Data from Go AS, Mozaffarian D, Roger VL, et al.: Heart disease and stroke statistics—2014 update: a report from the American Heart Association, Circulation 129:e28–e292, 2013.
Table 17-2
Annual Cost of Heart Failure in the United States
Cardiovascular disease | Estimated direct and indirect costs in billions |
Coronary heart disease | $108.9 |
Hypertensive disease | $93.5 |
Stroke | $53.9 |
Heart failure | $34.4 |
Data from Heidenreich PA, Trogdon JG, Khavjou OA, et al.: Forecasting the future of cardiovascular diseases in the United States: a policy statement from the American Heart Association, Circulation 123:933–944, 2011.
The continuum of heart failure has both acute and chronic stages. However, the literature now refers to acute heart failure as acute decompensated heart failure (ADHF), reflecting the progressive nature of the illness.5 Compensated heart failure describes the condition in a patient who has a heart failure diagnosis but whose symptoms are minimal because of effective pharmacologic and lifestyle management. ADHF represents the failure of those treatment interventions to limit symptoms and often requires hospitalization to optimize therapies and to regain control of the patient’s symptoms. At the far extreme of the continuum is cardiogenic shock, in which the heart’s ability to support circulation is so impaired that end organ compromise results if rapid and effective interventions are not initiated. Heart failure may impact the left or right ventricle and, not uncommonly, involves both. To understand more about right ventricular (RV) failure, refer to Chapter 18. The rest of this chapter will focus on left heart failure.
In the early stages of left heart failure, a series of compensatory mechanisms are activated to correct blood flow abnormalities. The Starling mechanism yields an increased stroke volume when the left ventricular end-diastolic volume increases because of incomplete emptying of the ventricle. The resulting distention of the ventricle results in stretching of the myocardial fibers and increased ability to contract, thus increasing stroke volume (Figure 17-1).
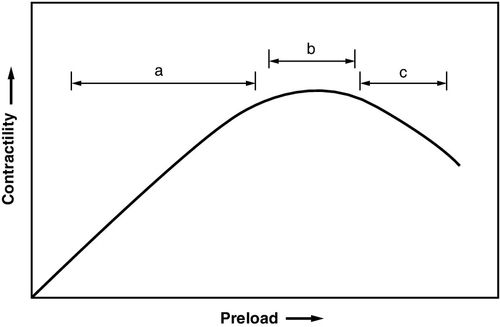
Neurohormonal responses are activated in heart failure to improve organ and tissue perfusion. The sympathetic nervous system responds to decreased flow with increased concentrations of norepinephrine in the bloodstream. This adrenergic chemical stimulates beta1-receptors, resulting in increases in both heart rate (chronotropy) and myocardial contractility (inotropy). Norepinephrine also stimulates alpha1-receptors in the myocardium increasing contractility. In the arterioles, norepinephrine increases vasoconstriction (afterload), raising blood pressure and shifting blood flow toward the vital organs. Over time, in response to reduced renal blood flow and other stimuli, the renin–angiotensin–aldosterone system (RAAS) converts angiotensinogen, through angiotensin I to angiotensin II, using several pathways. This powerful hormone also contributes to increased arterial vasoconstriction. At the same time, release of aldosterone by the adrenal gland stimulates the renal tubules to retain sodium and, with it, water, to preserve circulating volume (see Chapter 1, Figure 1-17). The third neurohormonal pathway, arginine vasopressin, stimulates a reduction in the excretion of water.6
Although initially effective, over time, these compensatory mechanisms may accelerate the rate of decline of cardiac function. The cardiac muscle becomes progressively weaker because of a combination of myocyte necrosis (myocardial infarction), myocyte apoptosis (programmed cell death), cytokines such as tumor necrosis factor alpha (TNF-α), and oxidative stress proteins.6 This reduced contractility inhibits the heart’s ability to increase stroke volume through the Starling mechanism. Similarly, contractile beta-receptors that were initially responsive to norepinephrine lose their sensitivity to the hormone. Vasoconstriction caused by sympathetic stimulation and the RAAS further limit the weakened ventricle from ejecting blood. The backup of blood from inadequate ejection along with continued volume retention stimulated by the RAAS and arginine vasopressin result in fluid volume overload, pulmonary edema, and systemic edema (Figure 17-2).
