Dosing by Body Weight
Aminoglycoside dosing is based on ideal body weight (see Appendix 2 for charts of ideal body weights). For obese patients, dosing should be based on an adjusted body weight (ABW), which is equivalent to the ideal body weight (IBW) plus 45% of the difference between the total body weight (TBW) and ideal body weight (1); i.e.,
(52.1)
Dosing by Renal Function
The aminoglycosides are cleared by filtration in the kidneys, and dose adjustments are necessary when renal clearance is impaired. Note in Table 52.1 that the strength of the dose is progressively decreased with decreasing creatinine clearance, and at a creatinine clearance below 40 mL/min, the dosing interval increases to 48 hours. (Formulas for estimating the creatinine clearance are in the Appendix at the end of the book.) Hemodialysis for 6 hours will eliminate about 40–50% of the accumulated aminoglycoside, so 50% of a full dose should be given after each dialysis session (1).
Monitoring
Serum aminoglycoside levels should be monitored to determine if dosing is appropriate in individual patients. Target peak levels are 4–8 mg/L for gentamicin and tobramycin, and 15–20 mg/L for amikacin (4). Target trough levels (obtained just before a dose) are 1–2 mg/L for gentamicin and tobramycin, and 5–10 mg/L for amikacin (4).
FIGURE 52.1 Antibiotic susceptibilities of Gram-negative aerobic bacilli isolated from abdominal infections in 37 hospitals in North America from 2005 to 2010. Data from Reference 2.
Adverse Effects
Nephrotoxicity is the principal adverse effect of aminoglycosides.
Nephrotoxicity
Aminoglycosides have been called obligate nephrotoxins because renal impairment will eventually develop in all patients if treatment is continued (5). The site of renal injury is the proximal tubules, and the risk of renal injury is the same with each of the aminoglycosides. The earliest signs of injury include cylindrical casts in the urine, proteinuria, and inability to concentrate urine (3). The urinary changes appear in the first week of drug treatment, and the serum creatinine begins to rise 5–7 days after the start of therapy. The renal impairment can progress to acute renal failure, which is usually reversible. Nephrotoxic effects are enhanced by hypovolemia and pre-existing renal disease (5,6).
Other Adverse Effects
Other adverse effects, which include ototoxicity and neuromuscular blockade, are rarely a problem. The ototoxicity can produce irreversible hearing loss and vestibular damage, but these changes are usually asymptomatic (5). Aminoglycosides can block acetylcholine release from presynaptic nerve terminals, but this is never clinically apparent with therapeutic dosing (4). There is a small risk that aminoglycosides will aggravate the neuromuscular blockade associated with myasthenia gravis and nondepolarizing muscle relaxants (7,8), and it is wise to avoid aminoglycosides in these conditions.
FIGURE 52.2 Susceptibility of Pseudomonas aeruginosa to selected antibiotics. Data from a survey of abdominal infections in 37 hospitals in North America from 2005 to 2010. From Reference 2.
Comment
Aminoglycosides were once the darlings of the infectious disease community because they were the first antibiotics capable of treating serious Gram-negative infections. However, because of their nephrotoxicity, and the availability of less harmful drugs capable of treating serious Gram-negative infections, aminoglycosides should be reserved for Pseudomonas bacteremias in patients with neutropenia or septic shock.
ANTIFUNGAL AGENTS
Antifungal therapy in the critical care setting is primarily directed against Candida species, and this is the focus of the following presentation.
Amphotericin B
Amphotericin B (AmB) is a naturally occurring antibiotic that is fungicidal for most of the pathogenic fungi in humans (9). It is one of the most effective antifungal agents available, but is plagued by toxic reactions; i.e., infusion-related inflammatory responses, and nephrotoxicity. As a result, AmB is used mostly as a backup, for patients who are intolerant of, or refractory to, less toxic antifungal drugs (see Table 52.2) (10).
Dosing Regimen
AmB is available for intravenous use only, and contains a vehicle (sodium deoxycholate) to enhance solubility in plasma. It is given once daily in a dose of 0.5–1 mg/kg. The dose is initially delivered over a 4-hour time period, but can be delivered in one hour, if tolerated. Daily infusions are continued until a specified cumulative dose is achieved. The total AmB dose is determined by the type and severity of the fungal infection: it can be as little as 500 mg (for catheter-related candidemia) or as much as 4 grams (for life-threatening invasive aspergillosis).
Infusion-Related Inflammatory Response
Infusions of AmB are accompanied by fever, chills, nausea, vomiting, and rigors in about 70% of instances (11). This reaction is most pronounced with the initial infusion, and often diminishes in intensity with repeated infusions. The following measures are used to reduce the intensity of this reaction (11):
1. Thirty minutes before the infusion, give acetaminophen (10–15 mg/kg orally) and diphenhydramine (25 mg orally or IV). If rigors are a problem, premedicate with meperidine (25 mg IV).
