FIGURE 17.1 Rates of survival and satisfactory neurologic recovery in cardiac arrests that occur inside the hospital (graph on the left) and outside the hospital (graph on the right), grouped according to the responsible rhythm disturbance. N=number of cases included in each survey, VF=ventricular fibrillation, VT=ventricular tachycardia, PEA=pulseless electrical activity. Data from References 2 and 3.
Chest Compressions
The most recent recommendations for chest compressions are summarized in Table 17.1 (6). The principal feature is the emphasis on early and uninterrupted chest compressions.
Early Chest Compressions
For the initial resuscitation, first responders should begin with a series of 30 chest compressions, followed by 2 rescue breaths. Chest compressions should be delivered at a rate of at least 100/min. The initial compression:ventilation ratio of 30:2 should be repeated until an advanced airway (e.g., endotracheal tube) is placed. Thereafter, lung inflations are provided at regular intervals (see later), while the chest compressions continue without interruption.
RATIONALE: The emphasis on early chest compressions is based on the observation that delays to initiating CPR have an adverse effect on survival (see later) (6). The importance of chest compressions over ventilation is demonstrated by the practice of hands-only bystander CPR (chest compressions only), which has the same survival benefit as standard CPR (chest compressions plus rescue breaths) in the initial resuscitation period (7). Chest compressions can produce cardiac outputs that are 25% or 30% of normal (6), but this effect diminishes rapidly with delays to initiating CPR.
Table 17.1 Recommendations for Chest Compressions
Avoiding Interruptions
Avoiding unnecessary interruptions in chest compressions is also emphasized. Observational studies have shown that interruptions in chest compressions are common, and the accumulated time of these interruptions can be as much as 50% of the total resuscitation time (8). Prolonging the time without chest compressions is considered to have a negative effect on outcomes (6), although there is evidence that is contrary to this view (9).
Ventilation
Prior to endotracheal intubation, rescue breathing is provided by a face mask that is connected to a self-inflating ventilation bag (e.g., Ambu Respirator) that fills with oxygen. The bag is compressed by hand to deliver the breath, and 2 breaths are provided for every 30 chest compressions, as mentioned earlier. After an endotracheal tube is in place, lung inflations are delivered at 6- to 8-second intervals (8 to 10 breaths/min) using the same type of self-inflating ventilation bag that is used for the bag-mask device. The recommended volume for each lung inflation is 6–7 mg/kg (6), or about 500 mL in a normal sized adult.
Inflation Volumes
The volume of lung inflations is not monitored during “bagged ventilation,” and large inflation volumes are considered to be common during CPR (6), resulting in troublesome hyperinflation of the lungs (10). Adherence to the recommended inflation volumes (6–7 mL/kg) is possible if the volume capacity of the inflation bag is known. For example, if the inflation bag has a volume capacity of 1 liter, compressing the bag until it is about half full will deliver close to 500 mL for lung inflation. (The volume capacity of most adult ventilation bags is 1 to 2 liters.) An alternative method is to use one hand to compress the ventilation bag; this generates a volume of 600 to 800 mL (personal observation), and is unlikely to produce short-term hyperinflation.
Hyperventilation During CPR
Rapid lung inflation rates are common during CPR (10,11), and average rates of 30 inflations/min (3 times the recommended rate) have been reported (11). Rapid breathing is problematic because there is insufficient time for the lungs to empty, and this results in progressive hyperinflation and positive end-expiratory pressure (PEEP). This is called dynamic hyperinflation, and is described in more detail Chapter 27. The increased intrathoracic pressure associated with PEEP has two adverse effects. First, it reduces venous return to the heart, which limits the ability of chest compressions to augment cardiac output. Secondly, it reduces coronary perfusion pressure (10), which is an important determinant of outcome in cardiac arrest. These adverse effects provide a reason to avoid rapid rates of “bagged ventilation.”
High-Quality CPR
The practices listed in Table 17.2 (which have all been described in this section) are considered essential for providing high-quality CPR (6). This table is included to provide you with a checklist for achieving the highest level of performance in CPR.
Table 17.2 The Elements of High-Quality CPR
ADVANCED LIFE SUPPORT
Advanced cardiovascular life support, or ACLS, includes a variety of interventions such as airway intubation, mechanical ventilation, defibrillation, and the administration of life-supporting drugs (12). This section will focus on defibrillation and life-supporting drugs, and will describe these interventions using a rhythm-based approach. This approach divides the management of cardiac arrest into 2 pathways: one for the management of ventricular fibrillation (VF) and pulseless ventricular tachycardia (VT), and the other for the management of pulseless electrical activity (PEA) and asystole.
