BURNS*
The organ system changes that occur following burn injury represent the stereotypic response to injury and make the burn patient the universal trauma model (Table 32.1). Those changes and the frequency of burn injury make burn patient management a challenge often presented to the trauma/acute care surgeon. The acute care surgery components of initial burn care include fluid resuscitation, and ventilatory support, as well as preservation and restoration of function of other organs and tissues. Following resuscitation, burn patient management is focused on wound care and provision of the necessary metabolic support. The involvement of the trauma/acute care surgeon in burn wound management is dependent upon the extent of the wound, depth of the wound, and site of care, that is, a general hospital in which a trauma/acute care surgeon might provide definitive wound care for a patient with burns of limited extent as opposed to a burn center where the wound care of a patient with extensive burns would be provided by a burn surgeon. The trauma/acute care surgeon must be able to identify those patients who are best cared for at a burn center and oversee their safe, prompt transfer.
EPIDEMIOLOGY
Only 21 states require the reporting of burn injury and in 9 of those states, only specific burns defined by etiology or extent must be reported. Consequently, an estimated total number of burns has been obtained only by extrapolation of the data collected in those states to the entire population. In 1996, 1.25 million was regarded as a realistic estimate of the annual incidence of burns in the United States.1
On the basis of data from federal surveys and the National Burn Registry, the American Burn Association estimates that in the United States, 450,000 burn injury patients receive medical treatment annually. The percentage of burn patients cared for at burn centers has increased steadily in recent years and of the 40,000 (~130 patients per million population) admitted to hospitals for treatment of their burn injury, two-thirds or 26,400 (~86 patients per million population) are cared for at the 125 hospitals with specialized burn centers of which 54 are verified by the American Burn Association.2 That subset of patients defined by the American Burn Association (Table 32.2), as best cared for in a burn center3 consists of 40 patients per million population with major burns and 46 patients per million population having lesser burns but a complicating cofactor. The geographic distribution of burn centers, which correlates closely with population density,4 necessitates the use of aeromedical transfer by either fixed wing or rotary wing aircraft to transport burn patients to those facilities from remote areas.
TABLE 32.2
ABA BURN CENTER REFERRAL CRITERIA
There are identifiable populations at high risk for specific types of injuries that will require treatment by the trauma/acute care surgeon. Thermal burns outnumber scald burns by more than a factor of 2 in children of all ages treated in emergency departments for burns5 even though scald burns are the most frequent form of burn injury overall.6 The occurrence of tap water scalds can be minimized by adjusting the temperature settings on hot water heaters or by installing special faucet valves that prevent delivery of water at unsafe temperatures.
In adults, flames and the ignition of flammable liquids are the most common causes of burns. In patients of 80 or more years, scalds and flames each cause approximately 30% of burn injuries. Preexisting disease contributes to the injury event in approximately two-thirds of elderly patients and also contributes to their morbidity and mortality rates, which are higher than in younger patients.7
The vast majority of the burns treated at hospitals involve <20% of the total body surface area (TBSA) as reflected by the overall survival rate of 94.8%. The American Burn Association National Burn Registry Advisory Committee has estimated that there are only “4,000 fire and burn deaths each year with 75% of those occurring at the scene or during initial transport”. Of patients admitted to burns centers, 63% are Caucasian and 70% are male. The cause of the burn injury in those patients was flame/fire 42%, scald 31%, hot object contact 9%, electric injury 4%, chemical injury 3%, and other 11%. Personal assault and self-inflicted injuries each accounted for approximately 2% of the burns. The home was the site of occurrence of the burn injury in two-thirds of the patients and, only 1 in 10 occurred at the work place.2 However, one-fifth to one-quarter of all serious burns are employment related. Kitchen workers are at relatively high risk for scald injury, and roofers and paving workers are at greatest risk for burns due to hot tar.
In 1988, there were 236,200 patients treated in emergency rooms for chemical injuries.8 Many chemical injuries are employment related. Strong acid injuries are of greatest incidence in those involved in plating processes and fertilizer manufacture and strong alkali injury is of greatest incidence in those involved in making soap. Injuries caused by phenol, hydrofluoric (HF) acid, anhydrous ammonia, cement, and petroleum distillates are also strongly associated with employment.
Injuries due to white phosphorus and mustard gas are most frequent in military personnel. Civilian recreational explosive devices, fireworks, are a seasonal cause of burn injury. The highest incidence of firework injuries occurs during the 4th of July holiday in the United States, and during religious celebrations in countries such as India.
Electric current causes approximately 1,000 deaths per year.9 One-quarter of electric injuries occur on farms or industrial sites, and one-third occur in the home. Young children have the highest incidence of electric injury caused by household current as a consequence of inserting objects into an electrical receptacle or biting or sucking on electric cords and sockets. Adults at greatest risk of high voltage electric injury are the employees of utility companies, electricians, construction workers (particularly those manning cranes), farm workers moving irrigation pipes, oil field workers, truck drivers, and individuals installing antennae. National death certificate data document an average of 107 lightning deaths annually.10 The vast majority (92%) of lightning-associated deaths occur during the summer months when thunderstorms are most common. Slightly more than one-half of patients killed by lightning were engaged in outdoor activities such as golfing or fishing, and a quarter of patients who died from lightning injury were engaged in employment-related activities.11
Child abuse is a special form of burn injury, typically inflicted by parents but also perpetrated by siblings and child care personnel. The most common form of thermal injury abuse in children is caused by intentional application of a lighted cigarette. Burning the dorsum of a hand by application of a hot clothing iron is another common form of child abuse. The burns in abused children who require in-hospital care are most often caused by immersion in scalding water with the injury typically involving the feet, posterior legs, buttocks, and sometimes the hands (Fig. 32.1). It is important that the trauma/acute care surgeon identify and report child abuse because if it is undetected and the child is returned to the abusive environment, repeated abuse is associated with a high risk of fatality. In recent years, elder abuse has become more common and it too should be reported and the victim protected.
FIGURE 32.1. The distribution of the burns on this child (feet, legs, posterior thighs, buttocks, and perineum) is characteristic of abuse by intentional immersion scalding. The bright red moist burns of the buttocks and left posterior thigh were partial thickness burns, while the darker red, dry burns of the legs and feet, which darkened further with time, were full thickness injuries, which required grafting for closure.
PATHOPHYSIOLOGY
Local effects
The depth of burn injury produced, that is, partial thickness or full thickness, is related to the temperature of the energy source, the duration of the exposure, and the tissue surface involved. At temperatures <45°C, tissue damage is unlikely to occur in either adults or children even with an extended period of exposure. In the adult, exposure for 30 seconds when the temperature is 54°C will cause a burn injury.12 In the child with relatively thinner skin, exposure to this same temperature for 10 seconds produces a substantial degree of tissue destruction. When the temperature is elevated to 60°C, a not uncommon setting for home water heaters, tissue destruction can occur in <5 seconds in children. At 71°C, a full thickness burn can occur in a near instantaneous manner. It is no surprise that when patients come in contact with boiling liquids, live flames, or are injured in industrial accidents where temperatures can exceed 1,000°C, substantial depths of injury occur. The systemic consequences of the injuries are proportional to the extent of the body surface area involved and may be modified by the patient’s underlying physiological status and the presence of concomitant mechanical trauma. As the depth of the burn increases from partial to full thickness, the systemic response increases but to a lesser degree than that associated with increase in burn extent.
A burn injury causes three zones of damage (Fig. 32.2A). Centrally located is the zone of coagulation necrosis. Surrounding this is an area of lesser cell injury, the zone of stasis, and surrounding that an area of minimally damaged tissue, the zone of hyperemia, which abuts undamaged tissue. In a full thickness burn, the zone of coagulation involves all layers of the skin extending down through the dermis and into the subcutaneous tissue (Fig. 32.2B). In partial thickness injuries, this zone extends down only into the dermis and there are surviving epithelial elements capable of ultimately resurfacing the wound. In the zone of stasis, blood flow is altered but is restored with time as resuscitation proceeds. If thrombosis were to occur in a patient who is not adequately resuscitated, the zone of stasis can be converted to a zone of coagulation. The zone of hyperemia is best seen in patients with superficial partial thickness injuries as occur with severe sun exposure.
FIGURE 32.2. A: In any burn there are three identifiable concentric zones of injury. The most severe injury is in the zone of coagulation in which all tissue is nonviable. Surrounding that is the zone of stasis in which injured tissue with impaired blood flow can progress to necrosis if resuscitation is inadequate or be salvaged with adequate resuscitation. Surrounding the zone of stasis is the zone of hyperemia that contains the least injured tissue and is characterized by surface erythema and increased blood flow as manifestations of the inflammatory response. B: Diagram of the skin showing the levels of tissue injury defining second degree or partial thickness burns in which epithelial cells migrating from skin adnexa will resurface the wound and third degree or full thickness burns in which the dermis and adnexa are destroyed and must be closed by grafting.