2. If the premedication regimen does not give full relief, add hydrocortisone to the AmB infusate (0.1 mg/mL).
Central venous cannulation is preferred for AmB infusions to reduce the risk of infusion-related phlebitis, which is common when AmB is infused through peripheral veins (9).
Nephrotoxicity
AmB binds to cholesterol on the surface of renal epithelial cells and produces an injury that clinically resembles a renal tubular acidosis (distal type), with increased urinary excretion of potassium and magnesium (12). Azotemia is reported in 30–40% of patients during daily infusions of AmB (13), and can occasionally progress to acute renal failure that requires hemodialysis (14). The renal impairment from AmB usually stabilizes with continued infusions, and improvement is expected if AmB is discontinued. Hypovolemia aggravates the renal injury, and maintaining intravascular volume is important for mitigating the injury. An increase in the serum creatinine above 3.0 mg/dL should prompt cessation of AmB infusions for a few days (11).
ELECTROLYTE ABNORMALITIES: Hypokalemia and hypomagnesemia are common during AmB therapy, and the hypokalemia can be difficult to correct until the magnesium deficits are replaced (as described in Chapter 37). Oral magnesium (300–600 mg elemental magnesium daily) is recommended during AmB therapy, except for patients with progressive azotemia.
Lipid Preparations
Specialized lipid preparations of AmB have been developed to enhance AmB binding to fungal cell membranes and reduce binding to mammalian cells (thereby reducing the risk of renal injury). There are 2 lipid preparations, liposomal amphotericin, and amphotericin B lipid complex, and the recommended dose is 3–5 mg/kg daily (10). Both reduce the incidence of nephrotoxic reactions, and the decrease is greater with the liposomal preparation (15). Both preparations are costly.
Triazoles
The triazoles are synthetic antifungal agents that are less toxic alternatives to AmB for selected fungal infections. There are 3 drugs in this class, fluconazole, itraconazole, and voriconazole, but fluconazole is the one used for Candida infections.
Clinical Uses
Fluconazole is the drug of choice for infections involving Candida albicans, C. tropicalis, and C. parapsilosis, but not for infections involving C. glabrata or C. krusei (see Table 52.2) (10).
Table 52.2 Antifungal Therapy for Invasive Candidiasis
Dosing Regimens
Fluconazole can be given orally or intravenously. The usual dose is 400 mg daily, given as a single dose. Doses of 800 mg daily are recommended for clinically unstable patients. The time to reach steady state levels after the start of therapy is 4–5 days, and this can be shortened by doubling the initial dose. Adjustments are necessary for renal impairment: if the creatinine clearance is <50 mL/min, the dose should be reduced by 50% (9).
Drug Interactions
The triazoles inhibit the cytochrome P450 enzyme system in the liver, and they can potentiate the activity of several drugs. For fluconazole, the significant interactions include phenytoin, cisapride, and the statins (lovastatin, atorvastatin) (9).
Toxicity
Fluconazole is largely devoid of serious toxicity. Asymptomatic elevation of liver enzymes has been reported (9), and there are rare reports of severe and even fatal hepatic necrosis during fluconazole therapy in HIV patients (16).
Echinocandins
The echinocandins are antifungal agents that are active against a broader spectrum of Candida species than fluconazole (except C. parapsilosis) (10). The drugs in this class include caspofungin, micafungin, and anidulafungin. These agents can be used as alternatives to fluconazole for treating invasive candidiasis involving C. albicans and C. tropicalis, and are the preferred agents for infections involving C. glabrata and C. krusei (10). They are also preferred by some for prophylaxis of invasive candidiasis in unstable or immunocompromised patients (10).
Caspofungin
Caspofungin is the flagship drug in this class, and is equivalent to amphotericin for treating invasive candidiasis (17). The drug is given intravenously, and the usual IV dose is 70 mg initially, then 50 mg daily thereafter. Like all echinocandins, no dose adjustment is needed for renal insufficiency (18).
Others
Anidulafungin (200 mg IV on day 1, then 100 mg IV daily) and micafungin (100 mg IV daily) are equivalent to caspofungin (10), but there is much less clinical experience with these drugs.
Toxicity
The echinocandins are relatively non-toxic. Transient elevations in liver enzymes can occur, and there are occasional reports of hepatic dysfunction associated with these drugs (18).
CARBAPENEMS
The carbapenems have the broadest spectrum of antibacterial activity of any class of antibiotics currently available. There are 4 carbapenems available for clinical use: imipenem, meropenem, doripenem, and ertapenem. Some notable features of these drugs are included in Table 52.3. The following description is limited to imipenem and meropenem, which are currently the most popular carbapenems. Doripenem is largely indistinguishable from meropenem, and ertapenem is not active against Pseudomonas aeruginosa, which makes it the least desirable carbapenem for critically ill patients.