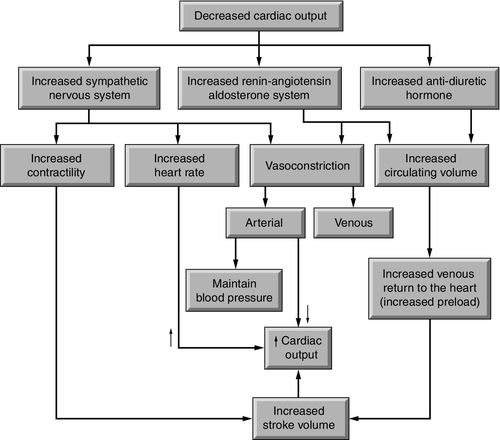
The myocardium, in response to continued elevation in afterload as a result of vasoconstriction or hypertension, undergoes hypertrophy. When persistently unable to propel enough blood to tissues, the ventricle dilates from the excess volume, and remodeling is complete. Reversal of this remodeling is difficult to achieve, and progression of remodeling accelerates mortality from heart failure. Symptoms often do not appear until after remodeling has begun.
Heart failure is categorized in several ways: (1) type of LV dysfunction: systolic or diastolic; (2) flow pattern: forward flow or backward flow; and (3) severity: heart failure or cardiogenic shock.
Subcategories of Heart Failure
Systolic versus Diastolic
To this point, the focus of the discussion has primarily been on systolic heart failure, the inability of the ventricle to eject blood to the tissues because of progressive weakness based on the pathophysiology described. Current literature also classifies this as heart failure with reduced ejection fraction (HFrEF). This type of heart failure is the most studied and involves a large percentage of those diagnosed with heart failure. Ejection fraction, calculated as (end-diastolic volume – end-systolic volume) ÷ end-diastolic volume of less than 35% to 40% is classified as heart failure. A normal ejection fraction is 55% to 65%.7
However, in recent years, recognition and interest in the role of diastolic dysfunction has grown. In diastolic heart failure, ejection fraction is 45% to 55%, but LV filling is slowed and stroke volume is reduced. Diastolic heart failure accounts for an increasing proportion of all cases of heart failure in the United States.8 Also referred to as heart failure with preserved ejection fraction (HFpEF).2 Causative mechanisms for this type of heart failure are postulated to include abnormal ventricular relaxation, difficulties with ventricular–vascular coupling, volume overload, chronotropic incompetence, or endothelial dysfunction.9 The prevalence of HFpEF has been reported to be growing over the last 15 years to now exceed 50% of newly diagnosed patients.10 Therapeutic options for HFpEF that alter the morbidity and mortality associated with it are limited.
Bursi and colleagues reported rates of diastolic HFpEF of 55% in their sample of 556 participants, associated with increased age and female gender.11 In this study, isolated diastolic dysfunction was present in 44% of the patients reviewed. Yancy and colleagues in their analysis of the ADHERE (Acute Decompensated Heart Failure National Registry) Database (n = 52,187) identified hypertension as a significant risk factor in those with HFpEF in contrast to patients without preserved ejection fraction. In addition, increased age and female gender were factors more likely to be associated with HFpEF (p < 0.0001).12
Forward Flow versus Backward Flow
Forward blood flow, measured as cardiac output, is impacted by its basic elements: heart rate and stroke volume (CO = HR × SV), as shown in Figure 1-7 in Chapter 1. Stroke volume may be altered by changes in preload (volume in the ventricle at the end of diastole), contractility (the force exerted against the ventricular contents to propel it out of the ventricle) and afterload (the resistance against which the heart has to pump the blood out of the ventricle and into the arterial circulation). It is important to distinguish this key concept of heart failure—inadequate forward flow to meet the metabolic demands of the tissues—from its most commonly associated symptom: fluid volume overload. As noted above, congestion is the result of inadequate emptying of the left ventricle and the compensatory mechanisms that are intended to correct the acute condition. This will be important to keep in mind during the discussion of interventions to improve cardiac output and reduce congestion.
