CHAPTER 13
ANTIBIOTIC USE AND MISUSE
Traditionally, surgeons were only involved with infections that required invasive measures for treatment (e.g., complicated intra-abdominal infections and skin/soft tissue infections [cSSTIs]). However, surgical patients are particularly vulnerable to nosocomial infections; therefore, the acute care surgeon must be concerned with the prevention and treatment of all infections that affect surgical patients, including surgical site infections (SSIs), central line–associated bloodstream infections (CLABSIs), urinary tract infections (UTIs), and hospital- or ventilator-associated pneumonia (HAP/VAP). Trauma patients are particularly vulnerable to infections of injured tissue as well as nosocomial infections related to environmental factors (e.g., hypothermia), host immunosuppression (e.g., inadequate glycemic control), and therapeutic interventions (e.g., multiple incisions and catheters, blood transfusion).1,2 The overall incidence of infection following trauma is estimated to be 25%–36; trauma patients may be twice as likely to become infected as critically ill general surgery patients.7 These disease states are discussed elsewhere in this volume; this chapter discusses the use and misuse of antibiotics for prophylaxis and therapy.
Considering that the development of a postoperative infection has a negative impact on surgical outcomes, recognizing and minimizing risk and an aggressive approach to the diagnosis and treatment of such infections go hand in hand. Infection is preventable to some degree, and every acute care surgeon must do his or her utmost to prevent infection. An ensemble of tactics is required, because no single method, including antibiotic prophylaxis, is universally effective. Infection control is paramount. It must always be remembered that surgical illness and injury are immunosuppressive, as are many critical care therapeutics. Surgical incisions and traumatic wounds must be handled gently, inspected daily, and dressed if necessary using strict asepsis. Drains and catheters must be avoided if possible and removed as soon as practicable. Prophylactic and therapeutic antibiotics, whether empiric or directed against a known infection, must be used optimally so as to minimize antibiotic selection pressure on the development of multidrug-resistant (MDR) pathogens.
INFECTION CONTROL
General principles of surgical care, critical care, and infection control must be adhered to at all times. As but a few examples, resuscitation must be rapid, yet precise; both over- and underresuscitation increase the risk of infection. Pathology must be identified and treated as soon as possible. Central venous catheters inserted under suboptimal barrier precautions (i.e., lack of cap, mask, sterile gown, and sterile gloves for the operator and a full-bed drape for the patient) must be removed and replaced (if necessary) by a new puncture at a new site as soon as the patient’s condition permits. Drains should be avoided and removed as soon as possible if required.8 Detailed evidence-based guidelines for the general prevention of SSI,9 CLABSI,10,11 and VAP have been published.12,13 Hand hygiene is the most effective means to reduce the spread of infection, yet compliance is a continual challenge.14
Endogenous flora are the source of most human pathogens. Skin surfaces, artificial airways, gut lumen, wounds, catheters, and inanimate surfaces (e.g., bed rails, computer terminals) may become colonized.15 Any break in natural epithelial barriers (e.g., incisions, percutaneous catheters, airway or urinary catheters) creates a portal of entry for invasion of pathogens. The fecal–oral route is the most common manner by which pathogens reach the portal, but health care workers facilitate the transmission of pathogens on their hands.15 In this context, antibiotics should be considered an essential component, but not a panacea, and certainly not as a substitute for best practices in all aspects of patient care.
Unfortunately, selection pressure from long-term overuse of antibiotics is a major factor in the emergence of MDR pathogens such as methicillin-resistant Staphylococcus aureus (MRSA), vancomycin-resistant enterococci (VRE), and gram-negative bacilli (e.g., Klebsiella spp., Acinetobacter spp., Stenotrophomonas spp., and Pseudomonas aeruginosa)16–19 (Table 13.1) that have developed numerous mechanisms to express resistance to antibiotics. Fortunately, adherence to optimal prescribing practices, sometimes known as antimicrobial stewardship ,20 can improve the microbial ecology of the facility or unit and preserve the efficacy of the few extant antibiotics for MDR pathogens. Reduction of usage of antibiotics that exert substantial selection pressure (e.g., vancomycin, fluoroquinolones, third-generation cephalosporins) can be used to manage outbreaks and restore susceptibility.19,21,–24
TABLE 13.1
CAUSES AND CONSEQUENCES OF BACTERIAL RESISTANCE AS RELATED TO ANTIBIOTIC SELECTION PRESSURE
MDR, multidrug resistant; MDR gram-negative bacilli include producers of extended-spectrum beta-lactamases, metallo-beta-lactamases, and carbapenemases; MRSA, methicillin-resistant Staphylococcus aureus ; VISA, vancomycin-intermediate Staphylococcus aureus ; VRE, vancomycin-resistant Enterococcus.
