Key Concepts
Cardiopulmonary bypass (CPB) is a technique that diverts venous blood away from the heart (most often from one or more cannulas in the right atrium), adds oxygen, removes CO2, and returns the blood through a cannula in a large artery (usually the ascending aorta or a femoral artery). As a result, nearly all blood bypasses the heart and lungs.
The fluid level in the reservoir is critical. If a “roller” pump is used and the reservoir is allowed to empty, air can enter the main pump and be embolized into the patient where it may cause organ damage or fatality.
Initiation of CPB is associated with a variable increase in stress hormones and systemic inflammatory response.
Establishing the adequacy of the patient’s preoperative cardiac function should be based on exercise (activity) tolerance, measurements of myocardial contractility such as ejection fraction, the severity and location of coronary stenoses, ventricular wall motion abnormalities, cardiac end-diastolic pressures, cardiac output, and valvular areas and gradients.
Blood should be immediately available for transfusion if the patient has already had a midline sternotomy (a “redo”); in these cases, the right ventricle or coronary grafts may be adherent to the sternum and may be accidentally entered during the repeat sternotomy.
In general, pulmonary artery catheterization has been most often used in patients with compromised ventricular function (ejection fraction <40-50%) or pulmonary hypertension and in those undergoing complicated procedures.
Transesophageal echocardiography (TEE) provides valuable information about cardiac anatomy and function during surgery. Two-dimensional, multiplane TEE can detect regional and global ventricular abnormalities, chamber dimensions, valvular anatomy, and the presence of intracardiac air. Three-dimensional TEE provides a more complete description of valvular anatomy and pathology.
Anesthetic dose requirements are variable and patient tolerance of inhaled anesthetics generally declines with declining ventricular function. Severely compromised patients should be given anesthetic agents in incremental, small doses.
Anticoagulation must be established before CPB to prevent acute disseminated intravascular coagulation and formation of clots in the CPB pump.
Antifibrinolytic therapy may be particularly useful for patients who are undergoing a repeat operation; who refuse blood products, such as Jehovah’s Witnesses; who are at high risk for postoperative bleeding because of recent administration of glycoprotein IIb/IIIa inhibitors (abciximab, eptifibatide, or tirofiban); who have preexisting coagulopathy; and who are undergoing long and complicated procedures involving the heart or aorta.
Hypotension from impaired ventricular filling may occur during manipulation of the venae cavae and the heart.
Hypothermia (<34°C) potentiates general anesthetic potency, but failure to give anesthetic agents, particularly during rewarming on CPB, may result in awareness and recall.
Protamine administration can result in a number of adverse hemodynamic effects, some of which are immunological in origin. Protamine given slowly (5-10 min) usually has few effects; when given more rapidly it produces a fairly consistent vasodilation that is easily treated with blood from the pump oxygenator and small doses of phenylephrine. Catastrophic protamine reactions often include myocardial depression and marked pulmonary hypertension. Diabetic patients previously maintained on protamine-containing insulin (such as NPH) may be at increased risk for adverse reactions to protamine.
Persistent bleeding often follows prolonged durations of bypass (>2 h) and in most instances has multiple causes. Inadequate surgical control of bleeding sites, incomplete reversal of heparin, thrombocytopenia, platelet dysfunction, hypothermia-induced coagulation defects, undiagnosed preoperative hemostatic defects, or newly acquired factor deficiency or hypofibrinogenemia may be responsible.
Chest tube drainage in the first 2 h of more than 250-300 mL/h (10 mL/kg/h)—in the absence of a hemostatic defect—is excessive and may require surgical reexploration. Intrathoracic bleeding at a site not adequately drained may cause cardiac tamponade, requiring immediate reopening of the chest.
Factors known to increase pulmonary vascular resistance (PVR) such as acidosis, hypercapnia, hypoxia, enhanced sympathetic tone, and high mean airway pressures are to be avoided for patients with right-to-left shunting; hyperventilation (hypocapnia) with 100% oxygen is usually effective in lowering PVR. Conversely, patients with left-to-right shunting benefit from systemic vasodilation and increases in PVR, although specific hemodynamic manipulation is generally not attempted.
Induction of general anesthesia in patients with cardiac tamponade can precipitate severe hypotension and cardiac arrest.
The sudden increase in left ventricular afterload after application of the aortic cross-clamp during aortic surgery may precipitate acute left ventricular failure and myocardial ischemia, particularly in patients with underlying ventricular dysfunction or coronary disease. The period of greatest hemodynamic instability follows the release of the aortic cross-clamp; the abrupt decrease in afterload together with bleeding and the release of vasodilating acid metabolites from the ischemic lower body can precipitate severe systemic hypotension.
The emphasis of anesthetic management during carotid surgery is on maintaining adequate perfusion to the brain and heart.
Anesthesia for Cardiovascular Surgery: Introduction
Anesthesia for cardiovascular surgery requires an understanding of circulatory physiology, pharmacology, and pathophysiology as well as a familiarity with cardiopulmonary bypass (CPB) pumps, filters, and circuitry; transesophageal echocardiography (TEE); and techniques of myocardial preservation. Because surgical manipulations often have a profound impact on circulatory function, the anesthesiologist must understand the rationale behind the surgical techniques, follow the progress of the surgery, and anticipate the potential problems associated with each step.
Cardiopulmonary Bypass
CPB is a technique that diverts venous blood away from the heart (most often from one or more cannulas in the right atrium), adds oxygen, removes CO2, and returns the blood through a cannula in a large artery (usually the ascending aorta or a femoral artery). As a result, nearly all blood bypasses the heart and lungs. When CPB is fully established, the extracorporeal circuit is in series with the systemic circulation and provides both artificial ventilation and perfusion. This technique provides distinctly nonphysiological conditions, because arterial pressure is usually less than normal and blood flow is usually nonpulsatile. To minimize organ damage during this stressful period, various degrees of systemic hypothermia may be employed. Topical hypothermia (an ice-slush solution) and cardioplegia (a chemical solution for arresting myocardial electrical activity) may also be used to protect the heart.
The operation of the CPB machine is a complex task requiring the attention of a perfusionist—a specialized (and certified) technician. Optimal results with CPB require close cooperation and communication between the surgeon, anesthesiologist, and perfusionist.