Cardiogenic Shock
Cardiogenic shock, regardless of cause, represents the most severe form of LV failure. In this severe low cardiac output state, LV volume is elevated, cardiac output and blood pressure are low, and organs demonstrate signs of hypoperfusion: clouded mentation, low urine output, metabolic acidosis and cool extremities.13 Without rapid and effective intervention, mortality associated with cardiogenic shock has been reported as high as 80% and is associated with about 10% of acute myocardial infarctions.14
The key to effective treatment of heart failure lies in recognizing the signs and symptoms of the disease to facilitate diagnosis; characterizing the type and degree of LV failure by utilizing hemodynamic measurement and other assessment tools to guide therapy; and evaluating the interventions undertaken to determine their effectiveness in improving the patient’s condition. This chapter will consider each of those elements.
Signs and Symptoms of Acute Decompensated Heart Failure and Cardiogenic Shock
The symptoms most commonly associated with heart failure include shortness of breath, fatigue with resulting exercise intolerance, and fluid retention reflected in edema both in the pulmonary circuit as well as in the periphery. In 1928, the New York Heart Association (NYHA) developed a functional classification system that has been applied to patients with heart failure for more than 80 years. It has undergone modifications over time, and was most recently revised in 1994.15 Classification is based on functional measures (subjective) as well as objective indicators of cardiac dysfunction (Tables 17-3 and 17-4). However, not all patients experience all of these symptoms. Some may complain of exercise intolerance and dyspnea without evidence of fluid retention; others develop significant edema and yet have no complaints related to exercise or breathing. Some patients are noted to have a severely reduced ejection fraction (HFrEF, systolic heart failure) but are asymptomatic, whereas others with relatively preserved contractility (HFpEF, diastolic heart failure) are symptomatic at rest or with mild exercise. A clear explanation of the physiology of heart failure symptoms remains somewhat elusive.
Table 17-3
NYHA Functional Classification of Heart Failure
Source: http://www.heart.org/HEARTORG/Conditions/HeartFailure/AboutHeartFailure/Classes-of-Heart-Failure_UCM_306328_Article.jsp. Accessed September 1, 2014.
NYHA, New York Heart Association.
Table 17-4
Objective Classification of Heart Failure Symptoms
Source: http://www.heart.org/HEARTORG/Conditions/HeartFailure/AboutHeartFailure/Classes-of-Heart-Failure_UCM_306328_Article.jsp. Accessed September 1, 2014.
Physical Examination
As is the case with many health problems, patients with heart failure often present with a constellation of complaints that could represent a variety of disorders. As noted above, although many patients have similar complaints, heart failure may exist without all of them being present. In addition, by some estimates, as many as 40% of patients in decompensated heart failure may not demonstrate symptoms detectable purely by physical examination.
However, in those patients exhibiting physical signs and symptoms of heart failure, important insight into their disease and potential initial therapies can be derived. A combination of intentional inquiry about potential symptoms in the patient interview, along with a comprehensive physical examination can yield subtle—and not so subtle—changes in end organ function that reflect an alteration in cardiac output related to heart failure. See Table 17-5 for possible indicators of LV or RV function.
Table 17-5
Symptoms of Left and Right Ventricular Failure
Data from Soine LA: Heart failure and cardiogenic shock. In Woods SJ, Froelicher ESS, Motzer SU, Bridges EJ, editors: Cardiac nursing, ed 6, Philadelphia, 2010, Wolters Kluwer/Lippincott Williams & Wilkins, p 567.
During the first encounter, patients with ADHF often show evidence of orthopnea and an inability to lie flat, which may be a clue that the patient is experiencing heart failure with volume overload and secondary pulmonary congestion. Other reported symptoms consistent with orthopnea include paroxysmal nocturnal dyspnea, sleeping with multiple pillows or sleeping in a recliner.
Neurologic Signs and Symptoms
During the interview with the patient with ADHF, inadequate cerebral perfusion may be evidenced by somnolence, confusion, and slowness in response to questions as well as complaints of memory impairment. This may be an early subtle sign of deteriorating heart failure in the critical care environment, particularly in the patient being prematurely weaned, or weaned too aggressively from inotropic support. In addition, patients may complain of dizziness or weakness with sudden changes in position (orthostasis). Distinguishing between this and medication side effects is challenging, requiring clinicians to be closely attentive to both current conditions and the trajectory of neurologic functioning over time.