PHARMACOKINETICS AND PHARMACODYNAMICS
Pharmacokinetics (PK) describes the principles of drug absorption, distribution, and metabolism.25 Dose–response relationships are influenced by dose, dosing interval, and route of administration. Plasma and tissue drug concentrations are influenced by absorption, distribution, and elimination, which in turn depend on drug metabolism and excretion. Serum drug concentrations may or may not correlate, depending on tissue penetration, but to the extent that they do, relationships between local drug concentration and effect are described by pharmacodynamic (PD) principles (see below).25
Bioavailability describes the percentage of drug dose that reaches the systemic circulation. Bioavailability is 100% after intravenous administration, but after oral administration is affected by absorption, intestinal transit time, and hepatic metabolism, if any. Half-life (T 1/2), the time required for the serum drug concentration to reduce by one-half, reflects both clearance and volume of distribution (V D).25 The V D is independent of a drug’s clearance or T 1/2 and is used to estimate the plasma drug concentration achievable from a given dose. Volume of distribution varies substantially due to pathophysiology; reduced VD may cause a higher plasma drug concentration for a given dose, whereas fluid overload and hypoalbuminemia (which decrease drug binding) increase VD, making dosing more complex. For example, dosing of hydrophilic drugs such as beta-lactams may need to be increased in the early phases of sepsis owing to the expansion of the extracellular space due to fluid resuscitation and alterations in microvascular permeability.26
Clearance refers to the volume of fluid from which drug is eliminated completely per unit of time, regardless of the mode of elimination (e.g., metabolism, excretion, or dialysis); knowledge of drug clearance is important to determine the dose of drug necessary to maintain a steady-state concentration. Most drugs are metabolized by the liver to polar compounds for eventual renal excretion, which may occur by filtration or either active or passive transport. The degree of filtration is determined by molecular size and charge and by the number of functional nephrons. In general, if ≥40% of active drug (including active metabolites) is eliminated unchanged in the urine, a dosage adjustment is required if renal function is decreased.
PDs are unique for antibiotic therapy, because drug–patient, drug–microbe, and microbe–patient interactions must be accounted for.25 The key drug interaction is with the microbe rather than the host. Microbial physiology, inoculum characteristics (i.e., size, quorum sensing, presence of a device-related biofilm),27,28 microbial growth phase, mechanisms of resistance, the microenvironment (e.g., local pH), and the host’s response are important factors to consider. Because of microbial resistance, mere administration of the “correct” drug may not be microbicidal if an adequate dose/concentration is not achieved.29,30 In vitro results may be irrelevant if bacteria are inhibited only by drug concentrations that cannot be achieved clinically.
Antibiotic PD parameters determined by laboratory analysis include the minimal inhibitory concentration (MIC), the lowest serum drug concentration that inhibits bacterial growth (MIC90 refers to 90% inhibition) (Fig. 13.1). However, some antibiotics may suppress bacterial growth at subinhibitory concentrations (postantibiotic effect , PAE).26,31 Appreciable PAE can be observed with aminoglycosides and fluoroquinolones for gram-negative bacteria, and with some β-lactam drugs (notably carbapenems) against S. aureus. However, MIC testing may not detect resistant bacterial subpopulations within the inoculum (e.g., “heteroresistance” of S. aureus),32 which may be selected for by therapy, overgrow in the ecologic vacuum caused by treatment, and cause clinical failure.
FIGURE 13.1. A stylized elimination curve is shown for a single bolus dose of a parenteral antibiotic. Some drugs (e.g., aminoglycosides) exhibit concentration-dependent bactericidal activity; a peak concentration/minimum inhibitory concentration (MIC) ratio >10 is optimal for bacterial killing. Beta-lactam agents exhibit time-dependent bactericidal activity; the proportion (fT) of time above the MIC should be at least 40% for optimal killing. Efficacy of still other drugs (e.g., vancomycin, fluoroquinolones) is reflected by the AUC, a method of measurement of the bioavailability of a drug based on a plot of blood concentrations sampled at frequent intervals. The AUC is directly proportional to the total amount of unaltered drug in the patient’s blood. A ratio of AUC: MIC > 400 is associated with optimal antibacterial effect and minimization of the development of resistance.
Sophisticated analytic strategies utilize both PK and PD, for example, by determination of the peak serum concentration: MIC ratio, the duration of time that plasma concentration remains above the MIC (fT>MIC), and the area of the plasma concentration-time curve above the MIC (the area under the curve or AUC). Accordingly, aminoglycosides exhibit concentration-dependent killing,31,33 whereas beta-lactam agents exhibit efficacy determined by time above the MIC.34 For beta-lactam antibiotics with short T1/2, it may be efficacious to administer by continuous infusion,25,35,36 although prolonged intermittent infusion may accomplish the same while freeing up vascular access for other medications for a period of time each dosing interval. Some agents (e.g., fluoroquinolones, vancomycin) exhibit both properties; bacterial killing increases as drug concentration increases up to a saturation point, after which the effect becomes concentration-independent. An AUC:MIC24h > 125 is associated with optimal effect and reduced risk of developing resistance, whereas some authors recommend an AUC:MIC24h > 400 if treating a MDR pathogen. A framework for considering the dosing of individual antibiotics according to PK/PD principles is presented in Table 13.2.
TABLE 13.2
PHARMACOKINETICS OF BACTERIAL KILLING: IMPLICATIONS FOR ANTIBIOTIC DOSING
aContinuous or prolonged infusion of linezolid is not recommended, as efficacy has not been established.
bExamples of maximized drug dosages include clindamycin 900 mg q8h rather than 600 mg q6h, and vancomycin 15 mg/kg/d for patients with normal renal function. Larger doses of tigecycline (a glyclcycline) and streptogramins (e.g., quinupristin/dalfopristin) may be limited by increased toxicity.
cExamples of dosing to achieve maximized peak drug concentrations include single daily-dose aminoglycoside therapy and metronidazole 1 g q12h rather than 500 mg q8h. Prolonging the dosing interval is not recommended for polymyxins, owing to a negligible PAE.
See text for additional explanations.
Auc, area under the concentration–time curve; Cmax, maximum drug concentration (peak concentration); fT, proportion of time; MIC, minimum inhibitory concentration; MDR, multidrug-resistant; PAE, postantibiotic effect.