The typical CPB machine has six basic components: a venous reservoir, an oxygenator, a heat exchanger, a main pump, an arterial filter, tubing that conducts venous blood to the venous reservoir, and tubing that conducts oxygenated blood back to the patient (Figure 22-1). Modern machines use a single disposable unit that includes the reservoir, oxygenator, and heat exchanger. Most machines also have separate accessory pumps that can be used for blood salvage (cardiotomy suction), venting (draining) the left ventricle, and administration of cardioplegia solutions. A number of other filters, alarms, and in-line pressure, oxygen-saturation, and temperature monitors are also typically used.
Prior to use, the CPB circuit must be primed with fluid (typically 1200-1800 mL for adults) that is devoid of bubbles. A balanced salt solution, such as lactated Ringer’s solution, is generally used, but other components are frequently added, including colloid (albumin or starch), mannitol (to promote diuresis), heparin (500-5000 units), and bicarbonate. At the onset of bypass, hemodilution decreases the hematocrit to about 22-27% in most patients. Blood is included in priming solutions for smaller children and severely anemic adults to prevent severe hemodilution.
The reservoir of the CPB machine receives blood from the patient via one or two venous cannulas placed in the right atrium, the superior and inferior vena cava, or a femoral vein. With most circuits blood returns to the reservoir by gravity drainage. During extracorporeal circulation the patient’s venous pressure is normally low. Thus, the driving force for flow into the pump is directly related to the difference in height between the patient and the reservoir and inversely proportional to the resistance of the cannulas and tubing. An appropriately primed CPB machine draws in blood like a siphon. Entrainment of air in the venous line can produce an air lock that may prevent blood flow. With some circuits (eg, use of an unusually small venous cannula) assisted venous drainage may be required; a regulated vacuum together with a hard shell venous reservoir or centrifugal pump (see below) is used in such instances. The fluid level in the reservoir is critical. If a “roller” pump is used and the reservoir is allowed to empty, air can enter the main pump and be embolized into the patient where it may cause organ damage or fatality. A low reservoir level alarm is typically present. Centrifugal pumps will not pump air but have the disadvantage of not impelling a well-defined volume with each turn of the head (unlike roller pumps).
Blood is drained by gravity from the bottom of the venous reservoir into the oxygenator, which contains a blood-gas interface that allows blood to equilibrate with the gas mixture (primarily oxygen). A volatile anesthetic is frequently added to the oxygenator gas mixture. The blood-gas interface in a modern, membrane-type oxygenator is a very thin, gas-permeable silicone membrane. Arterial CO2 tension during CPB is dependent on total gas flow past the oxygenator. By varying the inspired oxygen concentration, a membrane oxygenator allows independent control of Pao2 and Paco2.
Blood from the oxygenator enters the heat exchanger and can either be cooled or warmed, depending on the temperature of the water flowing through the exchanger; heat transfer occurs by conduction. Because gas solubility decreases as blood temperature rises, a filter is built into the unit to catch any bubbles that may form during rewarming.
Modern CPB machines use either an electrically driven double-arm roller (positive displacement) or a centrifugal pump to propel blood through the CPB circuit.
Roller pumps produce flow by compressing large-bore tubing in the main pumping chamber as the roller heads turn. Subtotal occlusion of the tubing prevents excessive red cell trauma. The rollers pump blood regardless of the resistance encountered, and produce a nearly continuous nonpulsatile flow. Flow is directly proportional to the number of revolutions per minute. In some pumps, an emergency back-up battery provides power in case of an electrical power failure. All roller pumps have a hand crank to allow manual pumping, but those who have hand cranked a roller pump head will confirm that this is not a good long-term solution.
Centrifugal pumps consist of a series of cones in a plastic housing. As the cones spin, the centrifugal forces created propel the blood from the centrally located inlet to the periphery. In contrast to roller pumps, blood flow with centrifugal pumps is pressure sensitive and must be monitored by an electromagnetic flowmeter. Increases in distal pressure will decrease flow and must be compensated for by increasing the pump speed. Because these pumps are nonocclusive, they are less traumatic to blood than roller pumps. Unlike roller pumps, which are placed after the oxygenator (Figure 22-1), centrifugal pumps are normally located between the venous reservoir and the oxygenator. Centrifugal (unlike roller) pumps have the advantage of not being able to pump air.
Pulsatile blood flow is possible with some roller pumps. Pulsations can be produced by instantaneous variations in the rate of rotation of the roller heads; they can also be added after flow is generated. Pulsatile flow is not available with centrifugal pumps. Although there is no consensus and the data are contradictory, some clinicians believe that pulsatile flow improves tissue perfusion, enhances oxygen extraction, attenuates the release of stress hormones, and results in lower systemic vascular resistances (SVRs) during CPB.
Particulate matter (eg, thrombi, fat globules, tissue debris) may enter the CPB circuit via the cardiotomy suction line. Although filters are often used at other locations, a final, in-line, arterial filter (27-40 μm) helps to reduce systemic embolism. Once filtered, the propelled blood returns to the patient, usually via a cannula in the ascending aorta, or less commonly in the femoral artery. A normally functioning aortic valve prevents blood from regurgitating into the left ventricle.
The filter is always in parallel with a (normally clamped) bypass limb in case the filter becomes clogged or develops increased resistance. For the same reason, arterial inflow pressure is measured before the filter. The filter is also designed to trap air, which can be bled out through a built-in stopcock.
The cardiotomy suction pump aspirates blood from the surgical field during CPB and returns it directly to the main pump reservoir. This is a potential port of entry for fat and other debris to the pump that could embolize to organs. A so-called cell-saver suction device may also be used to aspirate blood from the surgical field, in which case blood is returned to a separate reservoir on a separate device. When sufficient blood has accumulated (or at the end of the procedure), the cell-saver blood is centrifuged, washed, and returned to the patient. Excessive suction pressure can theoretically contribute to red cell trauma. Use of cell-saver suction (instead of cardiotomy suction) during bypass will deplete CPB circuit volume if blood loss is brisk. The high negative pressure of ordinary wall suction devices produces excessive red cell trauma precluding blood salvage from that source.
With time, even with “total” CPB, blood reaccumulates in the left ventricle as a result of residual pulmonary flow from the bronchial arteries (which arise directly from the aorta or the intercostal arteries) or thebesian vessels (see Chapter 20), or sometimes as a result of aortic valvular regurgitation. Aortic regurgitation can occur as a result of either (structural) valvular abnormalities or surgical manipulation of the heart (functional). Distention by blood of the left ventricle compromises myocardial preservation (see below) and requires decompression (venting). Most surgeons accomplish this by inserting a catheter via the right superior pulmonary vein and left atrium into the left ventricle. Venting may also be accomplished using a catheter placed in the left ventricular apex or across the aortic valve. The blood aspirated by the vent pump normally passes through a filter before being returned to the venous reservoir.