Heart and Vascular Signs and Symptoms
Heart rate and stroke volume are the variables that impact cardiac output. It is common in heart failure for heart rate to be elevated. This response, mitigated by the sympathetic nervous system, is intended to enhance cardiac output by affecting one of its two components (Cardiac Output = Heart Rate × Stroke Volume). This response may be blunted or negligible in patients who are receiving medications such as beta-blockers, which will limit their ability to significantly increase heart rate. Aging may also impair this chronotropic response to reduced blood flow. Patients may report palpitations or an irregular heartbeat. This may be the result of premature contractions of the atria or the ventricles or the development of atrial fibrillation. With those dysrhythmias, the loss of atrial contribution to ventricular filling is another important negative influence on forward flow.
Auscultation and palpation may reveal additional indicators of heart failure. An S3 murmur, reflecting LV distension and severe hemodynamic compromise, may be combined with an S4 murmur, common in diastolic dysfunction, to produce a gallop rhythm.8 Murmurs associated with either of the atrioventricular valves may provide additional evidence of valvular incompetence associated with volume excess and myocardial dilation disrupting the normal closure of the valve leaflets. Also consistent with cardiac enlargement is the shift of the point of maximal intensity to the left of the midclavicular line and downward toward the fifth intercostal space.8 Finally, a ventricular heave felt over the precordium provides additional evidence of ventricular enlargement.
Visualization and palpation of the vascular structures provides evidence of inadequate forward flow and volume congestion. Arterial pulses may be diminished. Pulsus alternans, which describes alternating strength of the palpated pulse, is associated with LV systolic dysfunction, and a beat-to-beat variation in ventricular preload.7 Jugular venous distension, measured with the patient lying at a 45-degree angle and estimated in centimeters of water (cm H2O), is evidence of the fluid volume excess common in decompensated heart failure.
Kidney Signs and Symptoms
Reduction in perfusion to the kidney stimulates a cascade of neurohormonal responses in the RAAS, which result in a reduction in urine output to control perceived loss of cardiac output. This reflects a decrease in throughput of blood through the nephrons based on both reduced cardiac output and venous congestion that obstructs renal venous outflow. The physiologic goal of this response is to preserve preload in an effort to improve cardiac performance. However, this compensatory mechanism, if left unchecked, may result in an accelerated decompensation of heart failure.
Pulmonary Signs and Symptoms
As forward flow is reduced, renal venous stasis increases, and fluid retention is stimulated by the RAAS, an increase in lung water is inevitable without intervention. LV congestion causes fluid to back up into the lungs. The elevation of hydrostatic pressure in the pulmonary circulation overwhelms the available oncotic pressure, preventing the recruitment of fluid back into the circulation, and instead shifting it into the alveoli. Patients may develop a dry, nonproductive cough and, on auscultation, have fine bibasilar crackles (rales) that can progress to rhonchi, wheezing, pleural friction rubs, and pulmonary edema, if left untreated. Further fluid pooling may lead to pleural effusion and a notable dullness on chest percussion.
Liver and Gastrointestinal Signs and Symptoms
Right-sided congestion may be associated with liver engorgement and ascites. The edge of the liver is palpable below the right costochondral margin in advanced heart failure, and percussion may reveal hepatomegaly.7
Integumentary Signs and Symptoms
The skin, as the body’s largest organ, will often reveal an early reduction in perfusion in heart failure as circulation is shunted to supply core organs. This reflects activation of the sympathetic nervous system and the RAAS, both of which stimulate vasoconstriction. Vasoconstriction will strive to raise central blood pressure and improve perfusion of the central organs. The result is cool, pale skin with reduced capillary refill, decreased peripheral pulses, and at times a mottled appearance. Because of significant vasoconstriction, peripheral pulses are often more difficult to detect manually and may require Doppler assessment. Pulse oximetry may be difficult to assess because of inadequate peripheral perfusion. In addition, the augmented afterload increases the workload of the already failing myocardium, again contributing to acceleration of the downward spiral of heart failure if no intervention is attempted.