Prophylactic antibiotics are used most often to prevent SSIs, for which the benefit has been proved in many circumstances. However, only the incision itself is protected, and only while it is open and thus vulnerable to inoculation. If not administered properly, antibiotic prophylaxis is ineffective and may be harmful. Antibiotic prophylaxis of surgery does not prevent postoperative nosocomial infections, which actually occur at an increased rate after prolonged prophylaxis,37,38 selecting for more resistant pathogens when infection does develop.8,38
Four principles guide the administration of antimicrobial agent for prophylaxis of a surgical incision: safety; an appropriate narrow spectrum of coverage of relevant pathogens; little or no reliance upon the agent for therapy of infection (owing to the possible induction of resistance with heavy usage); and administration within 1 hour before surgery and for a defined, brief period of time thereafter (no more than 24 hours [48 hours for cardiac surgery]; ideally, a single dose).39 According to these principles, quinolones or carbapenems are undesirable agents for surgical prophylaxis, although ertapenem and quinolone prophylaxis have been endorsed by the Surgical Care Improvement Project (SCIP) for prophylaxis of elective colon surgery (the latter with metronidazole for penicillin-allergic patients) (Table 13.3). The SCIP recommendations do not consider emergency surgery.
TABLE 13.3
SURGICAL CARE IMPROVEMENT PROGRAM: APPROVED ANTIBIOTIC PROPHYLACTIC REGIMENS FOR ELECTIVE SURGERY
aProphylaxis may be administered for up to 48 h for cardiac surgery; for all other cases, the limit is 24 h.
bFor beta-lactam allergy, clindamycin or vancomycin is an acceptable substitute for cardiac, vascular, and orthopedic surgery.
cVancomycin is acceptable with a physician-documented justification for use in the medical record.
dFor beta-lactam allergy, clindamycin plus gentamicin, a fluoroquinolone, or aztreonam; or metronidazole plus gentamicin or a fluoroquinolone are acceptable choices.
eFor colon surgery, either oral or parenteral prophylaxis alone, or both combined, are acceptable.
fFor beta-lactam allergy, either clindamycin plus gentamicin, a fluoroquinolone, or aztreonam; or metronidazole plus gentamicin or a fluoroquinolone or clindamycin monotherapy, is an acceptable choice.
CABG, coronary artery bypass grafting.
The spectrum of bacterial contamination of surgical sites is well described.40 Clean surgical procedures affect only skin structures and other soft tissues. Clean-contaminated procedures are characterized by controlled opening of a hollow viscus (e.g., elective aerodigestive or genitourinary tract surgery). Contaminated procedures introduce a large inoculum of bacteria into a normally sterile body cavity, but too briefly for infection to become established during surgery (e.g., penetrating abdominal trauma, enterotomy during adhesiolysis for mechanical bowel obstruction). Dirty procedures are those performed to control established infection (e.g., colon resection for perforated diverticulitis).
Most SSIs are caused by gram-positive cocci; therefore, prophylaxis should be directed primarily against staphylococci for clean cases and high-risk clean-contaminated upper abdominal surgery. A first-generation cephalosporin is preferred in almost all circumstances (Table 13.4), with clindamycin used for penicillin-allergic patients.39 If gram-negative or anaerobic coverage is required, a second-generation cephalosporin, or the combination of a first-generation agent plus metronidazole, is the regimen of first choice. Vancomycin prophylaxis is generally not recommended, except in institutions where the incidence of MRSA infection is high (>20% of all SSIs caused by MRSA).
TABLE 13.4
APPROPRIATE CEPHALOSPORIN PROPHYLAXIS FOR SELECTED OPERATIONS
a Should be given as a single intravenous dose just before the operation. Consider an additional dose if the operation is prolonged longer than 3–4 h.
b Primary prophylaxis with vancomycin (i.e., for the non–penicillin-allergic patient) may be appropriate for cardiac valve replacement, placement of a non-tissue peripheral vascular prosthesis, or total joint replacement in institutions where a high rate of infections with methicillin-resistant S. aureus or S. epidermidis has occurred. The precise definition of “high rate” is debated. A single dose administered immediately before surgery is sufficient unless operation lasts for more than 6 h, in which case the dose should be repeated. Prophylaxis should be discontinued after a maximum of two doses but may be continued for up to 48 h.
c An intraoperative dose should be given if cefoxitin is used and the duration of surgery exceeds 3–4 h, because of the short half-life of the drug. A postoperative dose is not necessary but is permissible for up to 24 h.
d Benefit beyond that provided by bowel preparation with mechanical cleansing and oral neomycin and erythromycin base is debatable.
The optimal time to administer parenteral antibiotic prophylaxis is within 1 hour prior to incision.41 Antibiotics given sooner are ineffective, as are agents given after the incision is closed. A 2001 audit of prescribing practices in the United States indicated that only 56% of patients who received prophylactic antibiotics did so within 1 hour prior to the skin incision42; timeliness was documented in only 76% of cases in a 2005 audit in US Department of Veterans Affairs hospitals.42
Most inappropriately timed first doses of prophylactic antibiotic occur too early.41,42 Antibiotics with short half-lives (<2 hours, e.g., cefazolin or cefoxitin) should be redosed every 3–4 hours during surgery if the operation is prolonged or bloody.43 Even though SCIP specifies a 24-hour limit for prophylaxis, single-dose prophylaxis (with intraoperative redosing, if indicated) is equivalent to multiple doses for the prevention of SSI.44 Prolonged prophylaxis increases the risk of nosocomial infections unrelated to the surgical site, and the emergence of MDR pathogens. Both pneumonia and vascular catheter–related infections have been associated with prolonged prophylaxis,37,38,45,46 as has the emergence of SSI caused by MRSA.8
Recent US data show that only 40% of patients who receive antibiotic prophylaxis do so for <24 hours.47 As a result of ischemia caused by surgical hemostasis, antibiotic penetration into the incision immediately after surgery is questionable until neovascularization occurs (24–48 hours). Antibiotics should not be given to “cover” indwelling drains or catheters, in lavage or irrigation fluid,48 or as a substitute for poor surgical technique.