Cardioplegic solutions are most often administered via an accessory pump on the CPB machine. This technique allows optimal control over the infusion pressure, rate, and temperature. A separate heat exchanger ensures control of the temperature of the cardioplegia solution. Less commonly, cardioplegic solutions may be infused from a cold intravenous fluid bag given under pressure or by gravity.
Ultrafiltration can be used during CPB to increase the patient’s hematocrit without transfusion. Ultrafilters consist of hollow capillary fibers that can function as membranes, allowing separation of the aqueous phase of blood from its cellular and proteinaceous elements. Blood can be diverted to pass through the fibers either from the arterial side of the main pump or from the venous reservoir using an accessory pump. Hydrostatic pressure forces water and electrolytes across the fiber membrane. Effluents of up to 40 mL/min may be removed.
Intentional hypothermia is often used following the initiation of CPB. Core body temperature may be reduced to 20-32°C. In recent years, so-called tepid bypass has been used; this may be accomplished by allowing the patient’s temperature to “drift” downward to 30-35°C. Metabolic oxygen requirements are generally halved with each reduction of 10°C in body temperature. At the end of the surgical procedure, rewarming via the heat exchanger restores normal body temperature.
For complex repairs, profound hypothermia to temperatures of 15-18°C allows total circulatory arrest for durations of as long as 60 min. During that time, both the heart and the CPB machine are stopped.
The adverse effects of hypothermia include platelet dysfunction; reversible coagulopathy; and depression of myocardial contractility.
Optimal results in cardiac surgery require an expeditious and complete surgical repair with minimal physical trauma to the heart. Meanwhile, several techniques are used to prevent myocardial damage and maintain normal cellular integrity and function during CPB. Nearly all patients sustain at least minimal myocardial injury during cardiac surgery. With good preservation techniques, however, most of the injury is reversible. Although myocardial injury can be related to the hemodynamic instability or surgical technique, it most commonly appears to be related to incomplete myocardial preservation during CPB. Injury related to hemodynamic instability results from an imbalance between oxygen demand and supply, producing cell ischemia. After ischemia, reperfusion injury may also play a role. Reperfusion following a period of ischemia may produce excess oxygen-derived free radicals, intracellular calcium overload, abnormal endothelial-leukocyte interactions, and myocardial cellular edema. Patients at greatest risk are those with poor ventricular function (as measured preoperatively) (see Table 21-13) those with ventricular hypertrophy, and those with diffuse severe coronary artery disease. Inadequate myocardial preservation is usually manifested at the end of bypass as a persistently reduced cardiac output, worsened ventricular function by TEE, or cardiac arrhythmias. Electrocardiographic signs of myocardial ischemia are often difficult to detect due to frequent use of electrical pacing. Myocardial “stunning,” resulting from ischemia and reperfusion, produces systolic and diastolic dysfunction that is reversible with time. The stunned myocardium usually responds to positive inotropic drugs. Myocardial necrosis, on the other hand, produces irreversible injury.
Aortic cross-clamping during CPB completely excludes the coronary arteries from the generalized bypass machine flow to the body, reducing coronary blood flow to 0. Although it is difficult to estimate a safe period for cross-clamping because of differing vulnerabilities among patients and differing techniques for myocardial preservation, CPB times longer than 120 min (while often unavoidable) increase risk relative to shorter bypass times. Myocardial ischemia during bypass may occur not only during aortic clamping, but also after release of the cross-clamp. Low arterial pressures, coronary embolism (from thrombi, platelets, air, fat, or atheromatous debris), reperfusion injury, coronary artery or bypass graft vasospasm, and contortion of the heart—causing compression or distortion of the coronary vessels—are all possible causes. Areas of the myocardium distal to a high-grade coronary obstruction are at greatest risk.
Ischemia causes depletion of high-energy phosphate compounds and an accumulation of intracellular calcium. When coronary blood flow ceases, creatine phosphate and anaerobic metabolism become the principal sources of cellular energy; fatty acid oxidation is impaired. Unfortunately, these energy stores rapidly become depleted, and the progressive acidosis that develops limits glycolysis.
Cardioplegic solutions maintain normal cellular integrity and function during CPB by reducing energy expenditure and preserving the availability of high-energy phosphate compounds. Although measures directed at increasing or replenishing energy substrates in the form of glucose or glutamate/aspartate infusions are used, the emphasis of myocardial preservation has been on reducing cellular energy requirements to minimal levels. This is accomplished initially by the use of potassium cardioplegia (below). The initial dose of cardioplegic solution may be hypothermic or may start warm (“hot shot”) and progress to cold. Maintenance of myocardial protection may be facilitated by systemic and topical cardiac hypothermia (ice slush). Myocardial hypothermia reduces basal metabolic oxygen consumption, and potassium cardioplegia minimizes energy expenditure by arresting both electrical and mechanical activity. Myocardial temperature is often monitored directly; 10-15°C is usually considered desirable. Cardioplegic solutions can be administered either antegrade through a catheter placed in the proximal aorta between the aortic clamp and the aortic valve, or retrograde through a catheter placed through the right atrium into the coronary sinus.
Ventricular fibrillation and distention (previously discussed) are important causes of myocardial damage. Ventricular fibrillation can dangerously increase myocardial oxygen demand, whereas distention not only increases oxygen demand but also reduces oxygen supply by interfering with subendocardial blood flow. The combination of the two is particularly bad. Other factors that might contribute to perioperative myocardial damage include the use of excessive doses of positive inotropes or calcium salts. In open heart procedures, de-airing of cardiac chambers and venting before and during initial cardiac ejection are critically important in preventing cerebral or coronary air embolism (and strokes—see below). Removing air from coronary grafts during bypass procedures is similarly important. Depending on the amount and the location of coronary emboli, even small air bubbles can cause varying degrees of ventricular dysfunction at the end of CPB. To some extent, air emboli may preferentially find their way into the right (versus left) coronary ostium because of its superior location on the aortic root in the supine patient.
