DIAGNOSTIC IMAGING IN ACUTE CARE SURGERY
The management of acute care surgical patients, as that of all patients, has largely benefited by advances in radiology. Current imaging techniques and modalities have vastly improved the ability to noninvasively diagnose and characterize disease and injury, which has largely supplanted the need for invasive exploratory surgery. Almost equally important, imaging can reliably exclude severe illness or injury in the acute presentation. This has resulted, for example, in a major shift in the management of trauma patients, in particular, with a greater emphasis on conservative management and reduction in the attendant morbidity and mortality introduced by surgical intervention and perisurgical (e.g. ICU) care.
There is a vast array of imaging modalities in the diagnostic armamentarium currently. While radiography, angiography, and nuclear medicine have undergone advances in their own right, the advances in magnetic resonance imaging (MRI), ultrasound (US), and, in particular, computed tomography (CT) have resulted in a major expansion in applications and extraordinary increases in utilization. This has led to justified concerns regarding health care costs, the need for outcomes research, and potential deleterious effects introduced by the imaging modalities themselves (e.g. radiation dose and CT). However, these imaging modalities each exhibit unique advantages that can be exploited for specific indications to help increase accuracy of, and decrease delays in, diagnosis. Additionally, these modalities also may have associated relative and absolute contraindications, which should be considered when considering utilization for a particular patient and clinical scenario.
In this chapter, we present these three imaging modalities in detail, introduce basic background concepts, and detail advantages and disadvantages as well as optimal target patient populations, clinical scenarios, and indications. Each subsection concludes with a gallery of images representing commonly encountered acute care surgical pathology, with detailed captions.
ULTRASOUND
Ultrasound is one of the most utilized and widespread diagnostic imaging modalities in medicine. Ultrasound images are generated by transmitting an ultrasonic pulse and detecting the amplitudes and delay times from returning acoustical echoes reflecting off of tissue interfaces. Typical operating frequencies range from 2 to 12 MHz. The major advantages of this modality include the ability to perform real-time noninvasive imaging without the use of ionizing radiation, its high degree of portability, its relatively low cost, and the capability to acquire images in an infinite number of planes. Additionally, by evaluating Doppler frequency shifts from moving blood, ultrasound can provide blood flow information by producing arterial or venous waveforms and quantitatively measuring blood flow velocities.
Ultrasound is particularly well suited to evaluate the abdomen and pelvis, owing to excellent acoustical windows provided by the liver, spleen, and bladder. The liver window provides a view of the gallbladder, pancreas, right kidney, heart, and right pleural space. The spleen is used to image the left kidney and left pleural space. A full bladder can allow a thorough assessment of the uterus and adnexa. Transvaginal, transrectal, transesophageal, and endoscopic probes are also available for more detailed imaging of the pelvis, prostate, rectum, heart, upper gastrointestinal tract, and pancreas. Ultrasound is also the primary imaging method used for assessment of the fetus, as no ionizing radiation or contrast materials are required, and the transmitted acoustic energy in typical obstetric examinations is accepted as safe.1 Furthermore, thoracentesis,2 paracentesis, superficial abscess drainage, percutaneous cholecystostomy, and other minimally invasive bedside procedures are commonly performed under real-time ultrasound guidance.
Common uses of ultrasound in the acute surgical setting include evaluation for acute cholecystitis (Fig. 9.1),–46 renal obstruction or calculi (Fig. 9.2), vascular occlusion,7 ovarian or testicular torsion (Fig. 9.3),9,10 and appendicitis (Fig. 9.4).11 Because of its portability and real-time imaging capability, ultrasound is also often employed in the trauma setting to assess patients who may be too unstable for a CT examination. Ultrasound can readily evaluate for solid organ injury within the liver, spleen, and kidneys. Additionally, the Focused Assessment with Sonography for Trauma (FAST) exam is a common bedside ultrasound study used to detect free fluid within the peritoneal, pericardial, and pleural spaces as a first-step measure to identify an internal hemorrhage (Fig. 9.5).–1315
FIGURE 9.1. Acute cholecystitis. A: A sagittal view shows a dilated gallbladder with a thickened wall (arrow) and a large shadowing stone (arrowhead). B: A transverse view demonstrates the presence of layering hyperechoic sludge (arrow). Sonographic signs that favor acute cholecystitis include wall thickening > 3 mm, gallbladder dilation, impacted stone, the presence of a sonographic Murphy’s sign, and pericholecystic fluid.3 Acute cholecystitis was confirmed at surgery. GB, gallbladder; LIV, liver; KID, kidney.