Cardiogenic Shock
In cardiogenic shock, the severity of all signs and symptoms is exacerbated. The patient will be unconscious, delirious or irritable and may have a sense of doom. Profound hypotension with narrowed pulse pressure and tachycardia are present, along with pallor, cyanosis, diaphoresis, cool clammy skin, reduced capillary refill, and thready or absent peripheral pulses. Cardiac output may be worsened by dysrhythmias and heart block. Oliguria or anuria may develop quickly. Deteriorating respiratory function is characterized by tachypnea, dyspnea, and irregular breathing patterns. Ventilation–perfusion mismatch leads to hypoxemia. Rapid recognition of these symptoms and initiation of immediate therapies to increase blood flow and restore perfusion to vital organs is essential to increase the likelihood of survival from this critical illness.
Framework for Evaluating Heart Failure
Stevenson and Perloff provided valuable conceptual insights for understanding the four hemodynamic presentations of heart failure.16,17 Using a 2-by-2 graphic, the presence of the primary features of heart failure—low perfusion and increased venous congestion—were either present or absent17:
In 2002, Stevenson and associates expanded the grid to include the principal symptoms that may be seen on physical examination as discussed above17 (Figure 17-3). The symptoms of congestion were orthopnea, elevated jugular venous pressure, presence of S3 and a loud P2, edema, ascites, rales (crackles), and hepatojugular reflux. Signs of low peripheral perfusion included narrow pulse pressure (the difference between systolic blood pressure and diastolic blood pressure), pulsus alternans (alternating higher and lower systolic pressure),17 cold forearms and legs, decreased level of consciousness, angiotensin-converting enzyme inhibitor–related symptomatic hypotension, declining serum sodium level, and worsening kidney function.18 It is important to note that the vast majority of these signs of heart failure can be determined without a single invasive measurement.
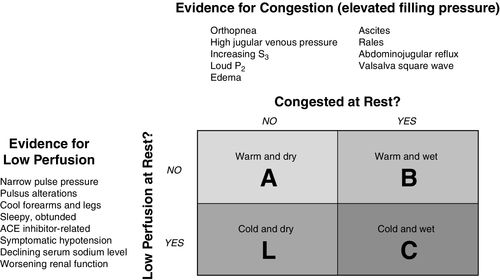
The advantage patients and clinicians have in today’s health care environment is the availability of noninvasive technologies that supplement the physical examination in determining the degree of dysfunction associated with a patient’s heart failure or cardiogenic shock.
Noninvasive Assessment
Beyond the physical examination, the assessment of blood pressure, cardiac rhythm, and pulse oximetry provide important additional data to better characterize the patient’s cardiovascular function. Critically thinking about what each of these tools provides—and does not provide—translates into data to include in the plan of care.
Cardiac Rate and Rhythm
In addition to the cardiac assessment, which can be performed by auscultatory or palpation methods, understanding the patient’s cardiac rhythm beyond rate and “regular or irregular” offers important insights into the possible reasons for reduction in cardiac output as well as possible interventions to improve it.
Flow is most effective when the heart rhythm begins in the sinus node, conducts as a single stimulus through the standard conduction pathways to the atrioventricular node, and then through the bundle branches to the Purkinje fibers. The associated sequential contraction, first of the atria and then of the ventricles, provides an opportunity for optimal filling of the ventricles prior to ejection.
Atrial fibrillation is frequently associated with dilated cardiomyopathy and myocardial infarction. The resultant loss of atrial “kick” reduces ventricular filling and may have a significant negative impact on cardiac output. Bradycardia, persistent supraventricular tachycardia, and ventricular tachycardia will also have a profound negative impact on forward flow. Tachycardia increases myocardial work and myocardial oxygen consumption while reducing coronary artery perfusion time and contributing further to myocardial dysfunction. Thus, monitoring the electrocardiogram (ECG) for signs of dysrhythmias as well as for evidence of myocardial ischemia and bundle branch block facilitates early intervention before permanent myocardial damage has occurred.21
Noninvasive Blood Pressure Monitoring
In the early stages of ADHF, systolic blood pressure may be elevated or normal. When heart failure is more advanced because of LV dysfunction, systolic blood pressure is often low. Diastolic blood pressure is frequently elevated owing to the persistent vasoconstriction associated with compensatory mechanisms for heart failure. Pulse pressure is low because of the combination of reduced systolic blood pressure and elevated diastolic pressure. This low pulse pressure is associated with reduced stroke volume.8 The degree of lowering of systolic blood pressure impacts end organs. Some patients are functional with systolic blood pressure between 80 and 90 millimeters of mercury (mm Hg), whereas others feel light-headed when their pressure is less than 100 mm Hg. Other evidence of the negative sequelae of hypotension may be detected from alterations in laboratory values or reduction in urine output.