Antibiotic prophylaxis is indicated for most clean-contaminated and contaminated (or potentially contaminated) operations. Antibiotic prophylaxis is indicated for high-risk biliary surgery; high risk is conferred by age >70 years, diabetes mellitus, or a recently instrumented biliary tract (e.g., biliary stent). By contrast, antibiotic prophylaxis is usually not indicated for elective laparoscopic cholecystectomy49; meta-analysis of 12 trials revealed no benefit compared with placebo for prevention of SSI (odds ratio [OR] 1.07, 95% confidence interval [CI], 0.59–1.94; p = 0.99), or “overall infection,” “major infection,” or “distant infection.” Emergency cholecystectomy (regardless of technique) is performed most commonly for acute cholecystitis, for which antibiotic administration would be therapeutic, not prophylactic.
Antibiotic prophylaxis of clean surgery is controversial. Where bone is incised (e.g. craniotomy, sternotomy) or a prosthesis is inserted, antibiotic prophylaxis is generally indicated. Meta-analysis of randomized controlled trials shows a decrease of SSI rate for groin hernia surgery,50 especially when nonabsorbable mesh is implanted. Twelve randomized clinical trials (6,705 patients) were identified, six of which used prosthetic material (hernioplasty). Infection rates were 2.9% and 3.9% in the prophylaxis and control groups, respectively (OR 0.64, 95% CI 0.48-0.85). Patients with herniorrhaphy had infection rates of 3.5% and 4.9% in the prophylaxis and control groups, respectively (OR 0.71, 95% CI 0.51–1.00). Patients with hernioplasty had infection rates of 1.4% and 2.9% in the prophylaxis and control groups, respectively (OR 0.48, 95% CI 0.27-0.85).
The incidence of SSI is higher for abdominal wall incisional hernia repair than for groin hernioplasty, especially for open surgery as compared with laparoscopic repair,51,52 and is increased dramatically if an enterotomy occurs during adhesiolysis.53 Because of the known risk of SSI, antibiotic prophylaxis is warranted, but has not been examined with rigor.
Arterial reconstruction with a prosthetic graft, especially infrainguinal, is an example of clean surgery where the risk of infection is substantial. Meta-analysis54 of 23 randomized, controlled trials of prophylactic antibiotics for peripheral arterial reconstruction demonstrated that prophylaxis reduced the risk of SSI by approximately 75%, and early graft infection by about 69%. There was no benefit to prophylaxis for more than 24 hours, of antibiotic bonding to the graft material itself, or preoperative bathing with an antiseptic agent compared with unmedicated bathing.
Skin closure of a contaminated or dirty incision increases the risk of SSI, but few good studies evaluate the multiplicity of wound closure techniques used by surgeons. “Open abdomen” techniques of temporary abdominal closure for management of trauma or severe peritonitis are utilized increasingly. Antibiotic prophylaxis of the open abdomen is probably not indicated55 (Table 13.5), although an inability to achieve primary abdominal closure is associated with several nosocomial infections (pneumonia, bloodstream infection, and SSI) and substantially increased cost from prolonged length of stay, but not mortality.56
TABLE 13.5
ANTIBIOTIC PROPHYLAXIS OF TRAUMA-SUMMARY OF EVIDENCE AND PUBLISHED GUIDELINES
ORIF: Open reduction.internal fixation.
Drains placed in incisions may cause more infections than they prevent. Epithelialization of the wound is prevented, and the drain becomes a conduit, holding open a portal for invasion by pathogens colonizing the skin. Several studies of drains placed into clean or clean-contaminated incisions show that the rate of SSI is not reduced57,58; in fact, the rate is increased.59,61–62 Considering that drains pose this risk, they should be used as little as possible and removed as soon as possible.63 Under no circumstances should prolonged antibiotic prophylaxis be administered to “cover” indwelling drains.
For trauma, antibiotic prophylaxis may be required for operative management, but may also be indicated to prevent traumatic wounds from becoming infected. Obviously, the principle of administration within 1 hour before surgical incision does not apply in the latter case. Moreover, many surgical procedures performed for trauma are likely to be in a contaminated field (e.g., penetrating trauma, open reduction/internal fixation of open fractures). Likewise, as many as three-quarters of traumatic wounds are likely to be contaminated to some degree, the likelihood of infection is increased when the degree of contamination is higher.64 The evidence for antibiotic prophylaxis of traumatic injury is presented in Table 13.5.55,65–89 The evidence is most robust for penetrating abdominal trauma, for which no more than 24 hours of prophylaxis with a second-generation cephalosporin (or equivalent) is recommended, even for colon injury. Weaker data sets that have been subjected to robust analysis include the evidence for prophylaxis of open extremity fractures.
PRINCIPLES OF ANTIBIOTIC THERAPY
Antimicrobial therapy is a mainstay of the treatment of infections, but widespread overuse and misuse of antibiotics have led to an alarming increase in MDR pathogens (Table 13.1). New agents and innovative ways to administer existing antibiotics may allow shorter courses of therapy, which is desirable for cost savings and control of microbial ecology. Effective therapy with no toxicity requires a careful but expeditious search for the source of infection and an understanding of the principles of PK (see above).