Along with the changes in wound blood supply there is considerable formation of edema in the burn-injured tissues. Release of local mediators from the burned tissue as well as from leukocytes causes alterations in local tissue homeostasis. Factors elaborated in the damaged tissues include histamine, serotonin, bradykinin, prostaglandins, leukotrienes, and interlukin-1. Complement is also activated, which can further modify transcapillary fluid flux. The changes in tissue water content have been ascribed to increased capillary filtration as well as changes in interstitial hydrostatic pressure.13–16 The net effect of these various changes is appreciable movement of fluid into the extravascular fluid compartment. The ongoing development of edema fluid in the burn-injured tissue conceptually represents increased vascular permeability. Subsequent changes in lymph flow from burned tissue have been ascribed to changes in lymphatic vessel patency with obstruction occurring due to serum proteins that have transmigrated from the damaged capillaries.17 Maximum accumulation of both water and protein in the burn wound occurs at 24 hours post injury.18 This accumulation in tissues can remain beyond the first week post-burn. In addition to the changes in transcapillary fluid movement within the burn injured tissues, patients who have greater than a 20%-25% body surface burn have similar fluid movement in undamaged tissue beds including the gut and the lung. This may in part be related to the changes in transcapillary fluid flux and also be in response to the volume of resuscitation fluids administered.19,20
The appearance of the injuries is determined by the level of tissue destruction (Fig. 32.3A). When the wounds are superficial, they are associated with hyperemia, fine blistering, increased sensation, and intense pain upon palpation. The wounds are erythematous, warm, and readily blanch. These types of injuries represent first-degree burns or alternatively are termed superficial partial thickness injuries. With a second degree or deeper partial thickness burn, the wound presents with intact or ruptured blisters or is covered by a thin coagulum or crust. The key physical finding is preservation of sensation in the burned tissue although it is reduced (Table 32.3).
FIGURE 32.3. A: The partial thickness burns on this patient’s arm display the characteristic blisters and areas of exfoliation of superficial epithelium revealing a pink moist wound shown just below the elbow. B: The full thickness burns on the left leg and foot of this child display the leathery, pallid, coagulated eschar characteristic of third degree burns. Edema beneath the circumferential eschar necessitated the escharotomy incision evident in the midlateral line of the burned leg and the lateral aspect of the foot. Note that the escharotomy was carried across the involved ankle joint.
With proper care, superficial and even deeper partial thickness injuries are capable of healing. Even though burn blister fluid represents a near pure acellular filtrate of plasma, studies have revealed that this fluid may not necessarily promote wound healing.21 Infection risk in deep partial thickness wounds is considerable and if an infection develops it can lead to greater tissue destruction and conversion to a full thickness injury. When the injury penetrates all layers of the skin or extends into the subcutaneous or deeper tissues, the wound will appear pale or waxy, be anesthetic, dry, and inelastic, and contain thrombosed vessels (Fig. 32.3B). Occasionally in children or young women, the wound may initially be cherry or brick red in appearance and be mistakenly considered to be partial thickness injury (Fig. 32.1). Such wounds typically have substantial edema, and are inelastic and insensate. Over the ensuing days, the wound darkens and becomes characteristic of a full thickness wound as the extravasated hemoglobin, which when fully oxygenated immediately post injury is responsible for the misleading wound color, undergoes reduction. Full thickness wounds are infection prone wounds, as they no longer provide any viable barrier to invading organisms and if left untreated are rapidly colonized and become a portal for invasive burn wound sepsis.
Systemic Response
The organ system response to a major burn injury results in some of the most profound physiologic changes that can occur. The magnitude of the response is proportional to the burn size and in some organ systems reaches a maximum at about a 50% body surface area burn. The duration of the changes is related to the persistence of the burn wound and the changes resolve with wound closure. The organ specific response follows the pattern that occurs with other forms of trauma with an initial level of hypofunction followed by the hyperdynamic flow phase. The changes in the cardiovascular response are some of the more critical ones and directly impact the initial care and management of the burn patient. Following burn injury, there is a transient period of decreased cardiac performance in association with elevated peripheral vascular resistance. This can be further compounded by failure to replace adequately the patient’s intravascular volume loss leading to further impairments of cardiac filling, decreased cardiac output, and worsening organ hypoperfusion. Systemic hypoperfusion can result in further increases in systemic vascular resistance and reprioritization of regional blood flow. Whether the burn is responsible for causing a myocardial depressant to appear in the circulation or the impaired cardiac performance is simply a consequence of inadequate volume restoration remains an open question. What seems to be clear from experimental studies is that when there is a failure to resuscitate a burn patient adequately there is substantial impaired myocardial performance.22 Conversely, the provision of adequate resuscitation volumes can preserve cardiac performance.23
Patients receiving appropriate volume restoration during the course of their resuscitation, develop normal cardiac performance values within 24 hours of injury and by the second 24 hours, those values further increase to supranormal levels. It is not uncommon to see adult patients with cardiac outputs in excess of 10 Liters per minute. In association with changes in cardiac output, there is a reduction in the systemic vascular resistance to 30%-40% of normal values. This hyperdynamic flow phase, part of the hypermetabolic response to the injury, will decrease toward normal levels with wound closure, but some degree of metabolic rate increase persists in major burn survivors for more than a year as the healed wounds fully mature.
Pulmonary changes following burn injury are the consequences of direct parenchymal damage as occurs with inhalation injury and the pathophysiologic effects of the burn per se. With isolated burn injury, neutrophil sequestration occurs in the lungs and may mediate lung injury. One mediator of this response appears to be the platelet-activating factor, which serves to prime neutrophils.24 The changes in the pulmonary vascular response parallel those of the peripheral circulation though the increase may be to a greater degree and with a longer duration of change.25 Capillary permeability in the lung appears to be mostly preserved following burn injury with the primary change being an increase in the lung lymph flow but no change in the lymph-to-plasma protein ratio.26 Lung ventilation increases in proportion to the extent of burn with the patient having both an increase in respiratory rate and tidal volume. The increases are primarily related to the overall hypermetabolic response to the burn injury. Further perturbations in the patient’s ventilatory status not related to the presence of an inhalation injury would indicate a supervening process such as sepsis, pneumonia, occult pneumothorax, pulmonary embolism, congestive heart failure, or an acute intraabdominal process.
The renal response to burn injuries is largely orchestrated by the cardiovascular response. While initially there may be a reduction in renal blood flow, this is restored with resuscitation. If the resuscitation is delayed or the fluid need underestimated, renal hypoperfusion will occur with early onset renal dysfunction secondary to renal ischemia. If the patient also experiences myoglobinuria or hemoglobinuria, which are capable of causing direct tubular damage, sequential injury can occur leading to further impairment of renal function. The changes in renal blood flow following burn injury require adjustment of the dosing schedule of a variety of medications such as aminoglycoside antibiotics to attain therapeutic levels. In patients who are receiving nutritional support, large doses of carbohydrates can cause glycosuria resulting in an inappropriate osmotic diuresis necessitating therapeutic intervention, that is, reduction of glucose load and/or administration of insulin. Daily urinary outputs in burn patients who are receiving protein loads greater than normal may be increased by the effects of the urinary urea load necessitating adjustment of fluid intake in order to excrete the increased solute load and avoid hypovolemia.
Burn-induced changes in gastrointestinal (GI) tract motility and a reduced capacity to tolerate early feedings had been previously thought to preclude the use of the GI tract as the primary route for nutritional support. With near immediate institution of enteral feedings via nasogastric or nasoduodenal tubes, GI motility can be preserved, mucosal integrity protected, and effective nutrient delivery achieved. It seems that delay in the initiation of enteral feeding is associated with the onset of ileus, which can also occur when the burn resuscitation has been complicated. Patients who are under-resuscitated will have alterations in GI tract motility and mucosal integrity as a consequence of intestinal hypoperfusion. Patients who have received massive resuscitation volumes will have considerable edema of the retroperitoneum, bowel mesentery, and bowel wall leading to a paralytic ileus. In patients who are intoxicated at the time of their burn injury, there may be further alterations in the GI tract with changes in the mucosal barrier function and alterations in local immunity.27 In the past, the major GI complications following burn injuries were related to upper GI ulceration and bleeding. However, there has been a relative shift in the site of post-burn GI complications with the small bowel and colon now being more often affected.28
Burn injury results in an elevated hormonal and neurotransmitter response similar in magnitude to that of the “fight or flight” response.29 The duration of the neurohumoral response is prolonged and it can be further increased with surgical stress. This can adversely impact the burn-induced metabolic changes and immune response. The increases in glucocorticoids and catecholamines correctly support the stress response of the injured patient except where this response becomes dysfunctional. In pathologic studies in humans as well as in animals, when there is an insufficient stress-hormone response, an otherwise survivable insult becomes fatal. Many of the multisystem changes occurring postburn can be related in part to the alterations in catecholamine secretion particularly the changes in resting metabolic expenditure, substrate utilization, and cardiac performance. As wound closure is accomplished, the altered neurohumoral response abates as the catabolic hormones recede and the anabolic hormones become predominant.