FIGURE 9.2. Hydronephrosis secondary to obstructing ureteral calculus. A: Moderate pelvic and calyceal dilation is present within the left kidney (asterisk). Urine and other simple fluids appear anechoic on ultrasound. The kidney is outlined by the caliper markers (+), and the cortex is appropriately homogeneous in echotexture. B: A 1-cm shadowing obstructive ureteral calculus, outlined by caliper markers (+), was found at the left ureterovesical junction.
FIGURE 9.3. Ovarian torsion. A: Transvaginal sagittal grey-scale ultrasound image of the left ovary demonstrates an enlarged left ovary measuring up to 8 cm with small, peripherally located follicles (arrows) and slightly heterogeneous stroma. B: Duplex ultrasound image of the left ovary demonstrates absence of normal arterial or venous spectral Doppler waveforms. C: In contrast, the normal right ovary demonstrates normal arterial waveforms. Upon surgery, the left ovary was twisted 720 degrees. Ultrasound features of ovarian torsion include unilateral enlarged ovary >4 cm, peripherally located small follicles, coexistent mass within the torsed ovary, presence of free pelvic fluid, and visualization of a twisted vascular pedicle. On color Doppler imaging, absence of arterial flow is a classic feature of ovarian torsion, although preserved normal flow may also be seen.8
FIGURE 9.4. Acute appendicitis. A: Graded compression ultrasound imaging at the area of tenderness in the right lower quadrant demonstrates a tubular, blind-ending structure measuring 8 mm in diameter, as marked by the calipers (+), compatible with a dilated appendix, which was noncompressible. B: A transverse view demonstrates the appendix to have an abnormally thickened wall, as outlined by the calipers (+). Sonographic signs for appendicitis include a diameter >6 mm, lack of compressibility, echogenic periappendiceal fat, hyperemia on color Doppler imaging, the presence of an appendicolith, and the presence of adjacent fluid collections that would raise concern for rupture.3 Acute appendicitis was confirmed upon surgery.
FIGURE 9.5. Hemoperitoneum in Morrison’s pouch.A hypoechoic collection (*) is seen between the inferior edge of the right hepatic lobe (L) and the right kidney (K) in this patient with hemoperitoneum. Fresh blood appears hypoechoic on ultrasound. Acute clots are initially hyperechoic; the echogenicity diminishes with time.12
Drawbacks of ultrasound include its limited ability to assess the brain, thorax, and bowel due to the presence of tissue–air and tissue–bone interfaces that are nearly completely acoustically reflective and therefore prevent the penetration of ultrasound waves. Ultrasound imaging of obese patients is also challenging, often resulting in low-quality images due to poor penetration of the ultrasound beam. Additionally, ultrasound can be subject to imaging artifacts from oblique scattering and multiple reflections.16 Most importantly, quality ultrasound imaging is highly dependent on operator skill and experience.
Table 9.1 summarizes the advantages and disadvantages of ultrasound.
TABLE 9.1
ADVANTAGES AND DISADVANTAGES OF ULTRASOUND
Figures 9.1 through 9.5 demonstrate common acute care surgical pathology optimally diagnosed via ultrasound.
MAGNETIC RESONANCE IMAGING
Magnetic resonance imaging (MRI) is performed through manipulation of the magnetic moment of water hydrogen protons within a static magnetic field. The behavior of these moments, or spins, is directly related to the environment surrounding the water molecule through local effects such as magnetic fields from neighboring spins and energy exchange with nearby nuclei. Images are created after applying pulsed radiofrequency (RF) waves and magnetic field gradients at calculated times, then extracting spatial and temporal data from the subsequent signal patterns. Each programmed set of RF and gradient settings is referred to as a pulse sequence. The signal from protons within fat, protein, or free water can be isolated and highlighted through different pulse sequences, allowing for excellent soft tissue contrast that is superior to other cross-sectional imaging methods.17 Isometric 3D MRI is now commonly used for multiplanar and curved reconstructions of vascular and ductal structures. Multiple types of contrast agents can be used to highlight the gastrointestinal tract, vascular structures, or bile ducts.18,19 Interventional MRI continues to be an active area of research, particularly in oncology.20
A particular advantage of MRI is that this modality does not employ ionizing radiation, and, therefore, is favored whenever there is a particular desire to limit or avoid radiation exposure, such as in the evaluation of pregnant patients (Figs. 9.6–9.8).22,23 MRI is also increasingly considered as a primary imaging modality in the evaluation of chronic diseases where the cumulative radiation dose from frequent follow-up CT examinations would be quite substantial. Inflammatory bowel disease is one such entity, where rapid pulse sequences are now able to compensate for peristaltic motion, and T2-weighted, fluid-sensitive sequences are particularly useful for detecting fistulas or abscesses (Figs. 9.9 and 9.10).–2527
FIGURE 9.6. Acute appendicitis in pregnancy. A: Coronal and (B) axial T2-weighted images of a pregnant female presenting with right lower quadrant pain demonstrate a dilated appendix with thickened walls and bright intraluminal contents, compatible with fluid (arrows). High signal intensity within the adjacent fat is compatible with periappendiceal stranding and edema. These findings, in combination with an appendiceal diameter >7 mm, are consistent with acute appendicitis.21 Medial and inferior to the appendix is the gravid uterus (U). C, cecum. C: Axial diffusion-weighted sequence performed at the same axial level shows increased signal intensity from restricted water motion within the appendiceal wall and lumen, compatible with inflammation (arrows). Appendicitis was confirmed surgically.