Pulse Oximetry
Noninvasive assessment of pulse oximeter oxygen saturation (SpO2) helps complete the picture of heart failure. Reduced oxygenation may reflect an increase in lung water and associated reduction in gas exchange at the capillary–alveolar interface.
To be accurate, the pulse oximeter must be able to detect a pulse with enough variation to recognize arterial flow and thus appropriately assess the oxyhemoglobin level of the blood passing by. Narrowed pulse pressure, peripheral vasoconstriction, or anemia may reduce the accuracy of the SpO2 measurement and result in inaccurate data being considered in the treatment plan. Careful monitoring of the waveform displayed by the pulse oximeter is crucial to accurate data collection.
Echocardiography
Transthoracic two-dimensional echocardiography, including two-dimensional Doppler flow studies, has been characterized as the most useful diagnostic tool for evaluating ADHF. This single noninvasive test provides measurements of ventricular chamber size, myocardial wall thickness, valvular function, systolic and diastolic function, and pericardial effusion. Because of its noninvasive nature, rapid patient assessment using a transthoracic echocardiography quickly provides information to guide interventions to improve patient condition.
Echocardiography helps the clinician distinguish between systolic and diastolic heart failure, provides visual evidence of changes in ventricular wall motion and evidence of changes in atrial dimensions. Hemodynamically, patients with diastolic heart failure have elevated filling pressures and reduced cardiac output similar to those with systolic heart failure, but with little reduction in ejection fraction. Thus, the differential diagnosis of diastolic heart failure is made by noting abnormal ventricular filling pressures in the presence of preserved systolic function. In many patients with heart failure, features of systolic and diastolic dysfunction coexist.2
Chest Radiography
The 2012 recommendations of the Canadian Cardiovascular Society include obtaining a chest radiograph within the first 2 hours after admission for ADHF. The value of this noninvasive assessment is to assist the clinician in evaluating the lung fields as well as the cardiac silhouette as part of a complete evaluation of the patient with dyspnea.22
Invasive Hemodynamic Assessment
Intra-arterial Monitoring
Placement of an arterial catheter in ADHF and cardiogenic shock is usually to facilitate closer monitoring of blood pressure. As mentioned above, pulsus alternans may be an important indication as the ventricle’s variable filling, resulting in alternating higher and lower peak systolic pressure tracings on the arterial line display. In addition, narrowed pulse pressure will be reflected in reduced amplitude of the arterial waveform.
Most patients with ADHF requiring hospital admission are hypertensive (SBP > 140 mm Hg). An analysis of the ADHERE (Acute Decompensated Heart Failure National Registry) Database (n = 52,187) demonstrated that 44% of patients admitted to the hospital with reduced systolic function had hypertension; an even higher proportion (61%) of those admitted with ADHF with preserved systolic function were hypertensive. DBP was preserved in both groups.12
A cardinal finding in cardiogenic shock is hypotension and patients admitted to the critical care area with ADHF often will have a systolic blood pressure less than 90 mm Hg, whereas diastolic blood pressure may remain preserved (70–85 mm Hg), resulting in a narrowed pulse pressure (systolic blood pressure – diastolic blood pressure). In addition, the presence of an arterial catheter and continuous arterial pressure monitoring may provide important feedback about the efficacy of a new therapy. Changes in absolute blood pressure, mean arterial pressure (MAP), and pulse pressure may all be valuable in titrating therapy for the individual patient. Finally, access to arterial blood gas monitoring offers additional clinical information related to the patient’s acid–base balance, oxygenation, and ventilatory efficiency.