Burn injury results in the loss of balance in both leukocyte and erythrocyte production and function. Burns of >20% TBSA are associated with alterations in red cell production resulting in anemia.30 Patients with major thermal injuries may lose up to 20% of their red cell mass in the first 24 hours due to thermal destruction of red cells in the cutaneous circulation. Such loss can be further compounded by frequent blood draws, blood loss related to surgical procedures, hemodilution with resuscitation and transient alterations in erythrocyte membrane integrity. Longer-term changes appear to be related to hyporesponsiveness of the erythroid progenitor cells in the bone marrow to erythropoietin.31 Burn patients manifest increased circulating levels of erythropoietin following injury and attempts to augment those levels to improve red cell production have met with little success. During the early stages of resuscitation, reductions in platelet number, depressed fibrinogen levels, and alterations in coagulation factors return to normal or near normal values with appropriate resuscitation. Subsequent changes if they occur may be related to a septic process or in the case of platelets, heparin-induced platelet antibodies if heparin flushes are used as part of the maintenance protocol for intravascular devices. Changes in white cell number occur early with an increase in neutrophils due to demargination and accelerated bone marrow release. With uncomplicated burn injury, bone marrow myelopoiesis is relatively preserved.32 With a septic complication, there appears, based on experimental evidence, to be a reduction in granulocyte formation and a relative shift to monocytopoiesis.33 This defect appears not to be related to a lack of granulocyte colony stimulating factor but a growth arrest within the bone marrow of granulocyte precursor cells.34,35
In addition to the changes occurring in the bone marrow and the nonspecific aspects of the host defense mechanisms, there are substantial further depressions in the immune response. Burn injury causes a global impairment in host defense mechanisms. Alterations of the humoral immune response include reductions in IgG and IgM secretion, decreased fibronectin levels, and increases in complement activation. Cellular changes include alterations in T-cell responsiveness, changes in the T-cell subpopulations favoring the cytotoxic/suppressor T-cell, alterations in antigen processing and presentation, reductions in IL-2 release, and impairment of delayed type hypersensitivity reactions. In addition to the changes noted in granulocytes and monocytes and their release from the bone marrow, there are corresponding functional changes. Granulocytes have been noted to have impaired chemotaxis, decreased phagocytic activity, decreased antibody-dependent cell cytotoxicity, and a relative impairment in their capacity to respond to a second challenge.36 The relative shift to monopoiesis is associated with an increase in secretion of PGE2. 37 Dendritic cells, a critical component in the immune response, have also been found to be considerably altered following burn injury with infection.38 Majetschak et al., who studied circulating proteasomes after burn injury identified elevation of 20 S proteasomes, which peaked on the day of injury, gradually declined during the ensuing 7 days, and had returned to baseline by postburn day 30. The proteasome levels did not discriminate for sepsis, multiple organ failure, or survival, but the circulating 20 S proteasomes were considered to be biomarkers of tissue damage and potentially useful in diagnosing inhalation injury.39
Recent studies of 242 pediatric patients with burns of more than 40% of the TBSA by Jeschke et al.40 have documented the pervasiveness, intensity, and duration of the pathophysiologic response evoked by a major burn. Every patient was markedly hypermetabolic throughout their hospital stay and sustained substantial muscle protein loss and loss of lean body mass. Bone mineral density and bone mineral content decreased as well. Serum proteomic analysis revealed profound changes immediately after injury which, with variable changes across time, persisted throughout hospital stay. Cardiac function was compromised immediately after burn and was still abnormal at discharge. Insulin resistance was evident in the first week after injury and still present at discharge. Striking changes in IL-8, MCP-1, and IL-6 induced an inflammatory state and were associated with infections and sepsis. The clinical importance of these observations is that the burn patient is at significant risk for postburn infectious complications. This mandates the strictest adherence to aseptic technique in the management of the wounds and the insertion of intravascular devices, the judicious use of antimicrobial agents, aggressive nutritional support, and the achievement of rapid wound closure.
RESUCITATION
Priorities
In the immediate postburn period, the changes induced in the cardiovascular system by burn injury receive therapeutic priority. If the early postburn plasma volume loss is unreplaced, burn shock may occur accompanied by kidney and other organ failure and even death. In all patients with burns of more than 20% of the TBSA and those with lesser burns in whom physiologic indices indicate a need for fluid infusion, a large caliber intravenous cannula should be placed in an appropriately sized peripheral vein underlying unburned skin. If no such sites are available, a vein underlying the burn wound may be cannulated. If there are no peripheral veins available, the cannula can be placed in a femoral, subclavian, or jugular vein. Lactated Ringers solution should be infused at an initial rate of 1 Liter per hour in the adult and 20 mL/kg/h for children who weigh 50 kg or less. That infusion rate is adjusted following estimation of the fluid needed for the first 24 hours following the burn.
Fluid Administration
Resuscitation fluid needs are proportional to the extent of the burn (combined extent of partial and full thickness burns expressed as a percentage of TBSA) and are related to body size (most readily expressed as body weight) and age (the surface area per unit body mass is greater in children than in adults.) The patient should be weighed on admission and the extent of partial and full thickness burns estimated according to standard diagrams (Fig. 32.4) or, in the adult, by the use of the rule of nines that recognizes the fact that the surface area of various body parts represents 9% or a multiple thereof of the TBSA, that is, each upper limb and the head and neck 9%, each lower limb, posterior trunk and buttocks, and anterior trunk 18%, and the perineum and genitalia 1%. Those surface area relationships differ in children in whom the head and neck represent 21% of the TBSA and each lower limb, 14% at age one. The fraction of the TBSA represented by the head decreases progressively and that represented by the lower limbs increases progressively reaching adult proportions at age 16. The fact that the palmar surface of the patient’s hand (palm and digits) represents 1% of his or her total body surface can be used to estimate the extent of irregularly distributed burns, that is, the number of the patient’s “hands” needed to cover the patient’s burn wounds.41
FIGURE 32.4. This diagram can be used to determine the extent of a patient’s burn. Areas of the figures are colored with a blue pencil to indicate partial thickness burns and a red pencil to indicate full thickness burns. The columns with numbers, to the left below the figures, indicate how the percentage of body surface represented by various body parts changes with age.
In recent years, increased recognition of the association between resuscitation volume and compartment syndromes has focused interest on minimizing resuscitation fluid volume and increased use of those formulae estimating the fluid needed for the first 24 hours as 2.0 mL/kg body weight/percent of body surface burned as opposed to those formulae estimating first 24 hour fluid resuscitation needs as 4.0 mL/kg body weight/percent body surface area burned.42,43–44
In a study of 105 combat casualties with burns of more than 20% of the TBSA, the investigators reported that resuscitation guided by the modified Brooke Formula resulted in significantly lower first 24-hour fluid volume without any greater morbidity and mortality than in those for whom resuscitation was guided by the Parkland Formula. A greater percentage of those patients resuscitated with the Parkland Formula exceeded the Ivy index (250 mL of resuscitation fluid per kilogram body weight), which was associated with a high risk for abdominal compartment syndrome and was an independent predictor of death.45
In line with such reports, the American Burn Association’s 2010 Advanced Burn Life Support (ABLS) manual has revised the recommendations for estimating the first 24-hour resuscitation fluid needs of burn patients.46 One half of the estimated volume should be administered in the first 8 hours after the burn. If the initiation of fluid therapy is delayed, the initial half of the volume estimated for the first 24 hours should be administered in the hours remaining before the eighth postburn hour. The remaining half of the fluid is administered over the subsequent 16 hours. The infused volume is increased only as needed to achieve adequate resuscitation (Table 32.4).
TABLE 32.4
ESTIMATION OF FIRST 24 HOUR FLUID RESUSCITATION NEEDS
a TBSA, total body surface area.
b The greater surface area per unit body mass in children necessitates a greater first 24′ resuscitation fluid volume.
The limited glycogen stores in a child may be rapidly exhausted by the marked stress hormone response to burn injury. Serum glucose levels in the burned child should be monitored and 5% dextrose in half normal saline administered if serum glucose decreases to hypoglycemic levels. In the case of small children with small burns, the resuscitation fluid volume as estimated on the basis of burn size may not meet normal daily metabolic requirements. In such patients, maintenance fluids should be added to the resuscitation regimen.
A “Rule of Ten” has recently been proposed as a simplified means of estimating the initial fluid infusion rate for adult burn patients and has been compared with existing formulae.
Rule of Ten.
- Estimate burn extent to the nearest 10% of the TBSA
- Extent of burns as estimated × 10 = initial infusion rate in mL per hour (for adults of 40–80 kg).
- Increase rate by 100 mL per hour for each 10 kg body weight >80 kg.
In 100,000 simulations evaluated in silico, 88% of the initial infusion rates calculated by the Rule of Ten fell between those calculated by the Modified Brooke Formula (MB) and the Parkland (PL) Formula. The majority of the calculated rates clustered about the MB infusion rate.47
The infusion rate is adjusted according to the individual patient’s response to the injury and the resuscitation regimen. The high circulating levels of catecholamines evoked by the burn and the progressive edema formation in burned and even unburned limbs commonly make measurements of pulse rate, pulse quality, and even blood pressure difficult and unreliable as indices of resuscitation adequacy. Since the hourly urinary output is a generally reliable and readily available index of resuscitation adequacy, an in-dwelling urethral catheter should be placed and the urinary output measured and recorded each hour. The fluid infusion rate is adjusted to obtain 30–50 mL of urine per hour in the adult and 1 ml per kg body weight per hour in children weighing <30 kg. To avoid excessive fluctuation of the infusion rate, the administration of fluid is increased or decreased only if the hourly urinary output is one-third or below or more 25% or more above the target level for 2 or 3 successive hours. If in either adults or children the resuscitation volume infused in the first 12 hours to achieve the desired urinary output or other indices of resuscitation adequacy exceeds estimated needs by more than twofold or will result in administration of six or more mL per percent body surface area burned per kg body weight in the first 24 hours, human albumin diluted to a physiologic concentration in normal saline should be infused and the volume of crystalloid solution reduced by a comparable amount. Inasmuch as functional capillary integrity is gradually restored during the first 24 hours postburn, such use of colloid-containing fluid is best reserved for the latter half of the first postburn day.