VF and Pulseless VT
The outcomes in cardiac arrest are most favorable when the initial rhythm is VF or pulseless VT, as demonstrated in Figure 17.1. This is the result of prompt electrical cardioversion, as described next.
Defibrillation
Electrical cardioversion using asynchronous shocks (i.e., not timed to the QRS complex), which is called defibrillation, is the most effective resuscitation measure for cardiac arrest associated with VF and pulseless VT. The survival benefit from defibrillation is time-dependent; i.e., the time elapsed from the cardiac arrest to the first electric shock is the most important factor in determining the likelihood of survival (12–14). This is demonstrated in Figure 17.2 (14). Note that 40% of patients survived when the first shock was delivered 5 minutes after the arrest, while only 10% of patients survived if defibrillation was delayed until 20 minutes after the arrest. These results emphasize the importance of prompt defibrillation when VF or pulseless VT is first apparent in a cardiac arrest victim.
IMPULSE ENERGY: Modern defibrillators deliver current based on stored energy, and the strength of the impulses is expressed in joules (J), which is a unit of thermal energy (see Appendix 1). The appropriate strength for effective cardioversion is determined by the waveform of the energy impulse. Biphasic waveforms (used by newer defibrillators) are effective at lower energy levels than monophasic waveforms (used by older defibrillators). For termination of VF and pulseless VT, the effective impulse strengths are 120 to 200 J for biphasic shocks, and 360 J for monophasic shocks (12,13). Manual defibrillators used in hospitals require the operator to select the desired energy level of the impulse, while automated external defibrillators (AEDs) use a preselected energy level.
FIGURE 17.2 Survival in out-of-hospital cardiac arrests with “shockable” arrhythmias (VF/pulseless VT) in relation to the time elapsed from the onset of the cardiac arrest to the initial defibrillation attempt. N=number of cases studied. Data from Reference 14.
Management Protocol
The flow diagram in Figure 17.3 is the ACLS algorithm for cardiac arrest in adults. The left half of the diagram is the management of cardiac arrest associated with VF and pulseless VT. The major features of the management are summarized below.
1. The management includes a series of 3 defibrillation attempts, if needed, and the impulse strength should be the same for each defibrillation. The recommended impulse strength is 120–200 J for bi-phasic waveforms, and 360 J for monophasic waveforms (12,13).
2. For each defibrillation attempt, one member of the resuscitation team charges the defibrillator and selects the impulse strength, while another member of the team provides chest compressions. The chest compressions are withheld when the defibrillation shock is delivered, and are resumed immediately thereafter. At least 2 minutes of uninterrupted chest compressions are recommended after defibrillation before the compressions are interrupted again to check the post-shock rhythm. If the rhythm is unchanged, the process is repeated twice, if necessary.
3. If a second defibrillation attempt is required, bolus injections of epinephrine are started using a dose of 1 mg IV (or intraosseous – IO) every 3–5 min, and these are continued for the duration of the resuscitation effort. A single dose of vasopressin (40 Units IV) can replace the first or second dose of epinephrine.
4. If a third defibrillation attempt is required, amiodarone is administered in an IV (or IO) dose of 300 mg, which can be followed by a second dose of 150 mg, if needed.
Failure to terminate VF or VT with the first or second defibrillation attempt carries a poor prognosis because the longer the arrhythmia persists, the less the likelihood of a successful outcome.
Asystole/PEA
The management of cardiac arrest associated with pulseless electrical activity (PEA) or ventricular asystole is notoriously unsuccessful, as indicated in Figure 17.1. The principal elements of the management are shown in the right half of Figure 17.3. The major intervention is vasopressor therapy with epinephrine using the same dosing regimen used for VF and pulseless VT. Defibrillation is not attempted unless the cardiac rhythm changes to VF or VT.
FIGURE 17.3 The ACLS cardiac arrest algorithm. Adapted from Reference 12.
Reversible Causes of PEA
PEA offers some hope because there are reversible causes, and the prominent ones are easily remembered with the letter T: i.e., Tension pneumothorax, pericardial Tamponade, pulmonary Thromboembolism, and Throm-botic occlusion of the coronary arteries. Unfortunately, there is little time for a diagnostic workup during a cardiac arrest, but pericardial tamponade and tension pneumothorax can be rapidly identified at the bedside using ultrasound imaging, which is readily available in many ICUs.
