procedures
Surgical procedures in right heart failure include correction of underlying causes such as tricuspid regurgitation, ventricular septal defect, or ventricular aneurysm repair.1 For end-stage right heart failure, a palliative measure of atrial septostomy may decompress the right ventricle by creating a right-to-left shunt.20 The atrial septostomy procedure (Figure 18-3) is performed in the interventional cardiac catheterization suite to decrease RV preload and decrease RV pressures.20 Decreasing preload improves symptoms of right heart failure. The major side effect is that deoxygenated blood passes into the left atrium, which increases systemic hypoxia.
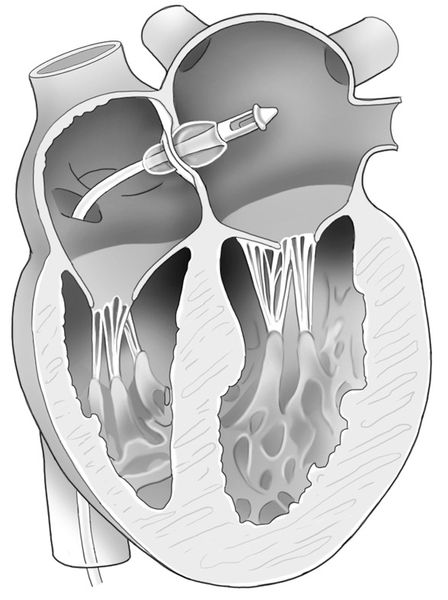
Right ventricular assist devices (RVAD) are used to treat right heart failure refractory to medical treatment. Mechanical circulatory assist devices may be used as a bridge to transplantation or as destination therapy. A number of devices can be inserted percutaneously for short-term use or implanted surgically.10 The hemodynamic effects of RVAD and other mechanical circulatory assist devices are discussed in detail in Chapter 15.
Outpatient Treatments
In the outpatient setting, medications to treat right heart failure are similar to those used to manage left heart failure. Beta-blockers and angiotensin-converting enzyme inhibitors or angiotensin receptor blockers are used to lower blood pressure and limit ventricular remodeling. Arrhythmia control will continue with the use of oral antiarrhythmic medications. Maintaining anticoagulation will be driven by the diagnosis and decisions of the patient and clinical management team. With recovery after the initial insult and as heart failure progresses, graded physical activity may improve functional capacity and quality of life.
It is important for patients with right heart failure to avoid isometric exercises, as these may be associated with syncope. Patient symptoms are what drive changes in patient management. Education for patients and caregivers include symptoms to report, ongoing weight management, moderate sodium restriction, medications, and regular office visits essential to follow-up. Verifying patients’ understanding of treatments and knowledge of whom to access for questions decreases hospital re-admissions and improves satisfaction.
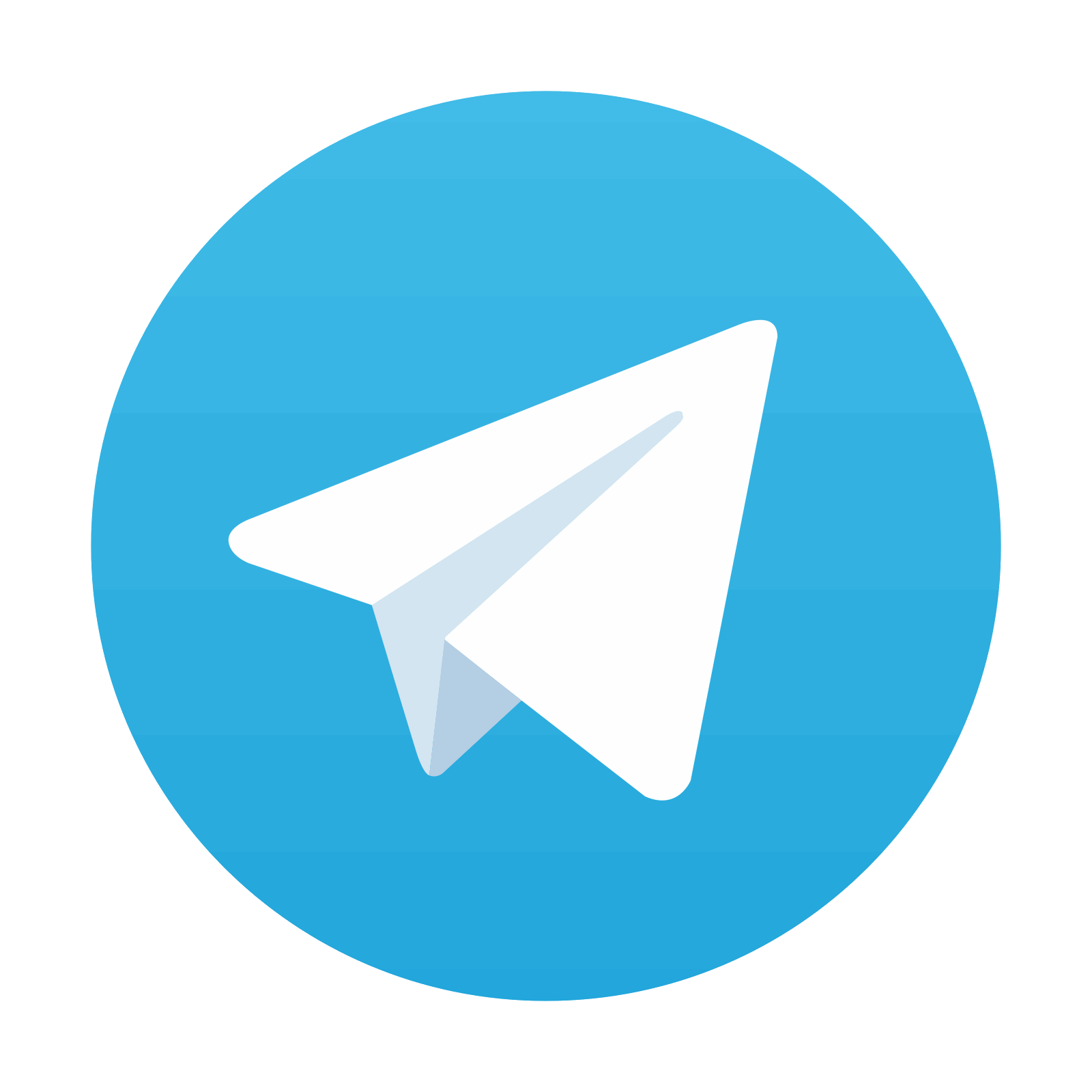
Stay updated, free articles. Join our Telegram channel

Full access? Get Clinical Tree
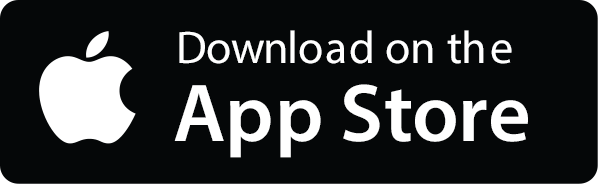
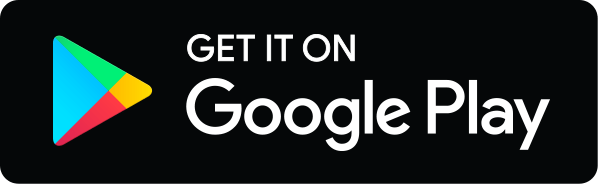