Table 52.3 The Carbapenems
Spectrum of Activity
Imipenem and meropenem are active against all common bacterial pathogens except methicillin-resistant staphylococci (MRSA) and vancomycin-resistant enterococci (19). As demonstrated in Figures 52.1 and 52.2, imipenem is one of the most active agents for aerobic Gram-negative bacilli, and is also active against Pseudomonas aeruginosa (although less so). These agents also provide adequate coverage for pneumococci, methicillin-sensitive staphylococci, coagulase-negative staphylococci, and anaerobes, including Bacteroides fragilis and Enterococcus faecalis. Furthermore, acquired resistance to the carbapenems has increased much less than with other antimicrobials used in critical care (19).
Clinical Uses
Because of their broad spectrum of activity, imipenem and meropenem are well suited for empiric antibiotic coverage of suspected Gram-negative infections (e.g., abdominal infections), or mixed aerobic/anaerobic infections (e.g., pelvic infections). Imipenem has also been effective as single-agent empiric coverage for neutropenic patients with fever (20). Meropenem readily crosses the blood-brain barrier (21), and can be used for empiric antibiotic coverage of suspected Gram-negative meningitis.
Dosing Regimens
The carbapenems can only be given intravenously. Imipenem is inactivated by enzymes on the luminal surface the proximal renal tubules, so it is impossible to achieve high levels of the drug in urine. To overcome this problem, the commercial preparation of imipenem contains an enzyme inhibitor, cilastatin (22). The combination imipenem–cilastatin preparation is available as Primaxin. The usual dose of imipenem–cilastatin is 500 mg IV every 6 hours. In suspected Pseudomonas infections, the dose is doubled to 1 g every 6 hours. In renal failure, the dose should be reduced by 50–75% (23).
Meropenem does not require the cilastatin addition, and the usual dose if 1 gram IV every 8 hours, which can be increased to 2 grams every 8 hours for serious infections. A 50% dose reduction is recommended in patients with renal failure (23).
Adverse Effects
The major risk associated with imipenem is generalized seizures, which has been reported in 1–3% of patients receiving the drug (22). Most of the seizure cases are in patients with a history of a seizure disorder, or an intracranial mass, who did not receive an adjusted dose of imipenem for renal insufficiency (22).
There is little risk of seizures with meropenem (19,21). However, mero-penem can reduce serum levels of valproic acid, and this can increase the risk of seizures in patients being treated with this anticonvulsant (19).
Cross-Reactivity
Patients with hypersensitivity reactions to penicillin can occasionally have a hypersensitivity reaction to carbapenems. The incidence of this cross-reactivity is not known, but the allergic reactions usually include a rash or urticaria, and are almost never life-threatening (24).
Comment
The ideal antibiotic would be effective against all pathogens and produce no adverse reactions. Meropenem comes closer to this ideal than any antibiotic currently available, with imipenem as a close second. These agents have been my personal favorites for several years because they cover almost everything, which simplifies the selection of empiric antibiotic coverage. Meropenem plus vancomycin (for MRSA) provides adequate empiric coverage for most patients in the ICU (unless there is a problem with vancomycin-resistant enterococci in your ICU). Add fluconazole for empiric coverage if invasive candidiasis is a possibility, and that should be it. The risk of seizures with imipenem is also overstated, and should not be a problem if you adjust the dose for renal insufficiency.
CEPHALOSPORINS
The first cephalosporin (cephalothin) was introduced in 1964, and this was followed by a small army of other cephalosporins. There are currently more than 20 cephalosporins available for clinical use (24,25). They are divided into generations, and some of the parenteral agents in the first four generations are shown in Table 52.4.
Table 52.4 The Generations of Parenteral Cephalosporins
Generations of Cephalosporins
The first-generation cephalosporins are primarily active against aerobic Gram-positive cocci, but are not active against Staphylococcus epidermidis or methicillin-resistant strains of S. aureus. The popular intravenous agent in this group is cefazolin (Ancef).
The second-generation cephalosporins exhibit stronger antibacterial activity against Gram-negative aerobic and anaerobic bacilli of enteric origin. The popular parenteral agents in this group are cefoxatin (Mefoxin) and cefamandole (Mandol).
The third-generation cephalosporins have greater antibacterial activity against Gram-negative aerobic bacilli, including P. aeruginosa and Hemophilus influenza, but are less active against aerobic Gram-positive cocci than the first-generation agents. The popular parenteral agents in this group are ceftriaxone (Rocephin), and ceftazidime (Fortaz). Ceftriaxone is popular for the treatment of severe community-acquired pneumonia, primarily because it is active against penicillin-resistant pneumococci and H. influenza. Ceftazidime has been a popular anti-pseudomonal antibiotic, but is being eclipsed by a drug (cefepime) in the next generation of cephalosporins.
