Sepsis and its complications continue to impose a significant public heath burden in terms of their high morbidity and mortality, reduced quality of life in survivors, and overall financial burden to the health care system. Although the last 15 years have been characterized by significant progress in combating sepsis, many challenges remain. Notwithstanding the significant advances in sepsis biology over the last three decades, major impediments to improving the care of patients with sepsis include (1) a relatively poor understanding of the pathophysiology of sepsis, (2) lack of accurate diagnostics, and (3) the failure of all studies to date to identify a therapeutic molecular target. This chapter focuses on the state of the art in the clinical management of sepsis and its complications, while also highlighting innovative diagnostic and therapeutic approaches to this complex problem.
I. DEFINITIONS: Sepsis traditionally has been defined as the host’s systemic response to an infection or, as defined by the International Sepsis Forum (2010), is “a life-threatening condition that arises when the body’s response to an infection injures its own tissues and organs.” In 1991, a North American consensus conference introduced criteria for sepsis phenotypes, including systemic inflammatory response syndrome (SIRS), sepsis, severe sepsis, septic shock, and multiple organ dysfunction syndrome that continue to be used today (Table 29.1). A second consensus conference in 2001 attempted to improve upon these definitions, but without notable success, and the 1991 definitions continue to be used by most clinicians. These definitions have a number of drawbacks. They are extremely sensitive (a common viral illness would satisfy the definition of sepsis if it causes fever and tachycardia), a “host response” is one of the characteristics of any infection (the absence of a host response in an immunocompetent individual suggests colonization, not infection) and these definitions do not clarify the role of infection in the pathogenesis of SIRS, since sterile inflammation (such as trauma, acute pancreatitis, burns, etc.) can present with clinical features identical to severe sepsis. In fact, the use of two or more SIRS criteria to define severe sepsis in a recent study failed to identify one in eight otherwise similar patients and failed to define a transition point in the risk of death (mortality increased linearly with each additional SIRS criterion).
II. EPIDEMIOLOGY: Recent data suggest that the incidence of sepsis and the number of sepsis-related deaths are increasing, although the overall mortality rate among patients with sepsis is declining. The declining mortality rate is related, at least in part, to increased awareness and early effective management of patients with sepsis, but may also reflect an increase in the overall number of reported cases of sepsis with the inclusion of more patients with less severe disease. While the precise incidence of sepsis in the United States is unclear, studies suggest a steady increase in incidence (between 8.7% and 13% per year) over the last few decades. As mentioned above, in-hospital mortality has declined (from 35% in 2004 to 26% in 2009), although patients with severe sepsis and septic shock have significantly higher mortality rates. Estimates of the economic impact of sepsis on the U.S. health care system range from $17 to $26 billion per year.
| 1991 ACCP/SCCM Consensus Definitions of Sepsis and Its Complications | |
Infection: Microbial phenomenon characterized by an inflammatory response to the presence of microorganisms or the invasion of normally sterile host tissue by those organisms
Bacteremia: The presence of viable bacteria in the blood
Systemic inflammatory response syndrome: The systemic inflammatory response to a variety of severe clinical insults. The response is manifested by two or more of the following conditions:
Temperature >38°C or <36°C
Heart rate >90 beats/min
Respiratory rate >20 breaths/min or PaCO2 <32 torr (<4.3 kPa)
WBC >12,000 cells/mm3, <4,000 cells/mm3, or >10% immature (band) forms
Sepsis: Systemic inflammatory response syndrome caused by a suspected or proven infection. This systemic response is manifested by two or more of the following conditions as a result of infection:
Temperature >38°C or <36°C
Heart rate >90 beats/min
Respiratory rate >20 breaths/min or PaCO2 <32 torr (<4.3 kPa)
WBC >12,000 cells/mm3, <4,000 cells/mm3, or >10% immature (band) forms
Severe sepsis:` Sepsis associated with organ dysfunction, hypoperfusion, or hypotension. Hypoperfusion and perfusion abnormalities may include, but are not limited to, lactic acidosis, oliguria, or an acute alteration in mental status
Septic shock: Sepsis with hypotension, despite adequate fluid resuscitation, along with the presence of perfusion abnormalities that may include, but are not limited to, lactic acidosis, oliguria, or an acute alteration in mental status. Patients who are on inotropic or vasopressor agents may not be hypotensive at the time that perfusion abnormalities are measured
Hypotension: A systolic BP of <90 mmHg or a reduction of >40 mmHg from baseline in the absence of other causes for hypotension
Multiple organ dysfunction syndrome: Presence of altered organ function in an acutely ill patient such that homeostasis cannot be maintained without intervention
Adapted from Bone RC, Balk RA, Cerra FB, et al. American College of Chest Physicians/Society of Critical Care Medicine Consensus Conference: definitions for sepsis and organ failure and guidelines for the use of innovative therapies in sepsis. Crit Care Med 1992;20:864–74, with permission.