Resuscitation Drugs
The use of drugs is considered a second-line treatment in cardiac arrest because there is no documented survival benefit (12). This may not be surprising, considering that the drugs are administered at a time of cardiovascular collapse, when drug delivery to target sites is sluggish and may not occur at all. The cardiac arrest drugs are either vasopressors or antiarrhythmic agents, and the recommended dosing regimen for each drug is shown in Table 17.3.
Vasopressors
EPINEPHRINE produces systemic vasoconstriction, which is accompanied by an increase in coronary perfusion pressure (the difference between aortic and right atrial relaxation pressures during the time between chest compressions). This is demonstrated in Figure 17.4 (15). In this case, there is a 30% increase in coronary perfusion pressure following IV epinephrine, and the effect lasts at least 3 minutes (the recommended time interval between epinephrine doses). The disadvantage with epinephrine is the β-Receptor-mediated cardiac stimulation, which can erase the benefit of increased coronary perfusion, and has also been implicated in postresuscitation heart failure (15).
Epinephrine use is associated with an increased rate of return to spontaneous circulation (ROSC), but the mortality rate is unchanged (12,16).
VASOPRESSIN is a non-adrenergic vasoconstrictor that is recommended only as a single dose (40 Units IV bolus) that can be used to replace the first or second dose of epinephrine (12). The advantage of vasopressin is the absence of cardiac stimulation, but vasopressin also causes coronary vasoconstriction, which is counterproductive. Clinical trials have shown no apparent advantage of vasopressin over epinephrine (17).
Antiarrhythmic Agents
AMIODARONE is the preferred antiarrhythmic agent for VF/VT-associated cardiac arrest that is refractory to defibrillation and vasopressor therapy (12). This preference is based on clinical studies that show superior results with amiodarone compared to placebo (18) or lidocaine (19). However, the superior results with amiodarone are limited to increased survival to hospital admission, but not to hospital discharge.
LIDOCAINE is the original antiarrhythmic agent used for shock-resistant VF and pulseless VT, but it is less effective than amiodarone, and should be used only when amiodarone is not available.
MAGNESIUM is used for polymorphic VT, but only when the arrhythmia is associated with QT prolongation (torsade de pointes). The recognition and treatment of polymorphic VT are described in Chapter 15.
The Endotracheal Route
In the rare instance when intravenous or intraosseous access is not available, some ACLS drugs (i.e., epinephrine, vasopressin, and lidocaine) can be administered by injection into the upper airway through an endotracheal tube. The drug dose for endotracheal injection should be 2–2.5 times the intravenous dose (e.g., 2–2.5 mg for epinephrine) (12).
Table 17.3 Advanced Cardiac Life Support Drugs
RESUSCITATION MONITORING
Monitoring for the return of spontaneous circulation (ROSC) is typically limited to palpation for carotid artery pulsations, but this is discouraged as a sole practice (12) because the search for pulses often requires interruption of chest compressions for extended periods of time, and there are often uncertainties about the presence or absence of pulses (20a). The end-tidal PCO2 and central venous O2 saturation provide a more reliable evaluation of the circulation, and can be used to predict the likelihood of ROSC.
FIGURE 17.4 The effect of intravenous epinephrine on coronary perfusion pressure (CPP) during resuscitation of cardiac arrest with VF/pulseless VT. Data from Reference 15.
End-Tidal PCO2
The end-tidal PCO2 measurement is described in detail in Chapter 21. The PCO2 in exhaled gas at the end of expiration (end-tidal PCO2) is a measure of the balance between ventilation and perfusion in the lungs (V/Q balance). The end-tidal PCO2 varies directly (in the same direction) with changes in cardiac output relative to ventilation, and when alveolar ventilation is constant, changes in end-tidal PCO2 reflect proportional changes in cardiac output (e.g., a 30% decrease in end-tidal PCO2 indicates a 30% decrease in the cardiac output). The end-tidal PCO2 is normally equivalent to the arterial PCO2 (i.e., about 40 mm Hg), but it can be lower than the arterial PCO2 in pulmonary conditions associated with increased physiologic dead space (i.e., V/Q ratio >1).