The fourth-generation cephalosporins retain activity against Gram-negative organisms, but add some Gram-positive coverage. The only drug in this generation is cefepime (Maxipime), which has the Gram-negative antibacterial spectrum of ceftazidime (i.e., it covers P. aeruginosa), but is also active against Gram-positive cocci (e.g. streptococci and methicillin-sensitive staphylococci).
There is a fifth-generation cephalosporin, ceftaroline, that is similar in activity to the fourth generation drugs, but is also active against methicillin-resistant S. aureus (MRSA) (25). This drug is not included in Table 52.4 because there is no clinical experience with the drug in ICUs, at least at the present time.
Dosing Regimens
The doses for the more popular parenteral cephalosporins are shown in Table 52.5, along with dose adjustments for renal failure. Note that the dose in renal failure is adjusted by extending the dosing interval rather than decreasing the amount of drug given with each dose (22). This is done to preserve concentration-dependent bacterial killing. Note also that ceftriaxone requires no dose adjustment in renal failure.
Table 52.5 Intravenous Dosing for Commonly Used Cephalosporins
Toxicity
Adverse reactions to cephalosporins are uncommon and nonspecific (e.g., nausea, rash, and diarrhea). There is a 5–15% incidence of cross-antigenicity with penicillin (24), and cephalosporins should be avoided in patients with a history of serious anaphylactic reactions to penicillin.
Comment
The only cephalosporins that continue to have some value in the ICU are ceftriaxone (for empiric therapy of serious community-acquired pneumonias) and cefepime (for empiric coverage of Gram-negative enteric pathogens).
THE FLUOROQUINOLONES
The fluoroquinolone era began in the mid-1980s with the introduction of norfloxacin. Since then, several fluoroquinolones have been introduced, but only three survive: ciprofloxacin, levofloxacin, and moxifloxacin.
Changing Spectrum of Activity
The fluoroquinolones are active against methicillin-sensitive staphylococci, and the newer agents (levofloxacin and moxifloxacin) are active against streptococci (including penicillin-resistant pneumococci), and “atypical” organisms like Mycoplasma pneumoniae and Hemophilus influenza (26). When first introduced, the fluoroquinolones were very active against Gram-negative aerobic bacilli, including Pseudomonas aeruginosa, but rapidly emerging resistance has reduced their activity against Gram-negative organisms, as shown in Figures 52.1 and 52.2.
The development of resistance in Gram-negative organisms has severely curtailed the use of fluoroquinolones in the ICU. Levofloxacin and moxifloxacin are used primarily for community-acquired pneumonia, exacerbations of chronic obstructive lung disease, and for uncomplicated urinary tract infections.
Dosing Regimens
Table 52.6 shows the intravenous dosing regimens for the quinolones. The newer quinolones have longer half-lives than ciprofloxacin, and require only one once-daily dosing. Dose adjustments are required for renal failure for all but moxifloxacin, which is metabolized in the liver (26).
Table 52.6 Intravenous Dosing Regimens for Fluoroquinolones
Drug Interactions
Ciprofloxacin interferes with the hepatic metabolism of theophylline and warfarin, and can potentiate the actions of both of these drugs (27,28). Ciprofloxacin causes a 25% increase in serum theophylline levels, and combined therapy has resulted in symptomatic theophylline toxicity (29). Although no dose adjustments are necessary, serum theophylline levels and prothrombin times should be monitored carefully when cipro-floxacin is given in combination with theophylline or warfarin.
Toxicity
The fluoroquinolones are relatively safe. Neurotoxic reactions (confusion, hallucinations, seizures) can develop days after starting quinolone therapy in 1–2% of patients (30). Prolongation of the QT interval and polymorphic ventricular tachycardia (torsade de pointes) have been reported with all quinolones except moxifloxacin, but is a rare occurrence (31).
Comment
The emerging resistance of Gram-negative pathogens to fluoroquin-olones has just about eliminated fluoroquinolones from the ICU formulary. Levofloxacin is a popular antibiotic for community-acquired pneumonias, and is also used for exacerbations of COPD, but these conditions are often managed outside the ICU.
PENICILLINS
The penicillin discovered by Alexander Fleming in 1929 is benzylpenicillin, or penicillin G, which is (was) active against aerobic streptococci (S. pneumoniae, S. pyogenes) and anaerobic mouth flora. The emergence of penicillin-resistant pneumococci in recent years, along with broader spectrum drugs for treating anaerobic infections, has eliminated penicillin G from the ICU formulary.
Extended-Spectrum Penicillins
The penicillins in this category have an extended antibacterial spectrum that covers aerobic Gram-negative bacilli. This category includes the aminopenicillins (ampicillin and amoxicillin), the carboxypenicillins (carbenicillin and ticarcillin), and the ureidopenicillins (azlocillin, mezlocillin, and piperacillin). All groups are active against Gram-negative pathogens, but the latter two groups are active against Pseudomonas aeruginosa (32). These agents are also known as antipseudomonal penicillins. The most popular drug in this class is piperacillin, which is available in a special combination product (see next).