Restoration of functional capillary integrity and the establishment of a new transvascular equilibrium across the burn wound are manifested by the fact that both protein and water content of the burn-injured tissue reach maxima at or near 24 hours after injury.18 Consequently, the volume of fluid needed for the second 24 hours postburn is less and colloid-containing fluids can be infused to reduce further volume and salt loading. Human albumin diluted to physiologic concentration in normal saline is the colloid-containing solution infused in a dosage of 0.3 mL per percent burn per kg body weight for patients with 30%-50% burns, 0.4 mL per percent burn per kg body weight for patients with 50%-70% burns, and 0.5 mL per percent burn per kg body weight for patients whose burns exceed 70% of the TBSA. Electrolyte-free, 5% glucose in water is also given in the amount necessary to maintain an adequate urinary output. The colloid-containing fluids for the second 24 hours for burned children are estimated according to the same formula, but half-normal saline is infused to maintain urinary output and avoid inducing physiologically substantive hyponatremia by infusion of large volumes of electrolyte-free fluid into the relatively small intravascular and interstitial volume of the child.
During the second 24 hours after injury, fluid infusion “weaning” should be initiated to minimize further volume loading. In a patient who is assessed to be adequately resuscitated, the volume of fluid infused per hour should be arbitrarily decreased by 25%-50%. If urinary output falls below target level, the prior infusion rate should be resumed. If urinary output remains adequate, the reduced infusion rate should be maintained over the next 3 hours at which time another similar fractional reduction of fluid infusion rate should be made. This decremental process will establish the minimum infusion rate that maintains resuscitation adequacy in the second postburn day.
Escharotomy
As resuscitation proceeds and edema forms beneath the inelastic eschar of encircling full thickness burns of a limb, blood flow to underlying and distal unburned tissue may be compromised. Circulatory compromise can occur in limbs with mixed depth partial thickness burns and on occasion in limbs with less than completely circumferential burns. Edema and coolness of distal unburned skin on a burned limb are normal accompaniments of the injury and are not indicative of circulatory compromise requiring surgical release. Cyanosis of distal unburned skin and progressive parasthesias, particularly unrelenting deep tissue pain, which are the most reliable clinical signs of impaired circulation, may become evident only after relatively long periods of relative or absolute ischemia. Those limitations can be overcome by scheduled (q1–2 h) monitoring of the pulse signal in the palmar arch vessels and the posterior tibial artery using an ultrasonic flowmeter. In an adequately resuscitated patient, absence of pulsatile flow or progressive diminution of the pulse signal on repetitive examinations is an indication for escharotomy.
Since the full thickness eschar is insensate, an escharotomy can be performed as a bedside procedure without anesthesia using a scalpel or an electrocautery device. On an extremity, the escharotomy incision, which is carried only through the eschar and the immediately subjacent superficial fascia, is placed in the midlateral line and must extend from the upper to the lower limit of the burn wound (Fig. 32.5). The circulatory status of the limb should then be reassessed. If that escharotomy has not restored distal flow, another escharotomy should be placed in the midmedial line of the involved limb. A fasciotomy may be needed when there has been a delay in restoring the patient’s limb circulation and in particular if the patient is receiving a massive fluid load. Mistakes in the performance of escharotomies include injuries to extensor tendons and digital neurovascular bundles, insufficient depth and length of the incision, and delay in performing the escharotomy. Additionally, delayed bleeding from previously thrombosed vessels transected during the escharotomy should be promptly controlled. Continuous elevation and active exercise of a burned extremity for 5 minutes every hour limits edema formation and may eliminate the need for escharotomy.48
Edema formation beneath encircling full thickness truncal burns can restrict the respiratory excursion of the chest wall. If the limitation of chest wall motion is associated with hypoxia and progressive increase in the work of breathing, and peak inspiratory pressure as well, escharotomy is indicated to restore chest wall motion and improve ventilation. These escharotomy incisions are placed in the anterior axillary line bilaterally, and if the eschar extends onto the abdominal wall, the anterior axillary line incisions are joined by a costal margin escharotomy incision (Fig. 32.5). Rarely, encircling full thickness burns of the neck or penis will require release by placement of an escharotomy incision in the midlateral line(s) of the neck or the dorsum of the penis.
FIGURE 32.5. The dashed lines indicate the preferred sites of escharotomy of circumferential burns of the limbs, trunk, and other body parts. The thickened areas on those lines denote the importance of extending the escharotomy incisions across involved joints.
Post Resuscitation Fluid Therapy
Fluid management after the first 48 hours postburn should permit excretion of the retained fraction of the water and salt loads infused to achieve resuscitation, prevent dehydration and electrolyte abnormalities, and allow the patient to return to preburn weight by the 8th to 10th postburn day.41 Infusion of the large volumes of lactated Ringers required for resuscitation commonly produces a weight gain of 20% or more and a reduction of serum sodium concentration to approximate that of lactated Ringers, that is, 130 mEq/L. Such patients do not need additional sodium and, in fact, have an elevated total body sodium mass in association with increased total body water. Correction of that relative hyponatremia is facilitated by the prodigious evaporative water loss from the surface of the burn wound, which is the major component of the markedly increased insensible water loss that is present following resuscitation. Insensible water loss, which must be taken into account in postresuscitation fluid management, can be estimated according to the formula:
Insensible water loss (mL per hour)
= (25 + % TBSAB) × TBSA in square meters
where TBSAB= Total Body Surface Area Burn, and TBSA= Total Body Surface Area.49
That water loss should be replaced only to the extent that will permit a daily loss of 2%-2.5% of the resuscitation-associated weight gain (as measured 48 hours after the burn) until preburn weight is attained. Inadequate replacement of insensible water loss makes hypernatremia the most commonly encountered electrolyte disturbance in the extensively burned patient following resuscitation. Such hypernatremia should be managed by provision of sufficient electrolyte-free water to allow excretion of the increased total body sodium mass and replace insensible water loss to the extent needed to prevent hypovolemia.
During resuscitation, hyperkalemia is the most frequently encountered electrolyte disturbance and is typically a laboratory sign of hemolysis and muscle destruction by high voltage electric injury or a particularly deep thermal burn. Hyperkalemia may also occur in association with acidosis in patients who are grossly underresuscitated. Hyperkalemia in the burn patient is treated as in any other surgical patient. In the case of patients with high voltage electric injury, emergency debridement of nonviable tissue and even amputation may be necessary to remove the source of the potassium. Hypokalemia may occur after the resuscitation period in association with alkalosis as a consequence of hyperventilation and may also accompany postresuscitation muscle wasting. Potassium losses are increased by the kaluretic effect of the mafenide acetate in Sulfamylon burn cream and, as noted below, by transeschar leaching in patients treated with 0.5% silver nitrate soaks.
Major depression of ionized calcium levels is uncommon, but total calcium levels may be depressed if calcium-binding proteins such as albumin decrease as may happen in patients receiving a high volume of resuscitation fluid. In extensively burned children, hypocalcemia has been associated with hypoparathyroidism and renal resistance to parathyroid hormone.50 Symptomatic acute hypocalcemia should be treated with intravenous calcium (90–180 mg of calcium infused over 5–10 minutes) to control cardiac dysfunction and neuromuscular hyperactivity. Prolonged administration of parenteral nutrition and/or failure to supply sufficient phosphate to meet the needs of tissue anabolism following wound closure may cause hypophosphatemia. Administration of large volumes of antacids for stress ulcer prophylaxis is an infrequent cause of hypophosphatemia now that acid secretion inhibitors are used for that purpose. Hypophosphatemia can be prevented and treated by appropriate dietary phosphate supplementation.
The timely administration of adequate fluid as detailed above has essentially eliminated acute renal failure as a complication of inadequate resuscitation of burn patients. In a recent 10-year period, only 2 out of 2,132 burn patients treated at the U. S. Army Burn Center developed early renal failure, and those patients had established anuria when they were received in transfer from other institutions.51 Far more common today are the complications of excessive resuscitation, that is, compartment syndromes and pulmonary compromise (Table 32.5). Compartment syndromes can be produced in the calvarium, muscle compartments beneath the investing fascia, and the abdominal cavity. Cerebral edema, which may efface the epidural space and compress the ventricular system, is most apt to occur in burned children and is manifested by obtundation and changes evident on computerized tomographic scans. Such changes should be addressed by maintaining cerebral perfusion pressure and minimizing further edema formation by reducing fluid infusion rate and inducing diuresis. Anterior ischemic optic neuropathy manifested by visual field defects and even blindness, is another manifestation of excessive fluid infusion. This complication has typically occurred in association with other compartment syndromes and anasarca in critically ill patients, particularly in those who are nursed in a prone position. Consequently, prone positioning should be avoided in severely injured, critically ill burn patients requiring large volumes of parenteral fluids. The occurrence of visual field defects should prompt alteration of fluid therapy and induction of a diuresis.52
TABLE 32.5
CONSEQUENCES OF EXCESS RESUSCITATION
Excessive fluid administration may also cause formation of enough ascitic fluid and edema of the abdominal contents to produce intra-abdominal hypertension. The abdominal compartment syndrome represents progression of intra-abdominal hypertension to the point of organ dysfunction, that is, typically oliguria, and/or altered pulmonary mechanics. Infusion of resuscitation fluid volumes in excess of 25% of body weight has been associated with a high incidence of abdominal compartment syndrome.53 In all patients who have received that volume of resuscitation fluid, hourly monitoring of peak inspiratory pressure and intracystic pressure should be instituted. Elevation of peak inspiratory pressure above 40 mm Hg and/or elevation of intracystic pressure above 25 mm of mercury, as measured through a urethral catheter, should prompt therapeutic intervention beginning with adequate sedation, reduction of fluid infusion rate, diuresis, and paracentesis. If organ failure becomes evident, a midline abdominal incision should be made to reduce the elevated intra-abdominal pressure. That incision can be temporarily closed with a polyethylene “bag,” or a vacuum-assisted closure device. The abdomen can be definitively closed as soon as visceral edema resolves.