FIGURE 9.7. Ischemic bowel in pregnancy. A: Coronal T2-weighted image in a pregnant woman at 25 weeks’ gestational age demonstrates fluid-filled loops of small bowel (black arrows) in the left abdomen with free intraperitoneal fluid (white arrow). F, fetus. B: Axial T2-weighted image demonstrates thickening of the proximal loops of small bowel with a target appearance compatible with mural edema. At surgery, the patient was discovered to have a small bowel volvulus secondary to an adhesion resulting in extensive small bowel ischemia. Ischemic bowel on MRI may demonstrate wall thickening and mural stratification or a target appearance, with high signal within the wall on T2-weighted imaging indicative of mural edema.32,63
FIGURE 9.8. Small bowel obstruction in pregnancy. A: Coronal T2-weighted image in a pregnant female with a history of total colectomy for ulcerative colitis demonstrates diffusely dilated loops of small bowel and free fluid within the abdomen (arrows). F, fetus. B: Axial T2-weighted image shows dilated loops of small bowel with abrupt tapering within the end ileal loop and a transition point noted at the level of the ostomy due to a stricture (arrow).
FIGURE 9.9. Active Crohn’s disease.Coronal, fat-suppressed, T1-weighted image of the abdomen obtained after the administration of intravenous contrast demonstrates a matted, abnormal appearance to the loops of small bowel within the midline abdomen. These bowel loops show intense bowel wall enhancement, mural thickening, luminal narrowing, and adjacent mesenteric stranding and enhancement compatible with active inflammation (arrows).24
FIGURE 9.10. Complex Crohn’s disease. A: Sagittal T2-weighted image of the pelvis demonstrates an abnormally thickened rectum (R) and distal sigmoid colon, in contrast to the proximal sigmoid colon (S), which appears normal. A superficial tract compatible with a perianal fistula is present (arrow). P, pubic symphysis; B, bladder; U, uterus, L5/S1: vertebral bodies. B: Axial T2-weighted image of the lower pelvis demonstrates abnormally thickened distal sigmoid colon (arrows). The adjacent proximal sigmoid is normal with smooth, thin walls. I: iliac bones. Axial (C) T2-weighted and (D) postcontrast T1-weighted fat-suppressed images of the perineum demonstrate a perianal fistula tracking along the right gluteal fold (arrows) with marked inflammatory enhancement within the fistula and surrounding soft tissues.
While magnetic resonance cholangiopancreatography (MRCP) is commonly used for assessment of the biliary tree, liver, and pancreas for oncologic staging, surgical planning, or radiotherapy targeting,–2831 in acute settings, MRCP is well suited for diagnosing emergent gallbladder and biliary tract conditions, including acute cholecystitis, choledocholithiasis, and acute cholangitis (Figs. 9.11 and 9.12).32 Furthermore, magnetic resonance angiography provides an excellent alternative to CT for the imaging of vascular abnormalities (Fig. 9.13), particularly when iodinated contrast agents cannot be used due to contrast allergies or poor renal function.
FIGURE 9.11. Choledocholithiasis.Coronal thick-slab heavily T2-weighted MRCP image of the biliary tree demonstrates multiple round filling defects within the gallbladder, cystic duct, and common bile duct (arrows) with mild intrahepatic and extrahepatic biliary dilatation.