Piperacillin-Tazobactam
When used for serious Gram-negative infections, piperacillin is given in combination with tazobactam, a β-lactamase inhibitor that has synergistic activity when combined with piperacillin. The commercial product (Zosyn) contains piperacillin in an 8:1 ratio with tazobactam. The recommended dose of the combination product is 3.375 grams (3 grams piperacillin and 0.375 mg tazobactam) IV every 4–6 hours. In the presence of renal insufficiency, the dose should be changed to 2.25 grams every 8 hours (33).
Comment
Piperacillin-tazobactam is a favorite drug for empiric coverage of suspected Gram-negative infections in the ICU. However, Figures 52.1 and 52.2 show that there are more effective drugs than piperacillin/tazobactam for empiric coverage, and treatment, of Gram-negative infections in the ICU.
VANCOMYCIN & ALTERNATIVES
Vancomycin is the bedrock of antibiotic therapy in the ICU, and has been for several years.
Antibacterial Spectrum
Vancomycin is active against all Gram-positive cocci, including all strains of Staphylococcus aureus (coagulase-positive, coagulase-negative, methicillin-sensitive, methicillin-resistant) as well as aerobic and anaerobic streptococci (including pneumococcus and enterococcus) (34). It is the drug of choice for penicillin-resistant pneumococci, and is one of the most active agents against Clostridium difficile. Enterococci can be resistant to vancomycin. The prevalence of vancomycin-resistant enterococci (VRE) varies from 2% to 60%, depending on the species involved (34).
Clinical Use
Vancomycin is the drug of choice for infections caused by methicillin-resistant Staphylococcus aureus (MRSA) and Staphylococcus epidermidis. However, as much as 2/3 of the vancomycin use in ICUs is not directed at a specific pathogen, but is used for empiric antibiotic coverage in patients with suspected infections (35). The popularity of vancomycin in empiric antibiotic regimens is a reflection of the prominent role played by MRSA and S. epidermidis in ICU-related infections.
Dosing Regimens
Vancomycin dosing is based on body weight and renal function.
Weight-Based Dosing
Standard dosing recommendations for vancomycin (1 gram IV every 12 hrs for normal renal function) frequently results in subtherapeutic vancomycin levels in blood. As a result, weight-based dosing is now recommended for vancomycin. The loading dose for most patients is 15–20 mg/kg, and a larger loading dose of 25–30 mg/kg is recommended for critically ill patients (36). Actual body weight can be used unless the weight is more than 20% above the upper limit of normal for ideal body weight. For overweight patients, an adjusted body weight should be calculated using Equation 52.1 (37).
After the loading dose, subsequent dosing is determined by renal function and the target vancomycin level in blood. An example of a vancomycin dosing nomogram based on body weight, renal function, and a target vancomycin blood level, is shown in Table 52.7. Most hospital pharmacies have nomograms, and will determine the appropriate vancomycin dose for you. After the dosing begins, serum vancomycin levels are used to adjust the dosing regimen.
DRUG LEVELS: Monitoring vancomycin blood levels is recommended when the drug is used to treat serious infections. Steady-state drug levels are usually reached after the fourth intravenous dose (36). Trough blood levels should be >10 mg/L to prevent the development of resistance. For serious infections, trough blood levels of 15–20 mg/L are recommended (36).
Toxicity
Rapid administration of vancomycin can be accompanied by vasodilation, flushing, and hypotension (red man syndrome) as a result of histamine release from mast cells (34). The trigger for this release is unknown, but slowing the infusion rate (to less than 10 mg/min) usually corrects the problem.
Original reports of vancomycin-induced nephrotoxicity were likely due to impurities in the vancomycin preparation, or other nephrotoxic drugs, because recent reports are unable to confirm nephrotoxicity with vancomycin monotherapy (34). There is evidence of an immune-mediated thrombocytopenia in 20% of patients receiving vancomycin (38), and vancomycin-induced neutropenia has been reported in 2–12% of patients who receive the drug for more than 7 days (39).
Table 52.7 Vancomycin Dosing Nomogram
Comment
Vancomycin continues to be a solid performer in the ICU, but alternative drugs are needed for infections with vancomycin-resistant enterococci, and for patients who are unable to receive vancomycin (e.g., because of toxic reactions). There is also a need for a vancomycin replacement, to curb the current use of vancomycin, and slow the rate of microbial resistance.
Alternatives
The alternative antibiotics for vancomycin are included in Table 52.8.