Compartment syndromes may also occur in the muscle compartments underlying the investing fascia of the limbs of burn patients, even in limbs that are unburned. To assess compartment pressure, the turgor of the muscle compartments should be assessed on a scheduled basis by simple palpation. A stony hard compartment is an ominous finding that should prompt further evaluation. The adequacy of arterial flow in all limbs of a burn patient should be monitored on a scheduled basis using the ultrasonic flowmeter. Ultrasonic detection of pulsatile flow is reassuring but the ultrasonic flow signals can be misleading since flow in large vessels may be maintained even though microcirculatory flow is severely compromised within a muscle compartment. Direct measurement of intracompartmental pressure using either a wick or needle catheter is much more reliable. A muscle compartment pressure of 25 mm of mercury or more necessitates performing a fasciotomy of the involved compartment in the operating room using general anesthesia.
Edema causing airway obstruction, in the absence of inhalation injury, has also been attributed to excessive resuscitation fluid. This complication, which necessitates endotracheal intubation, has been of particular concern in small children with extensive scald burns who have received in excess of 6 mL of lactated Ringers per percent body surface area burned per kilogram body weight. Pulmonary edema is rare during the initial 48-hour resuscitation period, but may occur in patients with extensive burns following resuscitation when the edema fluid is being resorbed and the protective early postburn pulmonary vascular changes have dissipated.
Mackie et al. have recently suggested that the application of mechanical ventilation alters postburn fluid dynamics and complicates fluid management by increasing net fluid balance independent of the presence of inhalation injury. Those investigators recorded a significantly greater cumulative fluid balance on the third and seventh postburn days in 186 patients with burns of more than 20% of the body surface who received mechanical ventilation as compared to that in patients who did not require mechanical ventilation.54 Careful monitoring of intake and output in such patients is mandatory. The treatment of pulmonary edema in the burn patient is the same as for other patients.
A closed loop computerized system to adjust resuscitation fluid infusion rate on the basis of urinary output measured at a scheduled frequency, for example, each hour or as recently proposed every 10 minutes has also been evaluated as a means of reducing resuscitation volume and decreasing the incidence of compartment syndromes. When the system was employed in patients with massive burns, the investigators reported better maintenance of the urinary output target and that the prompt response to minute systemic changes resulted in a lower total infused volume and net fluid balance.55
Pathophysiologic studies have also identified pharmacologic agents and physiologic mechanisms that may reduce resuscitation fluid needs. In studies of a murine model of a 30% burn, a threefold increase in intestinal myosin light chain kinase (MLCK) was observed 4 hours after burn injury in association with a marked (fivefold) increase in intestinal permeability.56 Postburn administration of pentoxifylline decreased the burn-induced phosphorylation of intracellular kinases and was associated with a decrease in histologic evidence of gut injury at 6 hours postburn and lung injury at 24 hours postburn.57 The authors concluded that pentoxifylline attenuated the activation of the tight junction protein (MLCK) as a consequence of its reduction in tumor necrosis factor—alpha synthesis and nuclear factor kappaB activation after burn injury. Because of its anti-inflammatory effects, pentoxifylline has been proposed as an immunomodulatory additive of resuscitation fluid. Recently, that same group of investigators has identified a protective effect of vagal nerve stimulation on intestinal barrier integrity in a murine model of severe burn injury. Vagal stimulation was found to reduce the postinjury increase in intestinal permeability, decrease histologic changes in the intestinal mucosa, and prevent loss of occludin localization at points of intestinal epithelial cell-to-cell contact.58
VENTILATORY SUPPORT AND TREATMENT OF INHALATION INJURY
Pathophysiology
Patients suffering both inhalation injury and thermal burns have a considerably increased incidence of complications and probability of death. While an inhalation injury alone carries a mortality of 5%-8%, a combination of a thermal injury plus inhalation injury can easily result in a mortality 20% above that predicted on the basis of age and burn size.59 Patients who have an otherwise survivable injury may succumb to their burn as a consequence of their inhalation injury and the complications that occur, particularly a gram negative pneumonia.60 Injuries to the airway are most often due to direct damage by inhaled products of combustion or pyrolysis that cause inflammation and edema. Damage to the airway, while in part related to the heat content of the inhaled material in the oropharynx is, in the more distal airways, principally related to the particulate material contained within the smoke and the chemical composition of inhaled materials. Moist heat, which occurs with steam, has 4,000 times the heat-carrying capacity of dry smoke and is capable of causing more extensive thermal damage of the tracheobronchial tree.61
Presenting patient signs and symptoms are stridor, hypoxia, and respiratory distress.62 The probability that a patient has suffered an inhalation injury is highly correlated with being burned in an enclosed space, having burns of the head and neck, and having elevated carbon monoxide levels. The extent and severity of the inhalation injury are directly related to the duration of exposure and the various toxins contained within the smoke. Injury due to heat is typically confined to the upper airway and supraglottic structures.63 Particulate material within smoke is the vehicle by which the toxic materials are carried to the distal airway.
Particles of <5 µ in size can reach the terminal bronchi and the alveoli. Upper airway injuries involve the mucous membranes, nasopharynx, hypopharynx, epiglottis, glottis, and larynx. The lining mucous membranes as well as the cartilage of the glottis are easily damaged and can cause acute airway compromise.63 Direct thermal injury to the lower airway is uncommon as rapid dissipation of heat occurs as the gases move through the upper airway. Injury to the tracheobronchial structures and pulmonary parenchyma is related to the toxins in the inhaled smoke and the ensuing host inflammatory response. Activation of the inflammatory cascade results in the recruitment of neutrophils and macrophages that propagate the injury.64 Cellular damage is perpetuated by those cells that further the inflammatory response, which in turn leads to progressive pulmonary dysfunction.65 Altered surfactant release causes obstruction and collapse of distal airway segments.66 As part of the response to injury there is a marked and near immediate change in bronchial artery blood flow that can increase by up to twentyfold.64 These changes in bronchial blood flow are also associated with marked alterations in vascular permeability within the lung and are thought to play an important role in the pathophysiologic response to inhalation injuries.67 The net effect is that extensive destruction and inflammation reduce pulmonary compliance and impair gas exchange resulting in altered pulmonary blood flow patterns and ventilation perfusion mismatches.68,69
Asphyxiants
Carbon monoxide and cyanide gases are present in smoke and when inhaled are rapidly absorbed and cause systemic toxicity as well as impaired oxygen utilization and delivery. Carbon monoxide is an odorless, nonirritating gas that rapidly diffuses into the blood stream and rapidly binds to the iron moiety of the hemoglobin molecule. Carbon monoxide has a 240 times greater affinity for hemoglobin than does oxygen; thus it easily displaces oxygen. Carbon monoxide directly impairs the ability of hemoglobin to deliver oxygen to the tissues. Carbon monoxide also binds to enzymes within the mitochondria involved in intracellular oxygen utilization and cellular energetics.70 Signs and symptoms of carbon monoxide poisoning are typically mild to none at all when carbon monoxide–hemoglobin (carboxyhemoglobin) levels are 10% or less. When carboxyhemoglobin levels are between 10% and 30%, symptoms are present and often manifested by headache and dizziness. Severe poisoning is seen in patients with carboxyhemoglobin levels of >50% that may be associated with syncope, seizures, and coma.
The diagnosis of carbon monoxide poisoning is made in a patient with burns on the basis of circumstances of injury, physical findings, and the measurement of blood carboxyhemoglobin level. It is important to note that pulse oximetry values do not differentiate between carboxyhemoglobin and oxyhemoglobin. Patients with appreciable carbon monoxide intoxication can have elevated oxygen saturations but will not have satisfactory blood oxygen contents. The primary treatment modality for carbon monoxide intoxication is provision of increased levels of inspired oxygen. The carbon monoxide “half-life” will decrease from 6 to 8 hours with room air to 40–80 minutes with 100% of FiO2. Administration of oxygen in a hyperbaric chamber can further decrease the “half-life” to 15–30 minutes.71 In a recently reported randomized trial, Weaver et al.72 found that hyperbaric oxygen therapy significantly benefited patients with acute carbon monoxide poisoning. The utility of this in patients suffering major burns in association with carbon monoxide poisoning is yet to be demonstrated. It is an important practical question whether a patient can safely undergo treatment with hyperbaric oxygen therapy when there are other life-saving treatments that are needed. An approach that has worked well is to maintain the patient on 100% FiO2 until carboxyhemoglobin levels are <15% and then to maintain this level of increased oxygen for an additional 6 hours at which time weaning of the FiO2 can be initiated and conducted in accordance with standard criteria.
Cyanide poisoning, which has been considered uncommon except in combination with carbon monoxide intoxication, further disrupts normal cellular utilization of oxygen by binding to the cytochrome oxidase resulting in cellular lactic acid production and greater cellular dysfunction. Blood concentrations of cyanide >0.5 mg/L are toxic. Treatment of cyanide poisoning includes the administration of oxygen as well as decontaminating agents such as amyl and sodium nitrates. These compounds induce the formation of methemoglobin, which can act as a scavenger of cyanide.73 Sodium thiosulphate, which can be administered intravenously, enhances the enzymatic detoxification of cyanide to thiocyanate but acts slowly. Hydroxycobalamin, which acts more rapidly and has few side effects, is the antidote of choice. The ready availability of cyanide antidote kits containing amyl nitrite pearls, 10% sodium nitrite, and 25% sodium thiosulfate or Cyanokits containing 5 g of hydroxycobalamin and their frequent use by emergency medical personnel must alert emergency department and burn physicians to the possibility that the patient has received such treatment in the field.74
Smoke may also contain a variety of toxic compounds that cause or initiate further damage to the airway (Table 32.6). The composition of each fire is different adding to the difficulty in caring for these patients. Such additional products in smoke include acrolein, hydrogen chloride, phosgene, ammonia, nitric oxide, and sulfur dioxide all of which are capable of causing substantial injury.