Evaluation of Possible Infection
Absent a fever, any of hypotension, tachycardia, tachypnea, confusion, rigors, skin lesions, respiratory manifestations, oliguria, lactic acidosis, leukocytosis, leukopenia, immature neutrophils (i.e., bands >10%), or thrombocytopenia may indicate a workup for infection and immediate empiric therapy. A new temperature elevation is usually the trigger for an evaluation for the presence of infection (hence, “fever workup”). However, some infected patients do not manifest fever, and may be even be hypothermic. Such patients include elderly patients; those with open abdominal wounds, end-stage liver disease, or chronic kidney disease; and patients taking anti-inflammatory or anti-pyretic drugs. Moreover, fever in the early postoperative period often has a non-infectious cause, hence fever does not equate with infection (Table 13.6).90,91 The acute care surgeon must also bear in mind that non-infectious and infectious causes of fever may coexist, and that an infected patient may harbor more than one discrete focus of infection.
TABLE 13.6
MISCELLANEOUS CAUSES OF FEVER RELATED TO NON-INFECTIOUS STATES
Antibiotics should not be given until evaluation has occurred, including the collection of specimens for culture. Therefore, evaluation must be expeditious, as delay in antibiotic administration is associated with an increased risk of death.–9295 It is mandatory to remove the surgical dressing to inspect the incision as part of any fever evaluation. However, if an incision is opened and cultured, a deep culture specimen should be collected; swabbing an open wound superficially or collecting fluid from drains (if present) for culture is un-helpful because the likelihood of colonization is high. A chest x-ray is optional for evaluation of early postoperative fever unless mechanical ventilation, physical examination, abnormal blood gases, or pulmonary secretions suggest a high yield. Urinalysis or culture is not mandatory in the early postoperative period unless there is reason by history or examination to suspect a UTI. After trauma, UTI is common only after injury to the urinary tract.96
Traditional diagnostic criteria have low specificity for the diagnosis of HAP/VAP; therefore, culture of a lower respiratory tract sample is mandatory for nosocomial pneumonia prior to administration of antibiotics. There is controversy regarding methods of sputum specimen collection (invasive vs. noninvasive) and specimen analysis (semiquantitative vs. quantitative). The crucial issue in diagnosis of HAP/VAP is whether a sputum isolate reflects colonization rather than infection. Endotracheal suction aspirates have lower specificity than deep specimens collected by bronchoalveolar lavage or protected-specimen brush, due to an increased likelihood of contamination of the former by oropharyngeal flora,97 although it is unclear if this makes a difference clinically. A meta-analysis of randomized trials that compared outcomes of patients with VAP managed with invasive versus noninvasive sampling showed no difference in mortality,98 although patients in the invasive group were more likely to undergo narrowing of the antimicrobial regimen or cessation of therapy.
Blood cultures should be obtained from febrile patients when clinical evaluation does not suggest a non-infectious cause. The venipuncture site should be cleaned with either 2% chlorhexidine gluconate in 70% isopropyl alcohol or 1–2% tincture of iodine. Povidone–iodine (10%) is acceptable but not bactericidal until dry; false-positive blood cultures may result from premature specimen collection.99 For adults, a minimum 20–30 mL sample of blood is drawn at a single time from a single site; additional guidelines apply for blood cultures taken from new or existing central venous catheters.91 The minimum inoculum for an adult blood culture should be 10 mL/bottle. The cumulative yield of pathogens is optimized when three blood cultures (i.e., six bottles inoculated) with adequate volume (20–30 mL each) are drawn.100 The sensitivity of blood culturing for detection of true bacteremia or fungemia is related to many factors, most importantly the volume of blood drawn and obtaining the cultures before initiation of anti-infective therapy.100
Empiric antibiotic therapy must be administered judiciously, but expeditiously. Injudicious therapy could result in undertreatment of established infection, or unnecessary therapy in the setting of sterile inflammation or bacterial colonization; either may be deleterious. Inappropriate therapy (e.g., delay,92,101 therapy misdirected against usual pathogens, failure to treat MDR pathogens) leads unequivocally to increased mortality.93–95 There is urgency because delay in initiation of empiric antibiotic therapy of as little as 30–60 minutes can result in increased mortality.101,102 Current guidelines for the diagnosis and management of severe sepsis recommend that empiric antibiotic therapy be instituted with 1 hour of presentation.103
Antibiotic choice is based on several interrelated factors (Tables 13.7–13.9). Paramount is activity against identified or likely (for empiric therapy) pathogens, presuming infecting and colonizing organisms can be distinguished, and that narrow-spectrum coverage is always desired. Estimation of likely pathogens depends on the disease process believed responsible; whether the infection is community-, health care–, or hospital-acquired; and whether MDR organisms are present, or likely to be. Local knowledge of antimicrobial resistance patterns is essential, even at the unit-specific level. Patient-specific factors of importance include age, debility, immunosuppression, intrinsic organ function, prior allergy or other adverse reaction, and recent antibiotic therapy. Institutional factors of importance include guidelines that may specify a particular therapy, formulary availability of specific agents, outbreaks of infections caused by MDR pathogens, and antibiotic control programs.
TABLE 13.7
FACTORS INFLUENCING ANTIBIOTIC CHOICE
TABLE 13.8
RANK ORDER OF KEY BACTERIAL PATHOGENS IN ICU INFECTIONS (BY INCIDENCE)
aYeast are also important pathogens in blood stream and UTIs.
bYeast are seldom pathogens in nosocomial infection, except for solid-organ transplant recipients and patients undergoing antineoplastic chemotherapy.
Coag-neg, coagulase-negative.
TABLE 13.9
BACTERIAL RESISTANCE: PROBLEM INFECTIONS AND PATHOGENS
CA-MRSA, community-acquired methicillin-resistant S. aureus ; CAP, community-acquired pneumonia; CLABSI, central line–associated blood stream infection; ESBL, extended-spectrum beta lactamase; VAP, ventilator-associated pneumonia; VRE, vancomycin-resistant Enterococcus.