Table 52.8 Alternatives to Vancomycin
Linezolid
Linezolid is a synthetic antibiotic that has the same spectrum of activity as vancomycin (including MRSA), but is also active against vancomycin-resistant enterococci (VRE) (34). The intravenous dose is 600 mg twice daily. Linezolid has much better penetration into lung secretions than vancomycin, but original studies suggesting improved outcomes with linezolid in MRSA pneumonia have not been confirmed in a review of all available studies (40).
Linezolid could be used as a replacement for vancomycin, although resistance is already beginning to appear (34). Toxicity associated with linezolid includes thrombocytopenia (with prolonged use) (34), a partially-reversible optic neuropathy (41), and serotonin syndrome (see Table 42.3).
Daptomycin
Daptomycin is a naturally occurring antibiotic that is active against Gram-positive organisms, including MRSA and VRE (34). The recommended IV dose is 4–6 mg/kg given once daily. Dose reductions are recommended for creatinine clearance <30 mL/min (34).
Daptomycin can be used to treat soft tissue infections or bacteremias involving MRSA and VRE (34). However, it cannot be used to treat pneumonias (42) because it is inactivated by surfactants in the lung. The major toxicity of daptomycin is a skeletal muscle myopathy, and monitoring of serum CPK levels is recommended during therapy with daptomycin (34).
Quinupristin-Dalfopristin
Quinupristin-dalfopristin is a combination of naturally occurring compounds that was the first antibiotic introduced for the treatment of VRE infections. The recommended dose is 7.5 mg/kg IV every 8 hours (34). The principal use of this drug is for infections involving VRE, and use has been curtailed because of troublesome side effects, including painful myalgias and arthralgias (34).
A FINAL WORD
A Simplified Approach
The first rule of antibiotics is try not to use them, and the second rule is try not to use too many of them for too long. If empiric antibiotic coverage is necessary, pending culture results, the combination of vancomycin and meropenem will provide adequate coverage for most infections. If invasive candidiasis is a concern, add fluconazole or caspofungin. You can then tailor antibiotic therapy according to the culture results, or discontinue antibiotics if the cultures are sterile (unless you suspect invasive candidiasis, when antifungal therapy should be continued until you have an answer). Remember that fever and leukocytosis are signs of systemic inflammation, not infection, and that only 25–50% of ICU patients with signs of systemic inflammation will have a documented infection (see page 266).
REFERENCES
Aminoglycosides
1. Craig WA. Optimizing aminoglycoside use. Crit Care Clin 2011; 27:107–111.
2. Babinchak T, Badal R, Hoban D, Hackel M, et al. Trends in susceptibility of selected gram-negative bacilli isolated from intra-abdominal infections in North America: SMART 2005-2010. Diag Micro Infect Dis 2013; 76:379–381.
3. Martinez JA, Cobos-Triqueros N, Soriano A, et al. Influence of empiric therapy with a beta-lactam alone or combined with an aminoglycoside on prognosis of bacteremia due to gram-negatice organisms. Antimicrob Agents Chemother 2010; 54:3590–3596.
4. Wallach J. Interpretation of diagnostic tests. 8th ed. Philadelphia: Lippincott, Williams & Wikins, 2007:1095.
5. Turnidge J. Pharmacodynamics and dosing of aminoglycosides. Infect Dis Clin N Am 2003; 17:503–528.
6. Wilson SE. Aminoglycosides: assessing the potential for nephrotoxicity. Surg Gynecol Obstet 1986; 171(Suppl):24–30.
7. Lippmann M, Yang E, Au E, Lee C. Neuromuscular blocking effects of tobramycin, gentamicin, and cefazolin. Anesth Analg 1982; 61:767–770.
8. Drachman DB. Myasthenia gravis. N Engl J Med 1994; 330:179–1810.
Antifungal Agents
9. Groll AH, Gea-Banacloche JC, Glasmacher A, et al. Clinical pharmacology of antifungal compounds. Infect Dis Clin N Am 2003; 17:159–191.
10. Limper AH, Knox KS, Sarosi GA, et al. An official American Thoracic Society statement: Treatment of fungal infections in adult pulmonary and critical care patients. Am J Respir Crit Care Med 2011; 183:96–128.
11. Bult J, Franklin CM. Using amphotericin B in the critically ill: a new look at an old drug. J Crit Illness 1996; 11:577–585.
12. Carlson MA, Condon RE. Nephrotoxicity of amphotericin B. J Am Coll Surg 1994; 179:361–381.
13. Walsh TJ, Finberg RW, Arndt C, et al. Liposomal amphotericin B for empirical therapy in patients with persistent fever and neutropenia. N Engl J Med 1999; 340:764–771.
14. Wingard JR, Kublis P, Lee L, et al. Clinical significance of nephrotoxicity in patients treated with amphotericin B for suspected or proven aspergillosis. Clin Infect Dis 1999; 29:1402–1407.
15. Wade WL, Chaudhari P, Naroli JL, et al. Nephrotoxicity and other adverse events among inpatients receiving liposomal amphotericin B and amphotericin B lipid complex. Diag Microbiol Infect Dis 2013; 76:361–367.