The most widely used method of arresting myocardial electrical activity is the administration of potassium-rich crystalloid or blood-crystalloid solutions. Following initiation of CPB and aortic cross-clamping, the coronary circulation is perfused intermittently with (usually cold) cardioplegic solutions. The resulting increase in extracellular potassium concentration reduces the transmembrane potential. Eventually, the heart is arrested in diastole. Usually, cold cardioplegia must be repeated at intervals (about every 30 min) because of gradual washout and rewarming of the myocardium. The heart is subject to warming by contact with blood in the adjacent descending aorta and by contact with warmer ambient air in the surgical theater. Moreover, multiple doses of cardioplegia solutions may improve myocardial preservation by preventing an excessive accumulation of metabolites that inhibit anaerobic metabolism.
Although the exact recipe varies from center to center, the essential ingredient of the induction dose of cardioplegic solution is the same: an elevated potassium (10-40 mEq/L) concentration. Potassium concentration is kept below 40 mEq/L, because higher levels can be associated with an excessive potassium load and excessive potassium concentrations at the end of termination of bypass perfusion. Sodium concentration in cardioplegic solutions is usually less than in plasma (<140 mEq/L) because ischemia tends to increase intracellular sodium content. A small amount of calcium (0.7-1.2 mmol/L) is needed to maintain cellular integrity, whereas magnesium (1.5-15 mmol/L) is usually added to control excessive intracellular influxes of calcium. A buffer—most commonly bicarbonate—is necessary to prevent excessive buildup of acid metabolites; in fact, alkalotic perfusates are reported to produce better myocardial preservation. Alternative buffers include histidine and tromethamine (also known as THAM). Other components may include hypertonic agents to control cellular edema (mannitol) and agents thought to have membrane-stabilizing effects (lidocaine or glucocorticoids). Energy substrates are provided as glucose, glutamate, or aspartate. The question of whether to use crystalloid or blood as a vehicle for achieving cardioplegia remains controversial, although blood cardioplegia has become very common in North America. Evidence suggests that at least some groups of high-risk patients may do better with blood cardioplegia. Certainly, oxygenated blood cardioplegia may contain more oxygen than crystalloid cardioplegia.
Because cardioplegia may not reach areas distal to high-grade coronary obstructions (the areas that need it most), many surgeons administer retrograde cardioplegia through a coronary sinus catheter. Some centers have reported that the combination of antegrade plus retrograde cardioplegia is superior to either technique alone. Others have suggested that continuous warm blood cardioplegia is superior to intermittent hypothermic cardioplegia for myocardial preservation, but many surgeons avoid continuous cardioplegia so that they can operate in a “bloodless” surgical field. Moreover, warm cardiac surgery raises additional concerns about loss of the potentially protective effects of systemic hypothermia against cerebral injury, when true normothermia (rather than tepid bypass) is maintained.
As discussed previously, with prolonged myocardial ischemic times (cross-clamp time), reperfusion of the myocardium can lead to extensive cell injury, rapid accumulation of intracellular calcium, and potentially irreversible cellular necrosis. This process has long been attributed to depletion of endogenous free radical scavengers during CPB and accumulation of deleterious oxygen-derived free radicals. Free radical scavengers, such as mannitol, may help decrease reperfusion injury and are typical constituents of cardioplegic solutions and bypass “priming” solutions. Several steps may help limit reperfusion injury before unclamping of the aorta. Just prior to reperfusion, the heart may be perfused by a reduced potassium cardioplegic solution that serves to wash out accumulated metabolic byproducts. Alternatively, a “hot shot” or warm blood cardioplegic solution may be administered to wash out byproducts and replenish metabolic substrates. Hypercalcemia should be avoided in the immediate reperfusion period. Reperfusion pressures should be controlled closely because of altered coronary autoregulation. Systemic perfusion pressure is reduced just prior to clamp release; it is then brought up initially to about 40 mm Hg before gradually being increased and maintained at about 70 mm Hg. To further minimize metabolic requirement, the heart should have the opportunity to recover and resume contracting in an empty state for some additional time (5-10 min), and acidosis and hypoxia should be corrected before attempting to wean the patient from bypass perfusion.
Inadequate myocardial protection or inadequate washout and recovery from cardioplegia can result in asystole, atrioventricular conduction block, or a poorly contracting heart at the end of bypass. Excessive volumes of hyperkalemic cardioplegic solutions may produce persisting systemic hyperkalemia. Although calcium salt administration partially offsets hyperkalemia, excessive calcium can promote and enhance myocardial damage. In the usual patient myocardial performance improves with time as the contents of the cardioplegia are cleared from the heart.
Initiation of CPB is associated with a variable increase in stress hormones and systemic inflammatory response. Elevated levels of catecholamines, cortisol, arginine vasopressin, and angiotensin are observed. These neurohormonal responses are variously influenced by depth of anesthesia, blood pressure, type of surgical repair, or presence of pulsatile CPB.
Multiple humoral systems are also activated, including complement, coagulation, fibrinolysis, and the kallikrein system. Contact of blood with the internal surfaces of the CPB system activates complement via the alternate pathway (C3) as well as the classic pathway; the latter also activates the coagulation cascade, platelets, plasminogen, and kallikrein. Mechanical trauma from blood contact with the bypass apparatus also activates platelets and leukocytes. Increased amounts of oxygen-derived free radicals are generated. A systemic inflammatory response syndrome similar to that seen with sepsis and trauma can develop. When this response is intense or prolonged, patients can develop the same complications, including generalized edema, the acute respiratory distress syndrome, coagulopathy, and acute kidney failure.
CPB alters and depletes glycoprotein receptors on the surface of platelets. The resulting platelet dysfunction likely increases perioperative bleeding and potentiates other coagulation abnormalities (activation of plasminogen and the inflammatory response described above).
Animal and clinical research has demonstrated that the inflammatory response to CPB can be modulated by various therapies. Leukocyte depletion reduces inflammation and may similarly reduce complications. Leukocyte-depleted blood cardioplegia has been shown to improve myocardial preservation in some studies. Hemofiltration (ultrafiltration) during CPB, which presumably removes inflammatory cytokines, appears beneficial in pediatric patients. Administration of free radical scavengers such as high-dose vitamins C and E and mannitol has improved outcome in some studies. Systemic corticosteroids before and during CPB can modulate the inflammatory response during CPB but improved outcome is not well established. Two large randomized clinical trials are underway to test whether there is an outcome benefit to the routine use of systemic corticosteroids with CPB.
One once-promising agent, aprotinin, reduced inflammation and surgical bleeding following CPB. Unfortunately, it increased mortality and is no longer available in North America.