FIGURE 9.12. Gangrenous cholecystitis with perforation. A: Axial T2-weighted image of the abdomen demonstrates a distended gallbladder (GB) with a thickened and edematous wall. B: Postcontrast T1-weighted fat-suppressed axial image shows inhomogeneous enhancement of the gallbladder wall and disruption of mucosal enhancement with a contained perforation (arrow). Characteristic features of gangrenous cholecystitis on MRI include a patchy pattern of gallbladder wall enhancement with an interrupted rim of mucosal enhancement.32,33
FIGURE 9.13. Abdominal aortic aneurysm with dissection. Coronal, contrast-enhanced, maximal-intensity projection image of the abdominal aorta demonstrates fusiform aneurysmal dilatation of the entire abdominal aorta with an extensive intimal flap (black arrow), compatible with dissection. The right renal artery is supplied by the true lumen (white arrow), while the left renal artery is supplied by the false lumen (arrowhead). Bilateral common iliac artery aneurysms are also present, with a more saccular, irregular appearance of the aneurysm on the right.
Drawbacks of MRI include the need for a large static magnetic field to achieve an adequate signal-to-noise ratio, requiring large superconducting coils that are relatively expensive to maintain. An MRI suite must also be specially shielded to avoid signal interference from electromagnetic noise. Furthermore, typical magnets have a narrow cylindrical bore that can induce claustrophobia and may not be able to accommodate obese patients or patients who require large support devices.
Another primary disadvantage of MRI is the inability to image patients with MRI-incompatible devices. The high magnetic field strengths of MRI are not compatible with ferromagnetic materials, such as many intracranial aneurysm clips. Additionally, RF pulses used to acquire MR images may interfere with electronic implants, including many current and older generations of cardiac pacemakers. MRI centers, therefore, require prescreening of all patients with a detailed checklist to ensure that the patient is safe for imaging and that all support equipment are MRI compatible.34 Even the suspected presence of embedded metal within the orbits warrants further evaluation, typically with a radiograph, as fluctuations in magnetic fields can cause current-induced heating and particle movement. Furthermore, metallic implants that are deemed safe for MRI still cause local magnetic field artifacts that distort and obscure adjacent structures, thereby degrading image quality.
Contrast reactions with gadolinium-based agents can occur, as they do with CT agents; however, particular care must be paid to patients with severely impaired renal function, who have an increased risk of developing nephrogenic systemic fibrosis, or NSF. Typical symptoms include swelling and tightening of the skin within the extremities, but can progress to involve the internal organs, including skeletal muscle, myocardium, lungs, kidneys, and dura mater, with potentially fatal outcomes and no effective treatments.–3436 The proposed pathogenesis is attributed to an abnormal activation of circulating fibrocytes as a response to residual gadolinium in tissues that has remained long after initial administration. Since the first description of NSF in 1997, there have been approximately 335 biopsy-confirmed cases worldwide, but virtually no new cases since 2008 due to widespread restrictions on gadolinium-based contrast use in patients with severe renal failure.37 The use of intravenous gadolinium-based contrast agents is also not considered safe in pregnancy.38 With the recent development of robust noncontrast MRI sequencing techniques, these issues related to contrast administration, fortunately, can be circumvented in many cases.
Motion-related artifacts are a common occurrence in MRI. Many sequences require the patient to lie still for a substantially longer amount of time than that needed for a CT or ultrasound, with some sequences lasting several minutes. Additionally, the total duration of an MRI examination can be lengthy compared to other modalities, typically lasting 40 minutes. As a result, sedation is often required in the pediatric population in order to obtain images free of motion artifact. Breath-hold imaging acquisitions can reduce the total imaging time and are frequently used to eliminate respiratory-related motion artifacts. Acutely ill patients who are unable to suspend their respiration, however, pose a challenge for MRI. The use of non–breath-hold, rapid imaging strategies can allow interpretable MR images to be acquired in these sicker patients.
Finally, MRI has a limited capability to image structures that have low water proton density. As a result, the detection of calcifications on MRI can be difficult. Furthermore, imaging of the lungs with MRI is particularly challenging as these structures contain mostly air and, therefore, generate little signal. The multiple air–tissue interfaces within the lung parenchyma also increase the rate of signal decay, further limiting the imaging of the pulmonary parenchyma.
Table 9.2 summarizes the advantages and disadvantages of MRI.