16. Gearhart MO. Worsening of liver function with fluconazole and a review of azole antifungal hepatotoxicity. Ann Pharmacother 1994; 28:1177–1181.
17. Mora-Duarte J, Betts R, Rotstein C, et al. Comparison of caspofungin and amphotericin B for invasive candidiasis. N Engl J Med 2002; 347:2020–2029.
18. Echinocandins. In: McEvoy GK, ed. AHFS Drug Information, 2012. Bethesda: American Society of Health-System Pharmacists, 2012:528–538.
Carbapenems
19. Baughman RP. The use of carbapenems in the treatment of serious infections. J Intensive Care Med 2009; 24:230–241.
20. Freifield A, Walsh T, Marshall D, et al. Monotherapy for fever and neutropenia in cancer patients: a randomized comparison of ceftazidime versus imi-penem. J Clin Oncol 1995; 13:165–176.
21. Cunha B. Meropenem for clinicians. Antibiotics for Clinicans 2000; 4:59–66.
22. Hellinger WC, Brewer NS. Imipenem. Mayo Clin Proc 1991; 66:1074–1081.
22. Bennett WM, Aronoff GR, Golper TA, et al. eds. Drug prescribing in renal failure. 3rd ed. Philadelphia: American College of Physicians, 1994.
23. Carbapenems. In: McEvoy GK, ed. AHFS Drug Information, 2012. Bethesda: American Society of Health-System Pharmacists, 2012:166–182.
Cephalosporins
24. Asbel LE, Levison ME. Cephalosporins, carbapenems, and monobactams. Infect Dis Clin N Am 2000; 14:1–10.
25. Cephalosporins: General statement. In: McEvoy GK, ed. AHFS Drug Infor-mation, 2012. Bethesda: American Society of Health-System Pharmacists, 2012:68–83.
Fluoroquinolones
26. Rotschafer JC, Ullman MA, Sullivan CJ. Optimal use of fluoroquinolones in the intensive care setting. Crit Care Clin 2011; 27:95–106.
27. Walker RC, Wright AJ. The fluoroquinolones. Mayo Clin Proc 1991; 66:1249–1259.
28. Robson RA. The effects of quinolones on xanthine pharmacokinetics. Am J Med 1992; 92(Suppl 4A):22S–26S.
29. Maddix DS. Do we need an intravenous fluoroquinolone? West J Med 992; 157:55–59.
30. Finch C, Self T. Quinolones: recognizing the potential for neurotoxicity. J Crit Illness 2000; 15:656–657.
31. Frothingham R. Rates of torsade de pointes associated with ciprofloxacin, ofloxacin, levofloxacin, gatifloxacin, and moxifloxacin. Pharmacother 2001; 21:1468–1472.
Penicillins
32. Wright AJ. The penicillins. Mayo Clin Proc 1999; 74:290–307.
33. Piperacillin and Tazobactam. In: McEvoy GK, ed. AHFS drug information, 2012. Bethesda: American Society of Hospital Pharmacists, 2012:340–344.
Vancomycin
34. Nailor MD, Sobel JD. Antibiotics for gram-positive bacterial infections: vancomycin, teicoplanin, quinupristin/dalfopristin, oxazolidinones, daptomycin, dalbavancin, and telavancin. Infect Dis Clin N Am 2009; 23:965–982.
35. Ena J, Dick RW, Jones RN. The epidemiology of intravenous vancomycin usage in a university hospital. JAMA 1993; 269:598–605.
36. Rybak M, Lomaestro B, Rotschafer JC, et al. Therapeutic monitoring of vancomycin in adult patients: A consensus review of the American Society of Health System Pharmacists, the Infectious Disease Society of America, and the Society of Infectious Diseases Pharmacists. Am J Heath-Syst Pharm 2009; 66:82–98.
37. Leong JVB, Boro MS, Winter ME. Determining vancomycin clearance in an overweight and obese population. Am J Heath-Syst Pharm 2011; 68:599–603.
38. Von Drygalski A, Curtis B, Bougie DW, et al. Vancomycin-induced immune thrombocytopenia. N Engl J Med 2007; 356:904–910.
39. Black E, Lau TT, Ensom MHH. Vancomycin-induced neutropenia. Is it dose- or duration-related? Ann Pharmacother 2011; 45:629–638.
Alternatives to Vancomycin
40. Kali AC, Murthy MH, Hermsen ED, et al. Linezolid versus vancomycin or teicoplanin for nosocomial pneumonia: A systematic review and meta-analysis. Crit Care Med 2010; 38:1802–1808.
41. Rucker JC, Hamilton SR, Bardenstein D, et al. Linezolid-associated toxic optic neuropathy. Neurology 2006; 66:595–598.