Plasma and serum concentrations of most water-soluble drugs (eg, nondepolarizing muscle relaxants) acutely decrease at the onset of CPB, but the change can be minimal and inconsequential for most lipid-soluble drugs (eg, fentanyl and sufentanil). The effects of CPB are complex because of the sudden increase in volume of distribution with hemodilution, decreased protein binding, and changes in perfusion and redistribution between peripheral and central compartments. Some drugs, such as opioids, also bind CPB components (but this is also minimal and inconsequential). Heparin potentially alters protein binding of drugs and ions by releasing and activating lipoprotein lipase, which hydrolyzes plasma triglycerides into free fatty acids; the latter can competitively inhibit drug binding to plasma proteins and bind free calcium ions. With the possible exception of propofol, constant infusion of a drug during CPB (even when adjusted to maintain a constant “effect site” concentration using data from patients not undergoing CPB) generally causes progressively increasing blood levels as a result of reduced hepatic and renal perfusion (reduced elimination) and hypothermia (reduced metabolism).
Anesthetic Management of Cardiac Surgery
The preoperative evaluation and anesthetic management of common cardiovascular diseases are discussed in Chapter 21. The same principles apply whether these patients are undergoing cardiac or noncardiac surgery. An important distinction is that patients undergoing cardiac procedures will by definition have advanced disease. Establishing the adequacy of the patient’s preoperative cardiac function should be based on exercise (activity) tolerance, measurements of myocardial contractility such as ejection fraction, the severity and location of coronary stenoses, ventricular wall motion abnormalities, cardiac end-diastolic pressures, cardiac output, and valvular areas and gradients. Fortunately, unlike noncardiac surgery, cardiac surgery improves cardiac function in the majority of patients, and these patients have usually been extensively evaluated before being offered surgical repair. The anesthetic preoperative evaluation should also include a focus on pulmonary, neurological, and renal function, as preoperative impairment of these organ systems predisposes patients to myriad postoperative complications.
The prospect of heart surgery is frightening, and relatively “heavy” oral or intramuscular premedication was often given in the past, particularly when patients had coronary artery disease with good left ventricular function (see Chapter 21). However, in current practice, most patients receive no sedative-hypnotic premedication until their arrival on the surgical unit, at which time most will receive small doses of intravenous midazolam.
Benzodiazepine sedative-hypnotics (diazepam, 5-10 mg orally), alone or in combination with an opioid (morphine, 5-10 mg intramuscularly or hydromorphone, 1-2 mg intramuscularly), were often used in the past. Longer acting premedicant agents (eg, lorazepam) are avoided by most practitioners to permit “fast tracking” of patients through their recovery.
The best practitioners of cardiac anesthesia formulate a simple anesthetic plan that includes adequate preparations for contingencies. Many patients are critically ill, and there is little time intraoperatively to have an assistant search for drugs and equipment. At the same time, the anesthetic plan should not be excessively rigid; when problems are encountered with one technique, one should be ready to change to another without delay. Preparation, organization, and attention to detail permit one to more efficiently deal with unexpected intraoperative problems. The anesthesia machine, monitors, infusion pumps, and blood warmer should all be checked before the patient arrives. Drugs—including anesthetic and vasoactive agents—should be immediately available. Many clinicians prepare one vasoconstrictor and one vasodilator infusion before the start of the procedure.
Cardiac surgery is sometimes associated with large and rapid blood loss, and with the need for multiple drug infusions. Ideally, two large-bore (16-gauge or larger) intravenous catheters should be placed. One of these should be in a large central vein, usually an internal or external jugular or subclavian vein. Central venous cannulations may be accomplished while the patient is awake but sedated or after induction of anesthesia. Studies show no benefit from placing either central venous or pulmonary arterial catheters in awake (versus anesthetized) patients undergoing cardiovascular surgery.
Drug infusions should ideally be given into a central catheter, preferably directly into the catheter or into the injection port closest to the catheter (to minimize dead space). Multilumen central venous catheters and multilumen pulmonary artery catheter introducer sheaths allow for multiple drug infusions with simultaneous measurement of vascular pressures. One intravenous port should be dedicated for drug infusions and nothing else; drug and fluid boluses should be administered through another site. The side port of the introducer sheath used for a pulmonary catheter can be used for drug infusions but serves better as a fluid bolus line when a large-bore introducer (9F) is used.
Blood should be immediately available for transfusion if the patient has already had a midline sternotomy (a “redo”); in these cases, the right ventricle or coronary grafts may be adherent to the sternum and may be accidentally entered during the repeat sternotomy.
The electrocardiogram (ECG) is continuously monitored with two leads, usually leads II and V5. Baseline tracings of all leads may be recorded on paper for further reference. The advent of monitors with computerized ST-segment analysis and the use of additional monitoring leads (V4, aVF, and V4R) have greatly improved detection of ischemic episodes, as has the frequent intraoperative use of TEE.
In addition to all basic monitoring, arterial cannulation is always performed either prior to or immediately after induction of anesthesia, as the induction period represents a time when major hemodynamic alterations may occur. Radial arterial catheters may occasionally give falsely low readings following sternal retraction as a result of compression of the subclavian artery between the clavicle and the first rib. They may also provide falsely low values early after CPB due to the opening of atrioventricular shunts in the hand during rewarming. The radial artery on the side of a previous brachial artery cutdown should be avoided, because its use is associated with a greater incidence of arterial thrombosis and wave distortion. Obviously, if a radial artery will be harvested for a coronary bypass conduit, it cannot be used as a site for arterial pressure monitoring. Other useful catheterization sites include the ulnar, axillary, and especially brachial and femoral arteries. A backup manual or automatic blood pressure cuff should also be placed on the opposite side for comparison with direct measurements.
Central venous pressure is not terribly useful for diagnosis of hypovolemia but has been customarily monitored in nearly all patients undergoing cardiac surgery. The decision about whether to use a pulmonary artery catheter is based on the patient, the procedure, and the preferences of the surgical team. Routine use of a pulmonary artery catheter, once nearly universal in adult cardiovascular practice, is controversial. Pulmonary artery catheterization has declined precipitously in nearly all circumstances except adult cardiac surgery due to lack of evidence of a positive effect on patient outcomes. Left ventricular filling pressures can be measured with a left atrial pressure line inserted by the surgeon during bypass. In general, pulmonary artery catheterization has been most often used in patients with compromised ventricular function (ejection fraction <40-50%) or pulmonary hypertension and in those undergoing complicated procedures. The most useful data are pulmonary artery pressures, the pulmonary artery occlusion (“wedge”) pressure, and thermodilution cardiac outputs. Specialized catheters provide extra infusion ports, continuous measurements of mixed venous oxygen saturation and cardiac output, and the capability for right ventricular or atrioventricular sequential pacing. Given the risk associated with placing any pulmonary artery catheter, some clinicians opine that it makes sense to restrict pulmonary artery catheterization only to devices that offer these advanced capabilities.