TABLE 9.2
ADVANTAGES AND DISADVANTAGES OF MRI
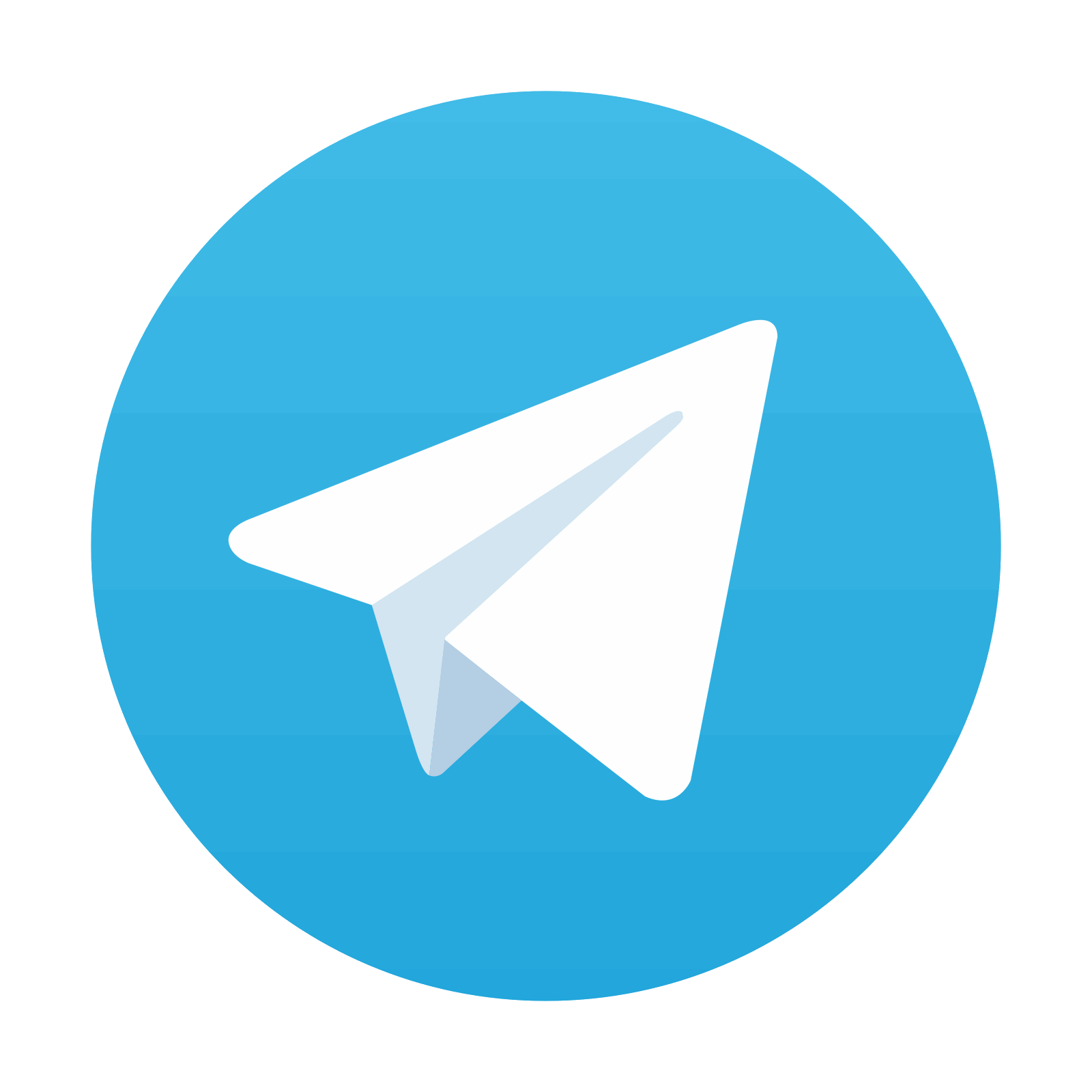
Stay updated, free articles. Join our Telegram channel

Full access? Get Clinical Tree
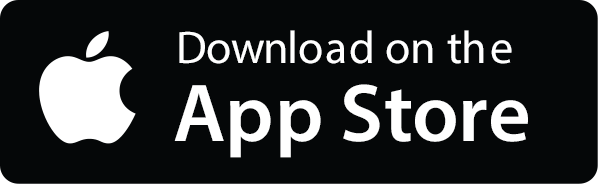
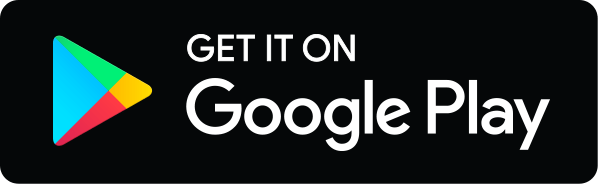