42. Daptomycin. In: McEvoy GK, ed. AHFS drug information, 2012. Bethesda: American Society of Hospital Pharmacists, 2012:454–457.
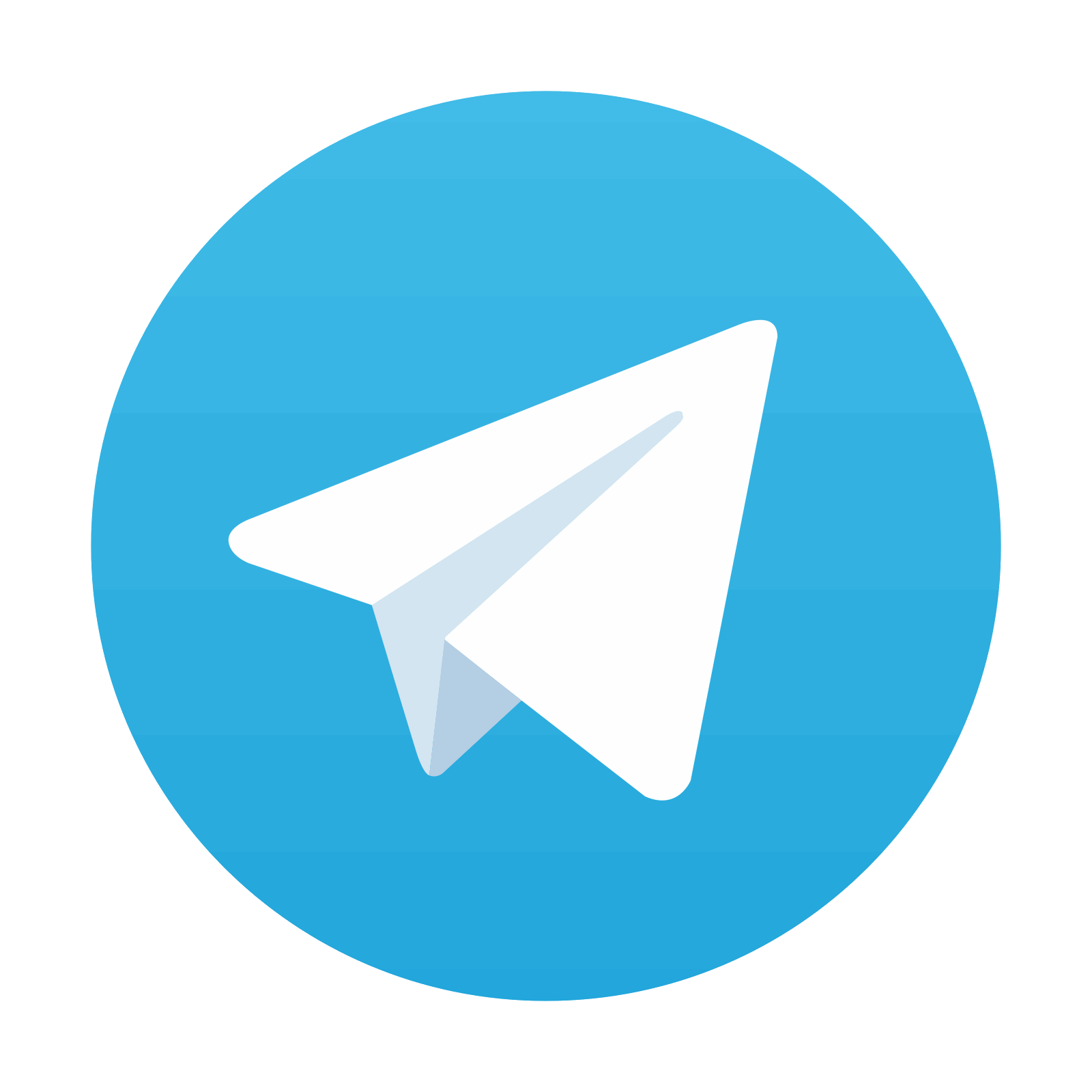
Stay updated, free articles. Join our Telegram channel

Full access? Get Clinical Tree
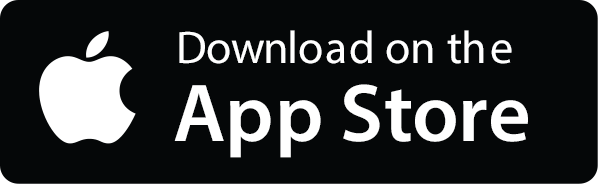
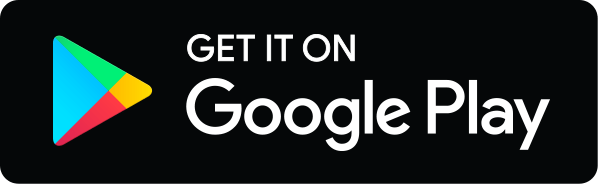