Intracardiac Pressure Monitoring
Hemodynamically, heart failure is characterized by a low flow state. Patients with compensated heart failure may have adapted reasonably well to this low flow state by increasing oxygen extraction from blood. However, in ADHF, the patient’s compensatory mechanisms have become overwhelmed as the cardiac output is insufficient to meet basic oxygen extraction and metabolic needs. Those patients are the ones most likely to be admitted to the critical care unit for stabilization and management of their heart failure symptoms, to reverse the progression of ventricular dysfunction and prolong survival.
Although no single parameter is essential for the diagnosis of ADHF, those most commonly cited include cardiac index below 2.2 liters per minute per square meter (L/min/m2); systolic blood pressure less than 100 mm Hg; pulmonary arterial occlusion pressure (PAOP) greater than 18 mm Hg; and right atrial pressure (RAP) or central venous pressure (CVP) greater than 15 mm Hg.
Congestion is not the cause of heart failure but rather the result of it. Utilizing the information gathered in the hemodynamic assessment, the clinician often can identify the affected ventricle(s) by recognizing which chamber (right ventricle, left ventricle, or both) is congested. This information will permit the clinician to choose the pharmacologic and mechanical therapies that will have the greatest likelihood of improving forward flow and reducing congestion.
Cardiogenic shock is the most extreme form of ADHF, in which tissues are suddenly deprived of blood because of an abrupt failure of the myocardium. Mortality rates of 50% to 100% are not uncommon in this form of shock related to end organ failure. Cardiogenic shock, like ADHF, is a syndrome whose hemodynamic parameters include hypotension defined as MAP < 60 mm Hg or systolic blood pressure < 90 mm Hg for more than 30 minutes, narrowed pulse pressure, cardiac index of less than 2.0 L/min/m2 and PAOP > 18 mm Hg. The severity of inadequate tissue perfusion is reflected in altered mental status, cool extremities, and low urine output. Disordered laboratory measures include elevated creatinine and serum lactate, metabolic acidosis, and abnormalities in liver tests and pulmonary function.
Clinical conditions that worsen cardiogenic shock may occur as a consequence of acute myocardial infarction. These include dysrhythmias, both tachycardia and bradycardia, and loss of atrial kick with atrial fibrillation. Other potential contributors include major surgery or trauma, and structural abnormalities such as ventricular septal rupture, acute disruption of a cardiac valve, or pulmonary embolism. Likewise, substances that depress myocardial function such as acidemia, drugs, and electrolyte abnormalities may worsen cardiogenic shock.
Cardiac Output Measurement
The standard thermodilution method for cardiac output measurement described in previous chapters and the derived values calculated using cardiac output and other measured hemodynamic values are important in assessing cardiac function in ADHF and cardiogenic shock. The transpulmonary gradient (TPG) and even ventricular stroke work index provide insight into the potential compensatory mechanisms that are causing functional deterioration (Table 17-6).
Table 17-6
Hemodynamics of Heart Failure and Cardiogenic Shock
Value (units) | Heart failure | Cardiogenic shock |
Blood Pressure (mm Hg) | ↑→↓ | ↓ |
CVP/RAP (mm Hg) | ↑→ | ↑ |
PAP (mm Hg) | ↑ | ↑ |
PAOP | ↑ | ↑ |
SV | ↓ | ↓ |
HR | ↑ | ↑ |
SVR | ↑ | ↑ |
PVR | ↑→ | ↑ |
RVSWI | ↓ | ↓ |
LVSWI | ↓ | ↓ |
CVP, Central venous pressure; HR, heart rate; LVSWI, left ventricular stroke work index; mm Hg, millimeters of mercury; PAOP, pulmonary artery occlusion pressure; PAP, pulmonary artery pressure; PVR, pulmonary vascular resistance; RAP, right atrial pressure; RVSWI, right ventricular stroke work index; SV, stroke volume; SVR, systemic vascular resistance.