TABLE 32.6
TOXIC AGENTS IN SMOKE
Airway Management
The most critical factor in the initial assessment of a burn patient is the patency of the airway and the ability of the patient to maintain and protect the airway. Standard criteria should be used to determine the need for mechanical stabilization of the airway also keeping in mind the systemic response to a major burn and the local response to an airway injury that may combine to cause progressive airway swelling and edema that will impair airflow. In an adult trachea of 14 mm, 1 mm of edema will result in a 25% reduction in cross sectional area. A similar degree of swelling in a 6 mm trachea of a child will result in greater than a 50% reduction in cross sectional area. Circumferential torso burns will further impair the ability of the patient to respire. Allowing airway compromise to proceed to a critical state before intubating the patient and stabilizing the airway is not appropriate care. The safest approach when there is concern about the airway, particularly in a patient needing transport for definitive care, is to perform early intubation.
Part of the initial management of the patient with inhalation injury should include a thorough evaluation of the airway including bronchoscopy. The clinical findings of an inhalation injury on bronchoscopy include airway edema, inflammation, increased bronchial secretions, presence of carbonaceous material that can diffusely carpet the airway, mucosal ulcerations and even endoluminal obliteration due to sloughing mucosa, mucous plugging, and cast formation (Fig. 32.6). Signs of gastric aspiration may also be evident. Bronchoscopy can be performed repeatedly for removal of debris and casts as well as surveillance for infection.
FIGURE 32.6. A bronchoscopic view of the airway in a patient with inhalation injury. Note the extensive deposition of carbonaceous material on the bronchial mucosa. Note also the erythematous areas of mucosal inflammation and pale focal areas of mucosal ulceration.
Direct airway treatment has been attempted with variable response. Desai et al. conducted an open label trial of the use of aerosolized heparin and N -acetylcysteine in children with inhalation injuries. The treated patients had an improved outcome (lesser incidence of atelectasis, decreased reintubation rate, and reduced mortality) compared to an untreated cohort.75 A randomized prospective study has not yet been performed to confirm the utility of this approach particularly in adults. One noticeable effect with the empiric use of aerosolized heparin is the rapid clearing of particulate material and carbonaceous deposits from the airway. Laboratory studies have shown that the effectiveness of aerosolized heparin was increased when combined with intravenous administration of lisofylline. The synergistic effect of that antiinflammantory agent supports clinical evaluation of such combination therapy.76 Inasmuch as the development of pneumonia in patients with inhalation injuries negatively impacts outcome, it is disappointing that prophylactic antibiotics have not been effective.77 As a practical matter, it is best to culture these patients early to identify the organisms that have colonized their airways as a consequence of their injury and urgent airway manipulation. That information can guide therapy should the patient develop the signs and symptoms of early onset pneumonia. Steroids are not recommended in patients with inhalation injuries.78
Mechanical Ventilation
The critical feature in the management of patients suffering inhalation injuries is to minimize further damage to the airway and lung parenchyma while providing adequate gas exchange.79 A major emphasis in the management of patients with inhalation injuries is to control airway pressures and thereby limit ventilation-induced barotraumas.62 It is important to recognize that lung damage is not homogenous but patchy in distribution and requires that the level of positive end expiratory pressure (PEEP) used to maximize airway recruitment be limited to avoid ventilator-associated lung injury.80,81 In states of severe lung injury, mechanical ventilation can lead to increases in sheer forces and changes in pulmonary blood flow. This in association with reductions in elasticity and alterations in lung compliance results in further lung injury and ventilation perfusion abnormalities.82,83
Using a standard volume mode of ventilation does not represent the best management of the damaged airway. Inverse ratio ventilation provides a strategy one can use in an attempt to counteract these changes and allow reductions in the level of PEEP and respiratory pressures to improve oxygenation.84 Unfortunately, a clear advantage of inverse ratio ventilation over standard approaches has not been consistently shown.85
High-frequency ventilation is widely utilized at present in the form of high-frequency percussive ventilation (HFPV). High-frequency interrupted flow positive pressure ventilation delivers small tidal volumes (4 mL/kg) at flow rates of 250 liters per minutes with a frequency of 100 breaths per minute. In this mode, expiration is passive and thus there is an increased risk of air trapping and over distention. Allan et al.86 recently reviewed and compared the use of HFPV using the VDR-4, (a volumetric diffusive respirator), high-frequency oscillatory ventilation, and high-frequency jet ventilation. Those investigators considered that HFPV in comparison to conventional ventilation improved oxygen and carbon dioxide tensions, attenuated lung inflammation and histologic lung injury, and improved static lung compliance, ventilation, oxygenation index, and the oxygen tension/fraction of inspired oxygen. That form of ventilation, which was also associated with a decrease in ventilator-associated pneumonia and an increase in survival of inhalation injury patients, was considered to have the unique capacity to exploit both high- and low-frequency ventilation to favorably influence gas exchange in a lung protective low tidal volume ventilation strategy.
There has been limited experience with the use of extracorporeal membrane oxygenation in the management of inhalation injuries in selected centers.87 A carbon dioxide removal device has been developed to provide limited extracorporeal support to patients with chronic obstructive pulmonary disease. Investigators have reported that removal of carbon dioxide by that device permitted reduction in a patient’s minute ventilation to one-third of baseline values.88 The device has been proposed as a treatment modality for patients with pulmonary failure as a consequence of inhalation injury.
An approach that has worked well in many patients with inhalation injuries has been to identify promptly the presence of airway compromise and ensure that the patient is intubated with a properly sized endotracheal tube. Currently, it is our preference in the pediatric population to use a cuffed tube that may need to be inflated to achieve maximum ventilatory efficiency. In patients who have signs of inhalation injury on bronchoscopy, there is aggressive management of retained secretions with the use of bronchodilators and mucolytic agents along with aerosolized heparin. Meticulous control of airway pressure is practiced with the early performance of torso escharotomies and prompt treatment of an abdominal compartment syndrome particularly in the burned child. Mean airway pressures are maintained at <32–34 cm of water and ready use is made of chemical paralysis of the patient with a low threshold for conversion to pressure-controlled ventilation with titration of tidal volumes to lessen further the risk of ventilator-associated barotrauma. This may require the acceptance of smaller than usual tidal volumes and permissive hypercapnia, which is acceptable as long as arterial blood pH is above 7.26 and the patient is hemodynamically stable. These approaches along with a tightly controlled fluid resuscitation will in most circumstances avoid the need for alternative ventilator strategies in the care of these patients. Others including the senior author prefer to use HFPV prophylactically. In all patients with documented inhalation injury, HFPV is initiated on admission to minimize airway obstruction, maintain lung volume, and reduce the risk of pneumonia.
Early extubation and cessation of mechanical ventilation in patients with inhalation injury reduce the incidence of ventilator-associated pneumonia and limit barotrauma. The use of sedation “holidays” and spontaneous breathing trials enable one to assess pulmonary reserve and predict extubation success, but the results can be misleading. White and associates at the U.S. Army Burn Center evaluated interbreath interval complexity during spontaneous breathing trials as a means of predicting extubation success and reducing extubation failure.89 Those investigators identified that lower interbreath interval complexity was associated with extubation failure. Analysis of wave form data identified lower sample entropy in the failure group with other nonlinear metrics moving in concert with entropy.
OTHER ORGAN SYSTEM SUPPORT
Pain Control
The pain experienced by patients suffering from acute thermal injuries is a complex integration of the objective neurologic input from the damaged tissue and the patient’s fear and anxiety resulting from the traumatic event. The patient’s pain is compounded further by wound care and the therapy required to maintain functional status. If the patient begins to perceive that there is no escape from this situation, fear and anxiety will be amplified and may be magnified further by the appearance and expression of concern by family and friends. Furthermore, as the burn begins to heal, there may be increased wound sensitivity during dressing changes and therapy sessions. This can further distress patients, as they perceive that instead of the pain improving with recovery it seems to be worsening. Pain is a fifth vital sign and it should be monitored, its level documented, and treatment be properly planned. Appropriate therapeutic options must be available to provide pain control to patients.
In patients who are hospitalized for ongoing care or require surgery, it is best to initiate long acting oral narcotic agents for background pain control and administer shorter acting narcotics either orally or parenterally, along with anxiolytic agents, for procedure related pain. For an acutely injured patient undergoing a procedure in the ICU or ward, intravenous morphine remains the main stay for analgesia along with oral compounds such as hydrocodone, which is preferable to oxycodone, which has a greater propensity for abuse. Clonidine can also be added to the pain regimen in patients who are having an initial poor response to narcotics and anxiolytic agents.90 In patients who are intubated and are being maintained on mechanical ventilation, the two commonly used regimens are the continuous administration of intravenous morphine and diazepam or propofol and fentanyl to achieve pain control and sedation and prevent unplanned extubation. In the postoperative care of patients having skin graft procedures, the most painful wound is often not the burn wound but the donor site. Jellish et al.91 have reported that the treatment of the skin graft harvest site with local anesthetic agents significantly improved the patient’s pain score postoperatively. Patient-controlled analgesia is also a very effective strategy in patients who understand and can manipulate the delivery system. An important factor leading to effective pain control is to have established protocols that all members of the team understand and can use safely. There must be flexibility in the medication regimen and the patients must be fully informed that every attempt will be made to provide them the greatest comfort within the context of safe and compassionate care.