The number of (+) indicators reflects relative prevalence.
Antibiotic stewardship programs20 support optimal antibiotic administration, including physician prescribing patterns, computerized decision support, administration by protocol, and formulary restriction programs. Owing to the increasing prevalence of MDR pathogens, it is crucial for initial empiric antibiotic therapy to be targeted appropriately, administered according to PK/PD principles, given in sufficient dosage to assure bacterial killing, narrowed in spectrum (de-escalation)104 as soon as possible based on microbiology data and clinical response, and continued only as long as necessary.105 Appropriate antibiotic prescribing not only optimizes patient care but supports infection control practice and preserves microbial ecology.20,104
Choice of Antibiotic Numerous agents are available for therapy (Table 13.10).106,107 Agents may be chosen based on spectrum, whether broad or targeted (e.g., antipseudomonal, antianaerobic), in addition to the above factors. If a nosocomial gram-positive pathogen is suspected (e.g., wound or SSI, CLABSI, HAP/VAP) or MRSA is endemic, empiric vancomycin (or linezolid) is appropriate. Some authorities recommend dual-agent therapy for serious Pseudomonas infections (i.e., an antipseudomonal beta-lactam drug plus an aminoglycoside), but evidence of efficacy is mixed.–108111 Combination therapy of a specific pathogen (e.g., “double coverage” of Pseudomonas) may actually worsen outcomes. Meta-analysis of β-lactam monotherapy versus β-lactam/aminoglycoside combination therapy for immunocompetent patients with sepsis (64 trials, 7,586 patients) found no difference in either mortality (RR 0.90, 95% CI 0.77–1.06) or the development of resistance.109 Clinical failure was more common with combination therapy, as was the incidence of acute kidney injury. Regardless of the specifics of the choice that is made, initial empiric therapy of any infection caused potentially by either a gram-positive or gram-negative bacterium (e.g., HAP/VAP, hospital-acquired intra-abdominal infection) must include activity against all likely pathogens.106,112–114
TABLE 13.10
ANTIBACTERIAL AGENTS FOR EMPIRIC USE
Optimization of Therapy Conventional antibiotic dosing may not apply to the critically ill or injured patient.26 Higher doses may be required for MDR isolates,26,115–117 increased extracellular volume,26,118 decreased serum albumin concentration,119 or increased glomerular filtration rate (e.g., morbid obesity,120 burns, traumatic brain injury,121 multiple trauma). Underdosing of antibiotics is a major factor for the development of resistance during therapy and failure thereof.116 By contrast, lower doses may be required with multiple organ dysfunction syndrome,122 acute kidney injury, and chronic kidney disease (see below and Chapter 52). Dosing of vancomycin and aminoglycosides may be monitored via measurement of drug concentrations in serum,123–125 and may be useful to limit toxicity.126 Therapeutic drug concentration monitoring is possible for a host of other antibiotics, although not widely available.
The practical and financial challenges of performing PK studies in critically ill patients have made valuable mathematical modeling such as Monte Carlo simulation (MCS) to perform virtual clinical trials.127 In order to utilize MCS, four conditions must be present: (1) A robust population PK model must be available for the patient population of interest; (2) descriptors of the effect of covariates that influence PK are needed; (3) the susceptibility of bacteria to the modeled antibiotic must be known; and (4) a PK/PD target must be associated with efficacy. Probability of target attainment outputs describe the proportion of patients who will achieve a prespecified PD target for any given MIC. Such analyses can then inform dosing decisions to achieve a high likelihood of achieving PK/PD targets for organisms with different MICs.
Zelenitsky et al. provided a useful example of MCS for interpreting the optimal methods for dosing meropenem, piperacillin/tazobactam, cefepime, and ceftobiprole in critically ill patients.128 For meropenem, at MICs up to 8 mcg/mL, the probability of achieving 40% fT > MIC was 96%, 90%, and 61% for 3-hour infusions of 2 g q8h, 1 g q8h, and 1 g q12h, respectively, in patients with creatinine clearances ≥50, 30–49, and 10–29 mL/min, respectively. Target attainment was 75%, 65%, and 44% for these same dosing regimens as 0.5 hour infusions.
Continuous or prolonged infusion of beta-lactam agents may be the optimal way to administer these drugs–129132 (Figs. 13.2 and 13.3). Lengthened fT > MIC is achieved, increasing the likelihood of therapeutic success, especially against organisms with higher MICs, while minimizing the possibility of the development of resistance. Prolonged infusions may accomplish the same without monopolizing an intravenous line. Clinical reports support the use of prolonged infusions of beta-lactam antibiotics,–133135 sometimes at lower total dose when highly susceptible organisms are being treated. However, adoption into clinical practice is not widespread.136 Continuous-infusion vancomycin has been described,137 but is not recommended currently (Table 13.11).
FIGURE 13.2. A: Stylized curves of bolus 30-min infusions of antibiotic at 6-h intervals. The proportion of time (fT) above the minimum inhibitory concentration (MIC) must be at least 40% for bactericidal activity in most cases. If the MIC is low, this can be achieved with conventional dosing, but not for organisms with higher MICs. B: Continuous infusion of antibiotic after an initial loading dose (dotted lines) is depicted. Not only is fT:MIC higher, but the antibiotic becomes effective against organisms with higher MICs, depending on the rate of continuous infusion. Lower total daily doses of antibiotic may be used against bacteria with low MICs. For drugs that exhibit time-dependent bactericidal activity (e.g., beta-lactams), this is the ideal mode of administration, provided vascular access is sufficient.