The right internal jugular vein is the preferred approach for intraoperative central venous cannulation. Catheters placed through the other sites, particularly on the left side, are more likely to kink following sternal retraction (above) and are not nearly as likely to pass into the superior vena cava as those placed through the right internal jugular vein.
Pulmonary artery catheters migrate distally during CPB and may spontaneously wedge without balloon inflation. Inflation of the balloon under these conditions can rupture a pulmonary artery causing lethal hemorrhage. Pulmonary artery catheters should be routinely retracted 2-3 cm during CPB and the balloon subsequently inflated slowly. If the catheter wedges with less than 1.5 mL of air in the balloon, it should be withdrawn farther.
Once the patient is anesthetized, an indwelling urinary catheter is placed to monitor the hourly output. Bladder temperature is often monitored as a measure of core temperature but may not track core temperature well with reduced urinary flow. The sudden appearance of reddish urine may indicate excessive red cell hemolysis caused by CPB or a transfusion reaction.
Multiple temperature monitors are usually placed once the patient is anesthetized. Bladder (or rectal), esophageal, and pulmonary artery (blood) temperatures are often simultaneously monitored. Because of the heterogeneity of readings during cooling and rewarming, bladder and rectal readings are generally taken to represent an average body temperature, whereas esophageal represents core temperature. Pulmonary artery temperature provides an accurate estimate of blood temperature, which should be the same as core temperature in the absence of active cooling or warming. Nasopharyngeal and tympanic probes may most closely approximate brain temperature. Myocardial temperature is often measured directly during CPB.
Intraoperative laboratory monitoring is mandatory during cardiac surgery. Blood gases, hematocrit, serum potassium, ionized calcium, and glucose measurements should be immediately available. The activated clotting time (ACT) approximates the Lee-White clotting time and is used to monitor heparin anticoagulation. Some centers routinely use thromboelastography (TEG) to identify causes of bleeding after CPB.
One of the most important actions in intraoperative monitoring is inspection of the surgical field. Once the sternum is opened, lung expansion can be observed through the pleura. When the pericardium is opened, the heart (primarily the right ventricle) is visible; thus cardiac rhythm, volume, and contractility can often be judged visually. Blood loss and surgical maneuvers must be closely watched and related to changes in hemodynamics and rhythm.
TEE provides valuable information about cardiac anatomy and function during surgery. Two-dimensional, multiplane TEE can detect regional and global ventricular abnormalities, chamber dimensions, valvular anatomy, and the presence of intracardiac air. Three-dimensional TEE provides a more complete description of valvular anatomy and pathology. TEE can also be helpful in confirming cannulation of the coronary sinus for cardioplegia. Multiple views should be obtained from the upper esophagus, mid-esophagus, and transgastric positions in the transverse, sagittal, and in-between planes (Figure 22-2). The two views most commonly used for monitoring during cardiac surgery are the four-chamber view (Figure 22-3) and the transgastric (short-axis) view (Figure 22-4). Three-dimensional echocardiography offers great promise for better visualization of complex anatomic features, particularly of cardiac valves. The following represent the most important applications of intraoperative TEE.
Figure 22-2
Useful views during transesophageal echocardiography. A: The relationship between the angle of the ultrasound beam and image orientation relative to the patient. B-D: Echocardiographic views from the upper mid-esophagus, lower mid-esophagus, and transgastric position (C). Note that different views can be obtained in each position as the tip of the probe is tilted either upward (anteflexion) or backward (retroflexion) and the angle of the beam is changed from 0° to 180°. The angle of the beam is shown in the upper left-hand corner of each image. The probe is also rotated clockwise or counterclockwise to optimize viewing of the various structures. AO, aorta; AV, aortic valve; CS, coronary sinus; IVC, inferior vena cava; LA, left atrium; LAA, left atrial appendage; LUPV, left upper pulmonary vein; LV, left ventricle; MPA, main pulmonary artery; MV, mitral valve; PA, pulmonary artery; RA, right atrium; RPA, right pulmonary artery; RV, right ventricle; SVC, superior vena cava.
Valvular morphology can be assessed by multiplane and three-dimensional TEE. Pressure gradients, area and severity of stenosis, and severity of valvular regurgitation can be assessed by Doppler echocardiography and color-flow imaging (Figure 22-5). Colors are usually adjusted so that flow toward the probe is red and flow in the opposite direction is blue. TEE also can detect prosthetic valve dysfunction, such as obstruction or regurgitation, and can detect vegetations from endocarditis. The TEE images in the upper mid-esophagus at 40-60° and 110-130° are useful for examining the aortic valve and ascending aorta (Figure 22-6). The valve annular diameter can also be estimated with reasonable accuracy. Doppler flow across the aortic valve must be measured looking up from the deep transgastric view (Figure 22-7). The anatomic features of the mitral valve relevant to TEE are shown in Figure 22-8. The mitral valve is examined from the mid-esophageal position, looking at the mitral valve apparatus with and without color in the 0° through 150° views (Figure 22-9). TEE is an invaluable aid to guide and assess the quality of mitral valve repair surgery. The commissural view (at about 60°) is particularly helpful because it cuts across many scallops of the mitral valve.
Figure 22-5
Transesophageal echocardiography Doppler and color-flow imaging. Pulse-wave Doppler recording of mitral valve inflow showing two phases, E (early filling) and A (atrial filling) (A). Color-flow imaging demonstrates backward flow (regurgitant jet) across the mitral valve during systole (mitral regurgitation) (B).
Figure 22-8
The anatomy of the mitral valve and its anatomic relationships to the aortic valve and left circumflex coronary artery. The posterior leaflet has three scallops, P1, P2, and P3. The anterior leaflet is usually divided into A1 and A2 regions; in some classifications the anterior leaflet is divided into three areas (A1, A2, A3), corresponding to the opposing corresponding areas of the posterior leaflet.