Neurological Deficits
Immediately following burn injury, the patient with an altered mental status needs to undergo a careful evaluation for injuries occurring during and prior to the time of the fire. Additionally, the patient’s preinjury neurological status needs to be determined for any prior impairment. In patients who are obtunded, the primary concern is central nervous system (CNS) injury due to hypoxia and carbon monoxide poisoning. The use of alcohol or drugs in the time leading up to the burn injury can confound the assessment of the burn-injured victim. Testing for alcohol and drugs aids in the evaluation of such patients.92 This is particularly important since the impact of alcohol appears to substantially modify the patient’s chance for survival. The patient, particularly an elderly patient, should also be evaluated for a primary CNS event that might have precipitated their injury such as a seizure, stroke, or intracranial hemorrhage. The possibly of an assault must always be foremost in one’s thoughts particularly in children when the history of the injury does not match the findings. It is not uncommon to find that a burn is a signal finding for child abuse.93 This can also be the case in adults where the initial event was an assault and the burn is an attempt to disguise the physical attack.
Patients presenting with agitation must be rapidly evaluated for hypoxia and treated. The initial management of a patient who has a deteriorating mental status is a review of the medications and medication doses that have been administered to determine whether reversal agents should be given. In the treatment of children, the doses should be age and weight appropriate and carefully titrated to the patient’s need. Later in the course of a burn patient’s care, changes in mental status require a detailed review of medications for pain and sedation, measurement of serum electrolyte values particularly sodium, and evaluation for a septic process and the onset of renal or hepatic failure. Patients with impaired mental status either early or late must always be assessed for the status of their airway, their ability to maintain a patent airway, and the need for tracheal intubation.
Gastrointestinal Responses and Complications
Impaired GI motility and focal gastric mucosal ischemia occur in virtually all patients with burns involving more than 25% of the total body surface with the severity of change proportional to the extent of the burn.94 The resulting ileus necessitates nasogastric intubation to prevent emesis and aspiration. If the mucosa is unprotected by instillation of antacid or treatment with an H2 histamine receptor antagonist, ischemic erosions in the mucosa may progress to frank ulceration with associated bleeding or even perforation. Sufficient antacid (typically 30 mL but 60 mL may be needed) should be instilled each hour to maintain the pH of the gastric contents above 5. At the present, a histamine H2 receptor antagonist (e.g., 400 mg of cimetadine given intravenously every 4 hours) or proton pump inhibitors are more commonly used for stress ulcer prophylaxis. When GI motility returns, enteral feeding should be initiated and the antacid therapy, for example, a 1,200–2,400 mg daily dose of cimetadine, can be added to enteral feeds. A randomized study comparing antacid prophylaxis and nonacid buffering sucralfate prophylaxis showed no difference in recovery of gram-negative organisms from the upper GI tract, and no difference in the occurrence of gram-negative pneumonia, but lesser gastric mucosal protection and a higher incidence of gram positive pneumonia in the sucralfate-treated group.95
WOUND CARE
Initial Wound Care
Initial wound care is focused on preventing further injury. Immediately upon removal of the burn victim from the site of injury, attention should be given to removal of burning clothing, disrupting contact with metal objects that may retain heat, and cooling of any molten materials adherent to the skin surface. Attempted cooling of burn wounds must be done with caution as local vasoconstriction can impair wound blood flow and extend the depth of the injury. The use of surface cooling of the burn is limited to patients with small burns typically not requiring hospitalization. Hypothermia can rapidly occur in children as well as adults particularly elderly patients if they are placed in cool or wet dressings. Patients being prepared for transport or admitted for definitive care should be placed in sterile or clean dry dressings and be kept warm. Prolonged exposure of the burn victim’s wounds leads to cooling and further impairs the patient’s response to their injury. Items of clothing or jewelry that may impair circulation should be removed prior to the onset of burn wound edema to prevent further compromise of the circulation. In cases of chemical injury, removal of contaminated clothing and copious water lavage of liquid chemicals and removal by brushing of powdered materials at the scene can limit the extent of the resultant burn injury. The ability to perform these maneuvers at the scene must be balanced against the patient’s associated injuries that would mandate immediate transport for the care of life threatening injuries. No attempt should be made at chemical neutralization of the suspected chemical agent as such treatment would result in an exothermic reaction and cause additional tissue damage. The care provider must exercise extreme caution when working with victims of chemical injury to prevent self-contamination and personal injury. In all circumstances, those individuals caring for burn patients should wear personal protection devices. After admission to the hospital and as soon as resuscitative measures have been instituted, the patient should be bathed and the burn wounds cleansed with a detergent disinfectant. Chlorhexidine gluconate is a cleansing agent with an excellent antimicrobial spectrum.
During cleansing of the wound, the patient must not be allowed to become hypothermic. The treatment area and the cleansing fluids should be warm and the procedure should be done expeditiously. Materials that are densely adherent to the wound such as wax, tar, plastic, and metal should be gently removed or allowed to separate during the course of subsequent dressing changes. Sloughing skin, devitalized tissue, and ruptured blisters should be gently trimmed from the wound. No formal attempt is made to remove the burned tissue during these wound-dressing debridements. Patients may experience considerable pain and apprehension during these dressing changes and should receive adequate pain medication. In the patient on whom blisters are present, whether to remove them or allow them to remain is a matter of opinion. Blisters that are intact, particularly thick blisters on the palm of a hand maybe left intact.96 Intact blisters must be closely monitored for signs of infection or rupture at which time they should be debrided and the wound treated. If a blister can be kept intact wound healing should be complete in <3 weeks. The notion that intact blisters are an effective biologic dressing has been called into question and some authors recommend removing all blisters and treating the burn as an open wound.21 Careful wound cleansing should be done at each dressing change with serial debridement of devitalized tissue performed as necessary. The wound should be monitored for signs of infection and change in depth from the initial assessment. It is not uncommon for the initial wound depth to have been underestimated in children and young women. Additionally, the extent of body surface involvement should be recalculated to ensure that the initial burn size determination was accurate.
Topical Antimicrobial Therapy
The burn injury sets in progress a series of events leading to impaired local and systemic immunity. The damaged skin surface can serve as the portal for microbial invasion if it becomes progressively colonized. As microbial numbers increase within the wound to levels of 100,000 organisms per gram of tissue, an invasive wound infection and ultimately systemic sepsis may occur. Topically applied antimicrobial agents, which penetrate the burn eschar, are capable of achieving sufficient levels to control microbial proliferation within the wound. Systemic antibiotics do not achieve sufficiently high concentrations in the wound to achieve therapeutic levels, as the eschar is in large part avascular. These concepts form the basis for the use of topical antimicrobial agents in the prophylactic treatment of the burn wound and as a part of the management of burn wound infections.97 Topical agents per se do not heal the wound but prevent local burn wound infection from destroying viable tissue in wounds capable of spontaneous healing. In burns that will require excision and grafting, control of the burn wound microbial density prevents the development of systemic sepsis secondary to invasive burn wound infection. The utility of topical agents is most clearly apparent in improving patient outcome in burns of >30% TBSA. The need for the use of topical antimicrobial compounds in the management of small burns has never been demonstrated and these injuries can be effectively managed with a petroleum-based dressing.98–101 That being said, it has become common practice to apply topical antimicrobial agents to even small burn wounds despite the fact that there are no data to support this approach. Topical antimicrobial burn wound agents include cream, ointment, and liquid based products that require daily to twice daily dressing changes and reapplication. Also available are materials impregnated with antimicrobial compounds that typically are changed following a several day period of application.
Silver sulfadiazine, the most widely used agent, is available as a 1% suspension in a water-soluble micronized cream base. The cream is easily applied, causes little or no pain on application, and can be used open or as a closed dressing. As a closed dressing, the cream can be directly applied to the wound as a continuous layer, then covered over with a dressing or be impregnated into the dressing, which is then applied to the wound. At each dressing change, the cream should be totally removed and not allowed to form a caseous layer that will obscure the wound bed. When silver sulfadiazine is used in the management of superficial partial thickness burns, the dressing becomes discolored, the wound exudate appears infected, and the wound develops a yellow-gray pseudoeschar that is easily removed. The ability of silver sulfadiazine to penetrate an eschar and prevent wound infectious complication in burns >40%-50% TBSA is thought to be poor. However, wound cleansing with chlorhexidine as a part of the wound management along with silver sulfadiazine has proven to be a very effective combination in patients with larger burns. The most common toxic side effect of silver sulfadiazine is a transient leukopenia, which when it does occur in up to 15% of treated patients, resolves spontaneously without discontinuation of the drug.102 The proposed mechanisms for this response have ranged from leukocyte margination in the wound (not drug related) to a direct cytotoxic effect of the drug on the bone marrow granulocyte and macrophage progenitor cells.103 Silver sulfadiazine is active against a wide range of microbes including Staphylococcus aureus , Escherichia coli , Klebsiella species, many but not all Pseudomonas aeruginosa , Proteus species, and Candida albicans.