III. PATHOPHYSIOLOGY
A. Host–Pathogen–Microbiome–Hospital Interactions: Humans harbor an extraordinarily diverse microbial ecosystem that has coevolved with our species and that has increasingly being recognized as essential for health. This “extended genome” of millions of microbial genes is referred to as the microbiome. As we learn more about the microbiome, it is becoming evident that pathogenic bacteria, physiologic derangements associated with critical illness, as well as therapeutic agents common to health care environments (such as antibiotics) can all significantly influence the microbiome, with important consequences for critical illness. For example, alterations in the composition and function of the gut microbiome (e.g., increasing colonization of C. difficile) may influence the course of critical illness and validate the decade-old hypothesis that “the gut is the driver of multiple organ dysfunction.” In addition to genetic factors, age, comorbid medical conditions, and current medications may substantially affect the severity of illness (e.g., gut decontamination contributing to multidrug-resistant organisms). While host genetic diversity and gene–environment interactions are no doubt critical to the sepsis response, we should not underestimate the impact of the health care environment. Infection control measures (including measures as simple as hand hygiene) have been shown to dramatically impact infectious disease transmission rates within health care environments and can achieve lasting improvement in patient outcomes. Sometimes, the best defense against complex diseases is to rigorously enforce simple practices that reduce the likelihood of initiating the infectious–inflammatory cascade.
B. Initiation of the Acute Inflammatory Response:
1. PAMPs and DAMPs: Pathogen-associated molecular patterns (PAMPs) refer to evolutionarily conserved components of microorganisms that are recognized by the host innate immune system, such as lipopolysaccharide (LPS, or endotoxin) that is a component of the cell membranes of gram-negative bacteria. Gram-positive and gram-negative bacteria, fungi, viruses, and protozoa have multiple PAMPs that come into contact with the host immune system and initiate a “preprogrammed” inflammatory response. Likewise, tissue or cellular damage provokes the release of endogenous molecules (such as mitochondrial DNA or High Mobility Group Box 1 [HMGB1] protein) that are also highly effective at binding to molecular patterns that trigger an innate immune response. These molecules are known as damage-associated molecular patterns (DAMPs) or alarmins. The fact that multiple pathogens, as well as sterile tissue injury, can activate a strikingly similar immune response partly accounts for the fact that the initial presentation of severe sepsis as well as severe noninfectious insults produces similar clinical manifestations (a.k.a., SIRS).