Ventricular function can be assessed by global systolic function, estimated by means of ejection fraction (often calculated using Simpson’s method of disks) and left ventricular end-diastolic volume; diastolic function (ie, looking for abnormal relaxation and restrictive diastolic patterns by checking mitral flow velocity or by measuring movements of the mitral valve annulus using tissue Doppler techniques); and regional systolic function (by assessing wall motion and thickening abnormalities). Regional wall abnormalities from myocardial ischemia often appear before ECG changes. Regional wall motion abnormalities can be classified into three categories based on severity (Figure 22-10): hypokinesis (reduced wall motion), akinesis (no wall motion), and dyskinesis (paradoxical wall motion). The location of a regional wall motion abnormality can indicate which coronary artery is experiencing reduced flow. The left ventricular myocardium is supplied by three major arteries: the left anterior descending artery, the left circumflex artery, and the right coronary artery (Figure 22-11). The areas of distribution of these arteries on echocardiographic views are shown in Figure 22-12. The ventricular short-axis mid view at the mid-papillary muscle level contains all three blood supplies from the major coronary arteries.
Figure 22-11
Standard angiographic views of the left (A) and right (B) coronary arteries. Note the left main coronary artery quickly divides into the left anterior descending and the left circumflex arteries. A: (1) Left anterior descending artery with septal branches; (2) ramus medianus; (3) diagonal artery; (4) first septal branch; (5) left circumflex artery; (6) left atrial circumflex artery; (7) obtuse marginal artery. B: (1) Conus artery; (2) SA node artery; (3) acute marginal artery; (4) posterior descending artery with septal branches; (5) AV node artery; (6) posterior left ventricular artery.
In an adult undergoing elective cardiac surgery TEE can help diagnose previously undetected congenital defects such as an atrial or ventricular septal defect; pericardial diseases such as pericardial effusions and constrictive pericarditis; and cardiac tumors. Doppler color-flow imaging helps delineate abnormal intracardiac blood flows and shunts. TEE can assess the extent of myomectomy in patients with hypertrophic cardiomyopathy (idiopathic hypertrophic subaortic stenosis). Upper-, mid-, and lower-esophageal views are valuable in diagnosing aortic disease processes such as dissection, aneurysm, and atheroma (Figure 22-13). The extent of dissections in the ascending and descending aorta can be accurately defined; however, airway structures prevent complete visualization of the aortic arch. The presence of protruding atheroma in the ascending aorta increases the risk of postoperative stroke and should prompt the use of epiaortic scanning to identify an atheroma-free cannulation site or a change in surgical plans.
Air is introduced into the cardiac chambers during all “open” heart procedures, such as valve surgery. Residual amounts of air often remain in the left ventricular apex even after the best deairing maneuvers. TEE is helpful in defining the volume of residual air, to determine whether additional surgical maneuvers need to be undertaken to help avoid cerebral or coronary embolism.
Computer-processed electroencephalographic (EEG) recordings can be used to assess anesthetic depth during cardiac surgery, and either the processed or “raw” EEG can be used to ensure complete drug-induced electrical silence (for brain protection) prior to circulatory arrest. These recordings are generally not useful in detecting neurological insults during CPB. Progressive hypothermia (or progressively deepened anesthesia) is typically associated with EEG slowing, burst suppression, and, finally, an isoelectric recording. Most strokes during CPB are due to small emboli that are not likely to produce changes in the EEG. Artifacts from the CPB roller pump may be seen on the raw EEG (due to piezoelectric effects from compression of the pump tubing) but can usually be identified as such by computer processing.
This modality provides noninvasive measurements of blood flow velocity in the middle cerebral artery, which is insonated through the temporal bone. TCD is useful for detecting cerebral emboli. Increased numbers of emboli detected by TCD or Doppler interrogation of the carotid artery have been associated with an increased risk of postoperative neurobehavioral dysfunction.
Cardiac operations usually require general anesthesia, endotracheal intubation, and controlled ventilation. Some centers have used thoracic epidural anesthesia alone for minimally invasive surgery without CPB or combined thoracic epidural with light general endotracheal anesthesia for other forms of cardiac surgery. These techniques have never been popular in North America due to concerns about the risk of spinal hematomas following heparinization, the associated medical-legal consequences, and the limited evidence of an outcome benefit. Other centers use a single intrathecal morphine injection to provide postoperative analgesia.
For elective procedures, induction of general anesthesia should be performed in a smooth, controlled (but not necessarily “slow”) fashion often referred to as a cardiac induction. The principles are discussed in Chapter 21. Selection of anesthetic agents is generally less important than the way they are used. Indeed, studies have failed to show differences in long-term outcome with various anesthetic techniques. Anesthetic dose requirements are variable and patient tolerance of inhaled anesthetics generally declines with declining ventricular function. Severely compromised patients should be given anesthetic agents in incremental, small doses. A series of challenges may be used to judge when anesthetic depth will allow intubation without a marked hypertensive response, while also avoiding hypotension from excessive anesthetic dosing. Blood pressure and heart rate are continuously evaluated following unconsciousness, insertion of an oral airway, urinary catheterization, and tracheal intubation. A sudden increase in heart rate or blood pressure may indicate light anesthesia and the need for more anesthetic prior to the next challenge, whereas a decrease or no change suggests that the patient is ready for the subsequent stimulus. Muscle relaxant is given after consciousness is lost. Reductions in blood pressure greater than 20% generally call for administration of a vasopressor (see below).
The period following intubation is often characterized by a gradual decrease in blood pressure resulting from the anesthetized state (often associated with vasodilation and decreased sympathetic tone) and a lack of surgical stimulation. Patients will usually respond to fluid boluses or a vasoconstrictor. Nevertheless, the administration of large amounts of intravenous fluids prior to the bypass may serve to accentuate the hemodilution associated with CPB (below). Small doses of phenylephrine (25-100 mcg), vasopressin (1-3 units), or ephedrine (5-10 mg) may be useful to avoid excessive hypotension. Following intubation and institution of controlled ventilation; arterial blood gases, hematocrit, serum potassium, and glucose concentrations are measured. The baseline ACT (normal <130 s) is best measured after skin incision.
Anesthetic techniques for cardiac surgery have evolved over the years. Successful techniques range from primarily volatile inhalation anesthesia to high-dose opioid totally intravenous techniques. In recent years, total intravenous anesthesia with short-acting agents and combinations of intravenous and volatile agents have become most popular.