FIGURE 13.3. A conventional bolus dose of antibiotic with a 30-min infusion and a 6-h dosing interval is compared with the same drug given as a 4-h infusion over the same dosing interval. fT:MIC is doubled (compare the lengths of the short and long double arrows), while organisms with higher MICs can still be treated. The interruption allows the intravenous line to be used for fluid or other medication during the hiatus, without compromising bactericidal action.
TABLE 13.11
VANCOMYCIN MIC INTERPRETIVE CRITERIA FOR S. AUREUS
From FDA Lowers Vancomycin Breakpoints for Staph Infections. May 1, 2008. Available at:http://news.idsociety.org/idsa/issues/2008-05-01/. Accessed July 5, 2011.
MIC: minimal inhibitory conccentration.
Vancomycin has been a mainstay of therapy of infections of critically ill patients, but MICs for vancomycin against MRSA have been increasing, even within the susceptible range. As a result, MIC cutpoints for resistance of S. aureus have been revised downward to minimize the chance of ineffective therapy (Table 13.12),138 and higher doses of vancomycin are recommended (Table 13.11),124,125 although at a greater risk of nephrotoxicity. Mortality in patients with MRSA HAP, VAP, and HCAP increases as a function of the vancomycin MIC, even for strains with MIC values within the susceptible range (Table 13.13).139,140 The use of vancomycin therapy in patients with MRSA infections caused by isolates with MICs between 1 and -2 mg/mL should be undertaken with caution. Adequate therapeutic concentrations may not be achievable for S. aureus isolates with MICs >2 mcg/mL,141 so alternative therapy should be considered. Linezolid retains generally excellent activity,142 but resistance to daptomycin is being reported.143
TABLE 13.12
SUMMARY OF VANCOMYCIN DOSING GUIDELINES
Adapted from 124, 125
MRSA: methicillin-resistant S. aureus; SSTI: skin and soft tissue infection.
TABLE 13.13
CAUSES OF VANCOMYCIN FAILURE IN A SINGLE-CENTER COHORT OF 320 PATIENTS WITH DOCUMENTED MRSA BACTEREMIA. LOGISTIC REGRESSION ANALYSIS
MIC, minimum inhibitory concentration; MRSA, methicillin-resistant S. aureus.
From Patel N, Pai MP, Rodvold KA, et al. Vancomycin: We can’t get there from here. Clin Infect Dis. 2011; 52:969–974
Aminoglycoside therapy is resurgent owing to limited options for treatment of MDR pathogens that have resulted from the increasing prevalence of extended-spectrum beta-lactamase (ESBL)-producing strains and the emergence of carbapenemases, although use as part of an empiric therapy regimen remains debated.108,109,123 Single daily-dose aminoglycoside therapy ensures that a peak:MIC concentration ratio >10 will be achieved to optimize therapy. A dose of gentamicin or tobramycin (7 mg/kg) or amikacin (20 mg/kg) is administered and a trough concentration is determined at 23-hour postdose (some protocols call for an intermediate determination as well, at approximately 16-hour post-dose, so that the elimination curve can be determined with greater precision). A trough concentration of 0.5–2 mcg/mL is sought for gentamicin or tobramycin, whereas a trough concentration of 5–10 mcg/mL is ideal for amikacin. Outcomes are comparable or better compared with conventional dosing, with decreased toxicity. Single-daily-dose aminoglycoside therapy has not been validated for children, pregnant patients, patients with burns, patients aged >70 years, or treatment of bacterial endocarditis.
Infections caused by highly resistant or pan-resistant gram-negative bacilli pose a major problem. Carbapenems, tigecycline, and polymyxins (see below) retain useful activity against ESBL-producing organisms. MDR non-fermenting gram-negative bacilli (e.g., P. aeruginosa , Acinetobacter spp., Stenotrophomonas spp., and carbapenemase-producing Enterobacteriaceae) may require therapy with a polymyxin, high-dose carbapenem by prolonged infusion, or unusual combinations of agents that have demonstrated synergy in vitro , but remain of uncertain clinical utility.106,107,143,144
Duration of Therapy
The end point of antibiotic therapy is largely undefined, because quality data are few. If cultures are negative, empiric antibiotic therapy should be stopped in most cases after no more than 48–72 hours. Unnecessary antibiotic therapy increases the risk of MDR infection; therefore, prolonged therapy with negative cultures is usually unjustifiable. The morbidity of antibiotic therapy also includes allergic reactions, development of nosocomial superinfections, (e.g., fungal, enterococcal, and Clostridium difficile–related infections), organ toxicity, reduced yield from subsequent cultures, and vitamin K deficiency with coagulopathy or accentuation of warfarin effect.
If infection is evident, treatment is continued as indicated clinically, but the microbiology data should be examined to see if the antibiotic regime can be de-escalated to a narrower regimen (e.g., choosing a narrower-spectrum agent, changing multi-drug therapy to monotherapy). If empiric therapy has been appropriate, the need to escalate therapy at this point (e.g., choosing a more broad-spectrum agent, changing monotherapy to multi-drug therapy, adding antifungal therapy [see below]) should be a rare event.
Some infections can be treated with therapy lasting 4 days or less105 (Table 13.14). Every decision to start antibiotics must be accompanied by an a priori decision regarding duration of therapy. A reason to continue therapy beyond the predetermined end point must be compelling. Bacterial killing is rapid in response to effective agents, but the host response may not subside immediately. Therefore, the clinical response of the patient should not be the sole determinant. There is increasing belief that shorter courses of antibiotic therapy that were used previously are equally effective with fewer side effects, and that therapy should continue to a predetermined end point, after which it should be stopped.