2. The host innate immune system: The last two decades have seen an explosion in our understanding of the innate immune system. Unlike the adaptive immune system, the innate immune system does not require prior exposure to a pathogen to be able to respond to it. The innate immune system consists principally of four receptor systems: the toll-like receptors (TLRs), C-type lectin receptors, retinoic acid inducible gene-1-like receptors, and nucleotide-binding oligomerization domain–like receptors
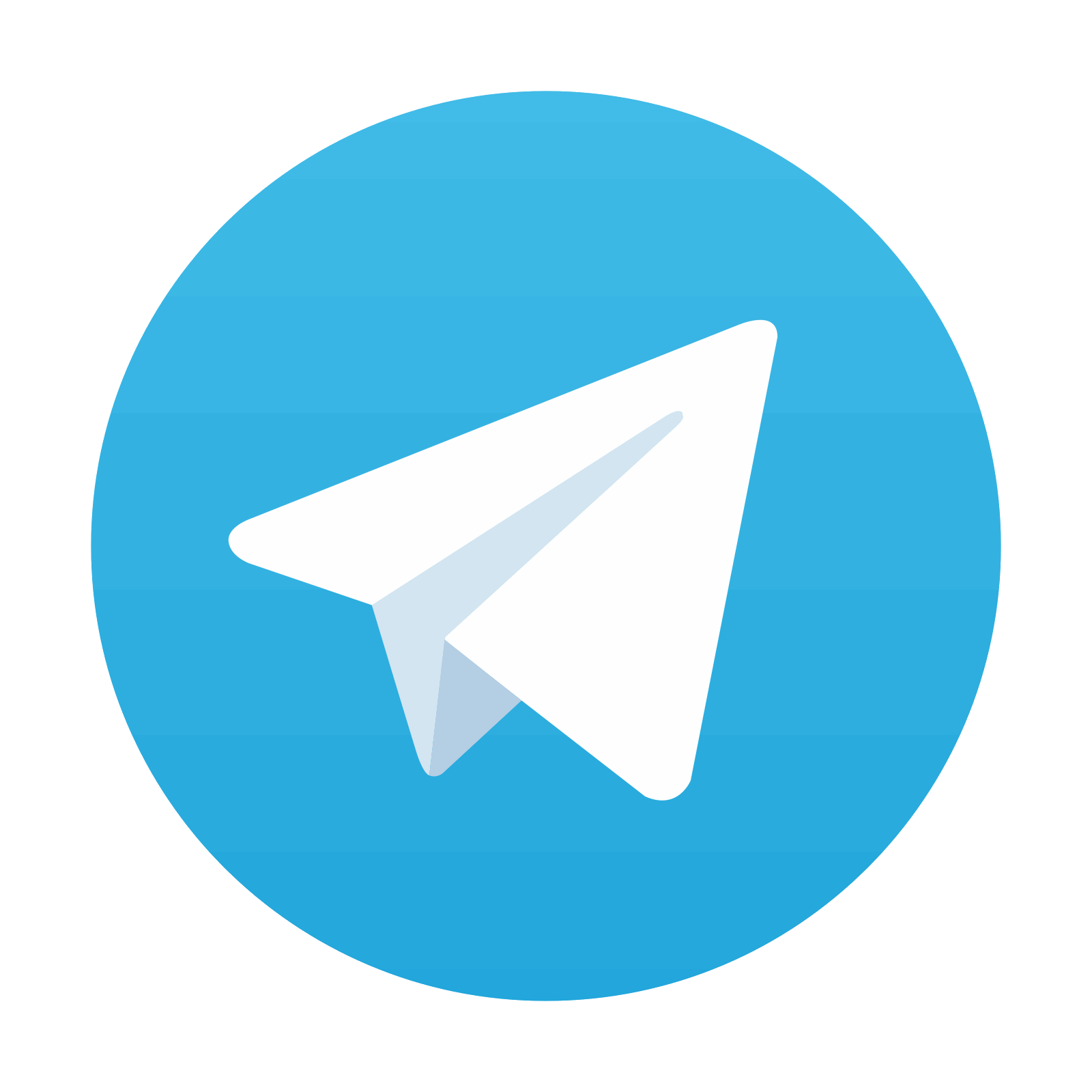
Stay updated, free articles. Join our Telegram channel

Full access? Get Clinical Tree
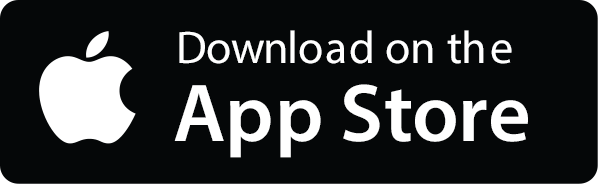
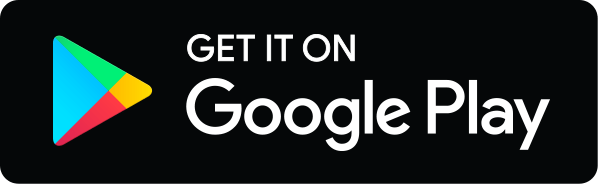