Mafenide acetate was one of the first effective topical agents introduced for the management of the burn wound. It was initially available as Sulfamylon burn cream (an 11.1% suspension in a vanishing cream base). It is commonly used on exposed wounds treated by the open technique though it is possible to use it under a light dressing. Mafenide acetate is highly effective against gram-positive and gram-negative organisms, but provides little antifungal activity.104 Mafenide acetate readily diffuses into the eschar and is the agent of choice for major burns of the ears because it is also capable of penetrating cartilage. Drawbacks with the use of mafenide acetate include pain on application to partial thickness burns, and limited activity against methicillin-resistant S. aureus. Mafenide acetate also inhibits carbonic anhydrase, which increases urinary loss of bicarbonate, which may cause hyperchloremic acidosis and accentuate postburn hyperventilation. Fortunately, this affect is time limited because the kidney typically escapes from such inhibition in 8–10 days. Mafenide acetate has more recently become available as a 5% aqueous solution and is an excellent agent to use on freshly grafted wounds and is not associated with the problems found with the cream formulation.
Silver nitrate as a 0.5% solution has also been available since the 1960s and is effective against gram-positive and gram-negative organisms. The silver moiety is deposited on the burn wound and does not penetrate the eschar to any great extent. Silver nitrate soak solution leaches sodium, potassium, chloride, and calcium from the wound in association with transeschar water absorption, which can result in mineral deficits, alkalosis, and water loading. Those side effects can be minimized by giving sodium and other mineral supplements and modifying fluid therapy. These problems and the labor required to use silver nitrate effectively limit its routine use currently and most see silver sulfadiazine as a highly acceptable alternative.
Silver impregnated dressings consisting of a polyethylene mesh coated with a nanocyrstalline film of pure silver ions bonded to a flexible rayon-polyester sheet have recently become available for clinical use.105 When the fabric base is in contact with wound fluids, the silver is released continuously to maintain a silver concentration of 50–100 mg/L on the wound for up to 48 hours. The treatment interval with such a composite may extend up to several days depending on the fabrication design with dressing changes needed only once or twice per week. On the basis of a multicenter randomized controlled trial it was concluded that Acticoat has better antimicrobial activity and fewer adverse effects than topical silver sulfadiazine, and reduces healing times.106 The investigators considered the ease of application and low frequency of change to make Acticoat an ideal dressing for burn wounds. However, one must know that the wound has not become compromised during extended periods between reapplication. It is not advisable to use this approach in the care of perineal wounds particularly in small children with frequent bowel movements or wounds with excessive drainage or difficulty in maintaining contact between the dressing and the burn wound surface. The effectiveness of this membrane in treating extensive full thickness burns is unconfirmed and at present it is used to treat partial thickness burns.
In superficial partial thickness burns, the use of bacitracin ointment represents a satisfactory alternative particularly in patients with a known sulfa allergy. It may be used open especially with superficial facial burns or as a component of a closed dressing. Other topical agents include antibiotic combinations such as triple antibiotic ointment (neomycin, bacitracin zinc, and polymyxin B) and polysporin (bacitracin zinc and polymyxin B). In the case of methicillin-resistant staphylococci, mupirocin represents a useful agent.107 These agents are also capable of being used with open or closed dressing techniques.
The application of topical antimicrobial agents to the burns of patients who will be transferred to a burn center may preclude the use of biological membrane dressings that will adhere to the wound surface to be effective. Additionally, upon admission to a burn center, any previously placed dressing will be removed to permit the burn team to make a precise assessment of the extent of the burn and the depth of injury. In addition to burn wound evaluation, the burned child must be inspected for any signs of abuse, which includes a detailed examination of the entire skin surface. Unless there will be an extended period of time before patient transfer to a burn center, placing the patient in a dry dressing, particularly if it is one with a nonadherent lining, and keeping the patient warm is the preferred initial management.
The sterile gloved hand is used to apply the burn cream of choice in a thickness sufficient to obscure the surface of the burn (Fig. 32.7). The wounds are then either left exposed or covered by a light occlusive dressing. Twelve hours later, the topical agent is reapplied to the entire burn wound. If a dressing is used over the topical agent, it is removed prior to reapplication of the topical agent following which a new dressing is applied. To optimize antimicrobial coverage and minimize side effects, one can alternate topical agents, that is, apply Sulfamylon burn cream after the morning cleansing and apply Silvadene burn cream in the evening.108 If 0.5% silver nitrate soaks are used for topical wound care (as may be necessary in a patient allergic to sulfonamides), they should be changed two or three times a day and kept moist between changes by infusing additional soak solution every 2 hours. All environmental surfaces and equipment as well as the clothing and exposed skin of attending personnel must be protected from contact with the silver nitrate solution, which will cause dark brown discoloration of virtually anything with which it comes in contact.
FIGURE 32.7. Following the initial cleansing and debridement, the burn wounds are covered with a topical antimicrobial burn cream as shown here. The topical agent is reapplied 12 hours later. The wounds are cleansed each day and thoroughly examined to identify signs of burn wound infection and if no such signs are evident the burn cream is reapplied.
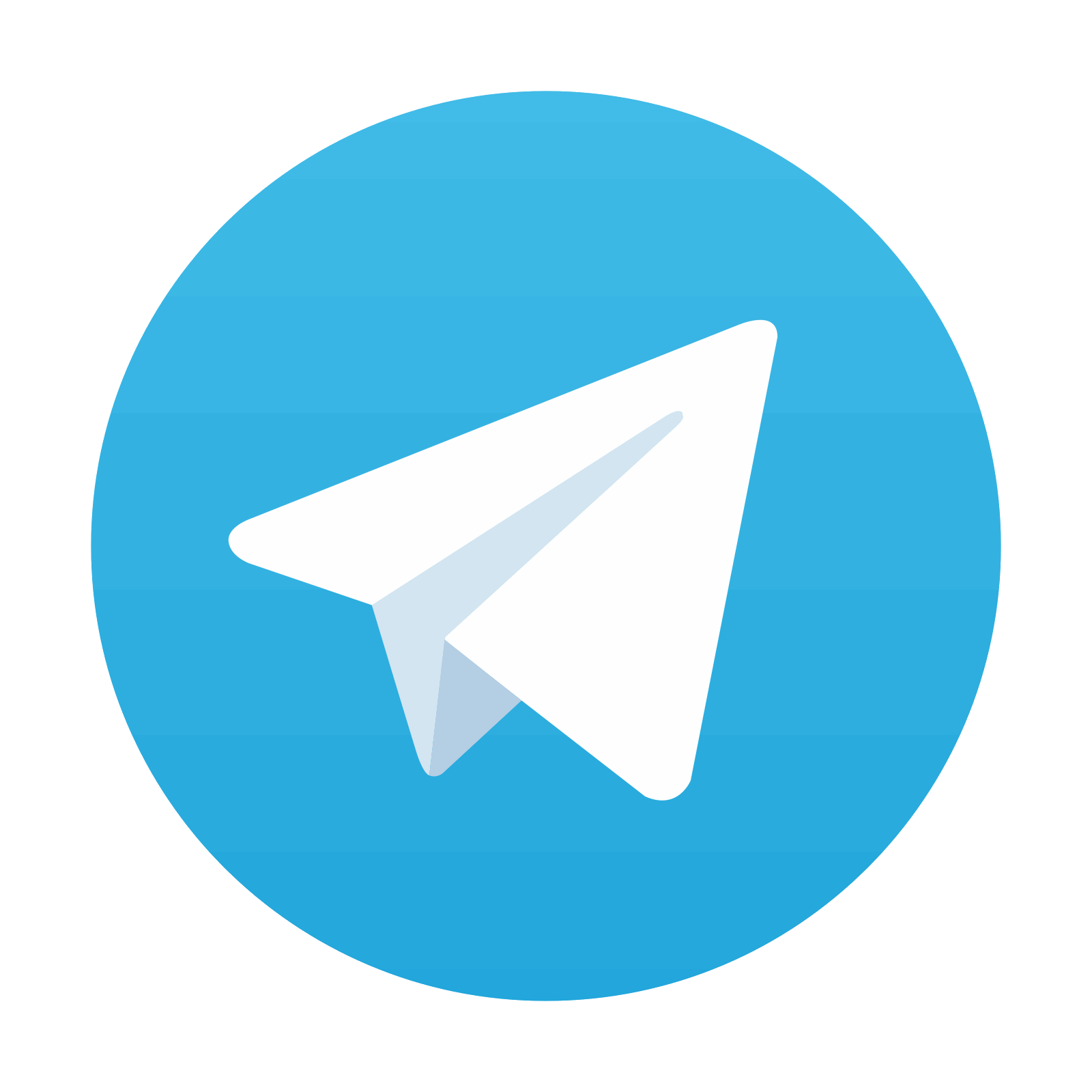
Stay updated, free articles. Join our Telegram channel

Full access? Get Clinical Tree
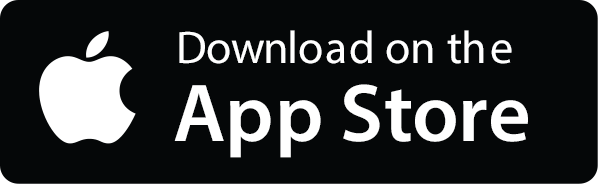
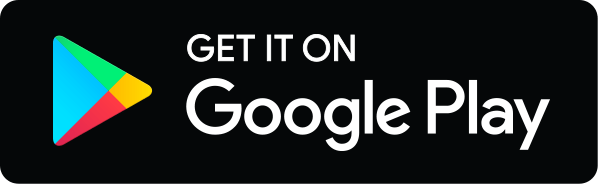