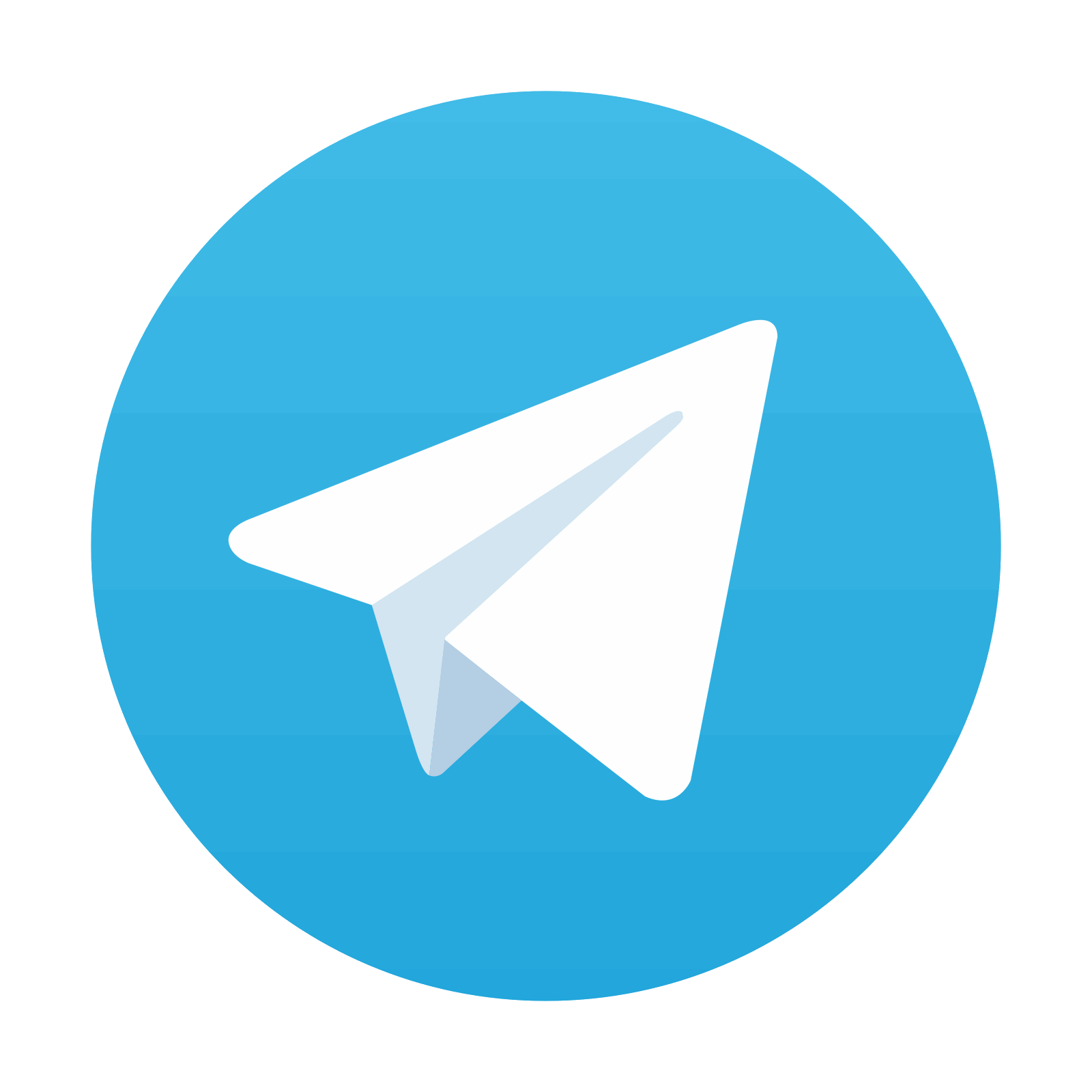
Stay updated, free articles. Join our Telegram channel

Full access? Get Clinical Tree
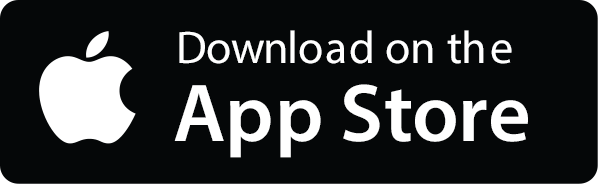
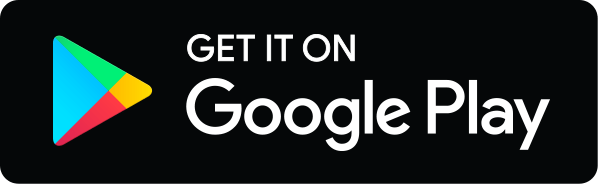