TABLE 13.14
CURRENT RECOMMENDATIONS FOR DURATION OF THERAPY FOR SELECTED SERIOUS INFECTIONS
aIf the patient is not immunosuppressed or neutropenic, the catheter has been removed, there is no recent placement of a prosthetic intravascular device, and there is neither evidence of endocarditis (by echocardiography) or suppurative thrombophlebitis (by ultrasound). Fever and bacteremia must resolve within 72 h, and there must be no evidence of metastatic infection by physical examination. If these conditions are not met, six weeks of therapy is recommended.
bSupported by one or more randomized, prospective trials, rather than expert opinion.
CAP, community-acquired pneumonia; NFGNB, nonfermenting gram-negative bacilli; VAP, ventilator-associated pneumonia
From Hayashi DL, Paterson DL. Strategies for reduction in duration of antibiotic use in hospitalized patients. Clin Infect Dis. 2011; 52:1232–1240.
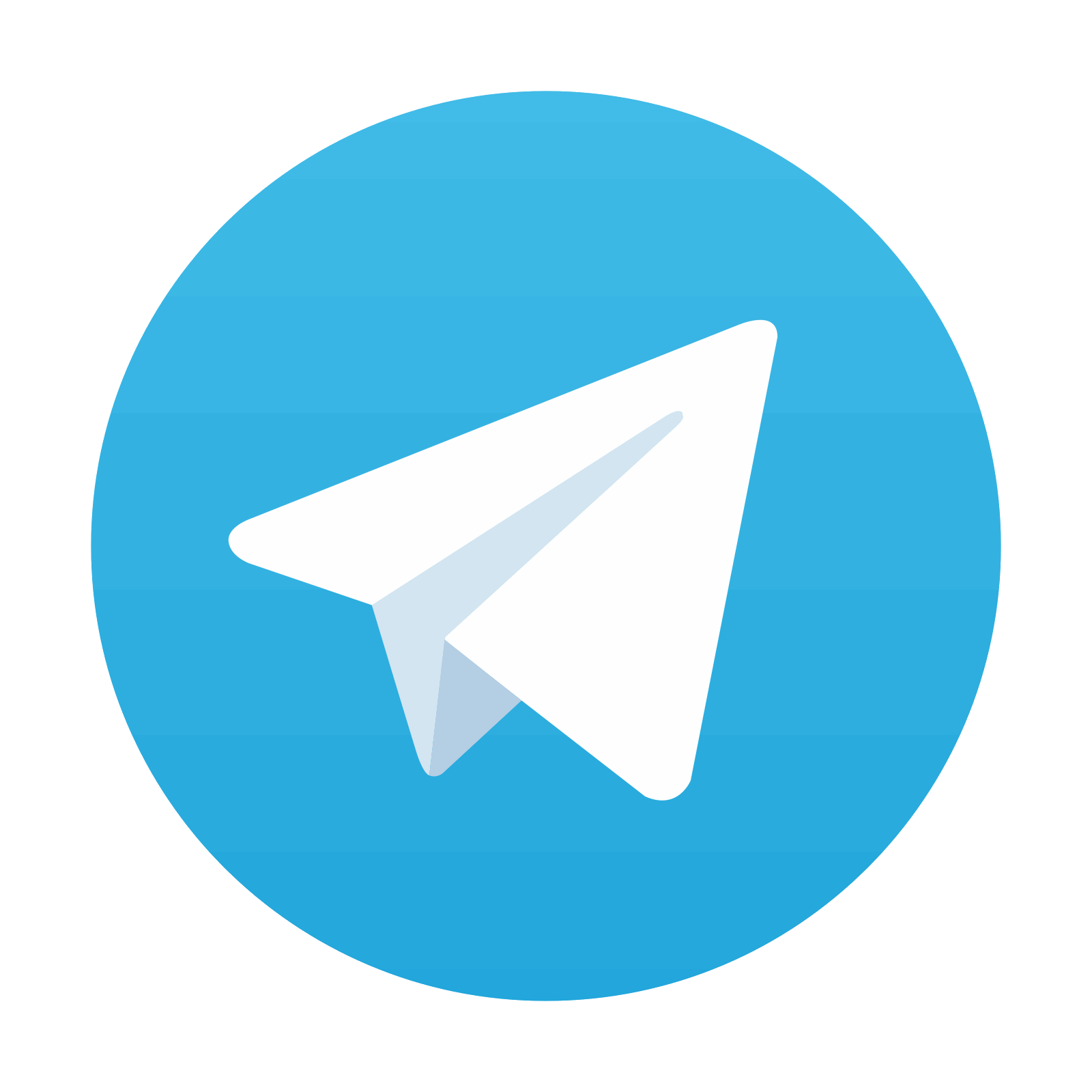
Stay updated, free articles. Join our Telegram channel

Full access? Get Clinical Tree
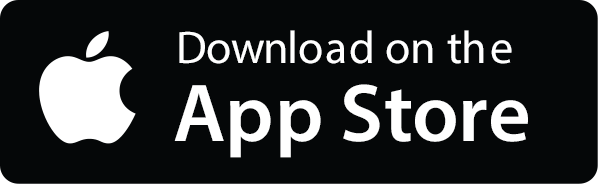
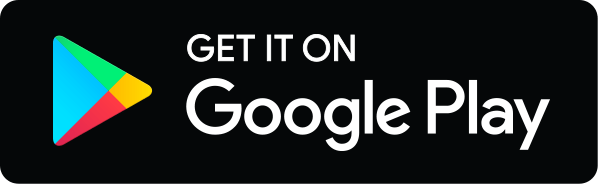