This technique was originally developed to circumvent the myocardial depression associated with older volatile anesthetics, particularly halothane. But pure high-dose opioid anesthesia (eg, fentanyl, 50-100 mcg/kg, or sufentanil, 15-25 mcg/kg) produces prolonged postoperative respiratory depression (12-24 h), is associated with an unacceptably high incidence of patient awareness (recall) during surgery, and often fails to control the hypertensive response to stimulation in many patients with preserved left ventricular function. Other undesirable effects include skeletal muscle rigidity during induction and prolonged postoperative ileus. Moreover, simultaneous administration of benzodiazepines with large doses of opioids can produce hypotension and myocardial depression. Patients anesthetized with sufentanil (and other shorter acting agents) generally regain consciousness and can be extubated sooner than those anesthetized with fentanyl.
The drive for cost containment in cardiac surgery was a major impetus for development of anesthesia techniques with short-acting agents. Although the drugs may be costlier, large economic benefits resulted from earlier extubation, decreased intensive care unit (ICU) stays, earlier ambulation, and earlier hospital discharge (“fast-track” management). One technique employs induction with propofol (0.5-1.5 mg/kg followed by 25-100 mcg/kg/min), and modest doses of fentanyl (total doses of 5-7 mcg/kg) or remifentanil (0-1 mcg/kg bolus followed by 0.25-1 mcg/kg/min). Target controlled infusion (TCI) employs software and hardware (computerized infusion pump) to deliver a drug and achieve a set concentration at the effect site based on pharmacokinetic modeling. For propofol the clinician sets only the patient’s age and weight, and the desired blood concentration on the Diprifusor, a TCI device widely available in countries outside North America. During cardiac surgery, this technique can be used for propofol with a target concentration of 1.5-2 mcg/mL. Whenever the very short-acting remifentanil is used for painful surgery, provision must be made for postoperative analgesia after its discontinuation.
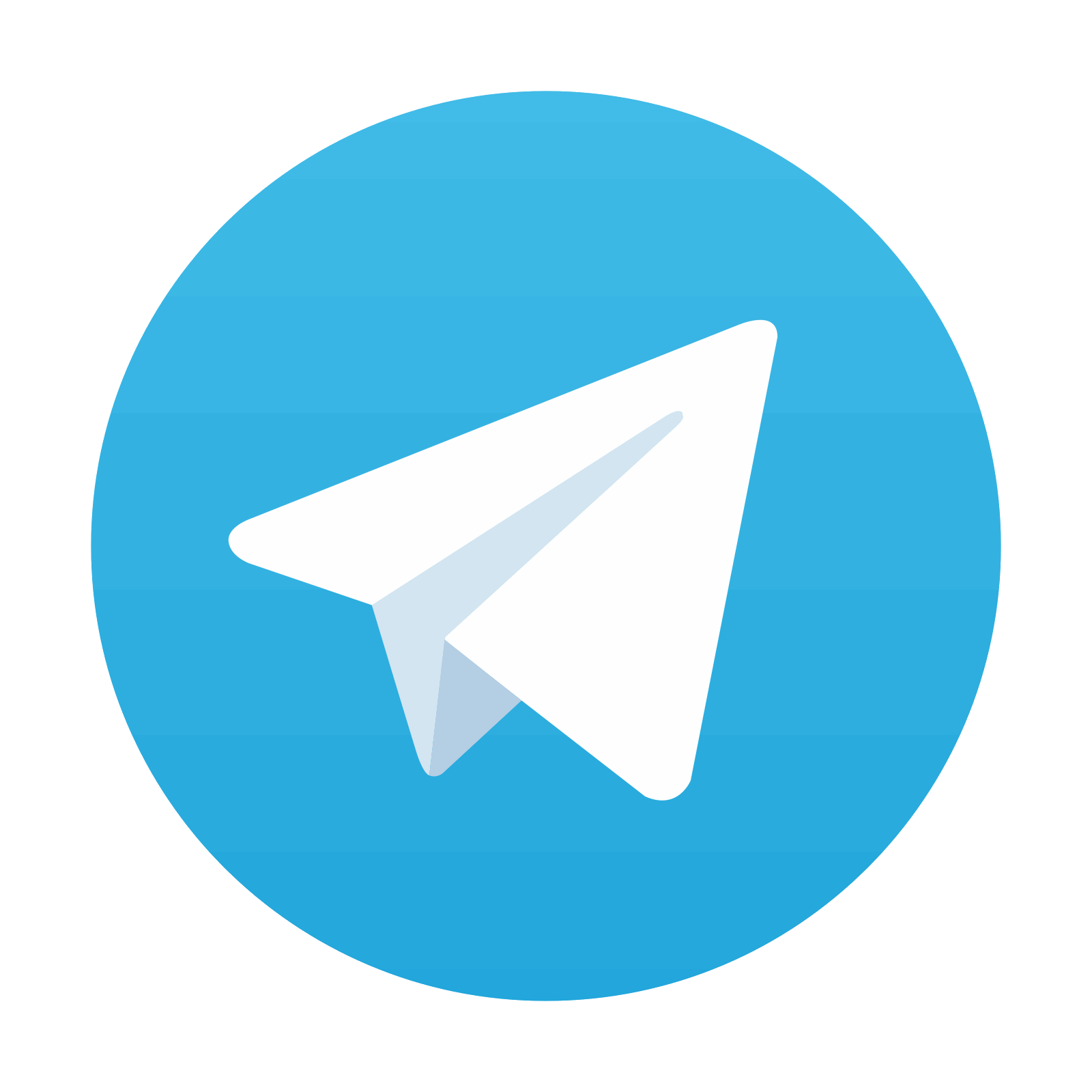
Stay updated, free articles. Join our Telegram channel

Full access? Get Clinical Tree
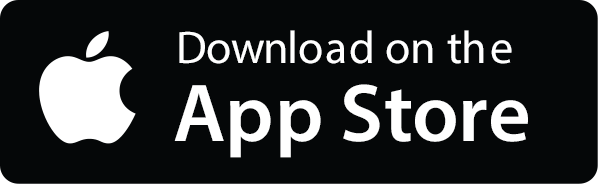
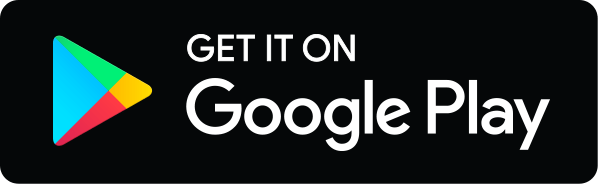