Predictive Value
End-tidal CO2 monitoring provides a measure of exhaled PCO2 for each breath, and serial measurements during CPR can be used to identify when ROSC occurs, or is unlikely to occur. The graph in Figure 17.5 shows the serial changes in end-tidal PCO2 during 20 minutes of CPR in patients who achieved ROSC and in patients who did not achieve ROSC (20b). Note that patients who achieved ROSC showed a progressive increase in end-tidal PCO2 during the resuscitation period, whereas patients who did not achieve ROSC showed a progressive decline in end-tidal PCO2. The end-tidal PCO2 that separated responders from nonresponders in this study was 15 mm Hg after 20 minutes of CPR. Other studies have shown a discriminant value of 10 mm Hg separating survivors and nonsurvivors (21,22).
The available studies indicate that a successful outcome is unlikely if the end-tidal PCO2 is not higher than 10–15 mm Hg after 20 minutes of CPR. When the end-tidal PCO2 remains above this level, continued resuscitation for as long as 11/2 hours has been associated with a favorable outcome (23).
Central Venous O2 Saturation
As described in Chapter 10, the O2 saturation of hemoglobin in central venous (superior vena cava) blood (ScvO2) is a measure of the balance between systemic O2 delivery (DO2) and systemic O2 uptake (VO2). When VO2 is constant, a decrease in DO2 (e.g., from a low cardiac output) is accompanied by a decrease in ScvO2. The normal ScvO2 is about 70–80% (see Chapter 10), and there is evidence that failure to achieve an ScvO2 ≥30% during CPR is associated with failure to achieve ROSC (24). The value of ScvO2 during CPR is limited by the need for a central venous catheter.
FIGURE 17.5 Serial changes in end-tidal PCO2 in relation to return of spontaneous circulation (ROSC) during the resuscitation of cardiac arrest with VF/pulseless VT. Data points represent mean values for the patients in each group. From Reference 20b.
POST-RESUSCITATION PERIOD
The immediate goal of CPR is a return of spontaneous circulation (ROSC), but this does not ensure a satisfactory outcome. In fact, surveys of cardiac arrest victims who survive the initial resuscitation indicate that about 70% do not survive to leave the hospital (25). This section describes the common problems encountered in the days following ROSC, and a few treatment modalities that can alleviate some of these problems.
Post-Cardiac Arrest Syndrome
The release of blood from areas of ischemia triggers an inflammatory response that can damage vital organs. This reperfusion injury is responsible for the post-cardiac arrest syndrome, which is characterized by dysfunction in one or more major organs (most often involving the brain and heart) in the early period following successful resuscitation of cardiac arrest (25,26). The principal features of this syndrome are summarized below:
1. Brain injury is the most common manifestation of the post-cardiac arrest syndrome, and is responsible for 23% to 68% of deaths following cardiac arrest (25). Clinical manifestations include failure to awaken, myoclonus, and generalized seizures. The high prevalence of brain injury after cardiac arrest is attributed to a limited tolerance to ischemia, and a predisposition to oxidative reperfusion injury.
2. Post-arrest cardiac dysfunction is a combination of systolic and diastolic dysfunction that can progress to cardiogenic shock within hours after ROSC (25). The underlying problem is a type of reperfusion injury known as myocardial “stunning,” which usually resolves within 72 hours (25).
3. Systemic inflammatory response syndrome (see Table 14.2) is almost universal after cardiac arrest, and can result in widespread inflammatory injury with multiorgan failure and circulatory shock. This condition can be described as a “whole-body reperfusion injury,” and is usually apparent within 24 hours after cardiac arrest. (See Chapter 14 for a description of inflammatory shock.)
The nexus of the post-cardiac arrest syndrome is inflammatory injury mediated by toxic oxygen metabolites (see Figure 14.1) (27). As with other life-threatening conditions involving oxidant injury, little attention has been given to supporting antioxidant defenses as a therapeutic modality. Of possible relevance in this regard, the consumption of endogenous antioxidants is reduced by hypothermia (28), which is also the most effective treatment for post-arrest brain injury (see next).
Targeted Temperature Management
Induced hypothermia, previously known as human refrigeration (29), was introduced for the management of post-cardiac arrest victims in the mid-twentieth century (30), but was abandoned because of uncertain benefit and the risks of severe hypothermia (<30°C) used at the time. About 40 years later (in 2002), two studies were published showing that comatose survivors of cardiac arrest who were treated with 12 to 24 hours of mild hypothermia (32–34°C) had more favorable neurologic outcomes and fewer deaths (31,32). The results of one of these studies (the larger one) is shown in Figure 17.6 (31). According to these results, mild hypothermia prevented one unfavorable neurologic outcome for every 6 patients treated, and prevented one death for every 7 patients treated. Since these results were published, mild induced hypothermia, now called targeted temperature management or TTM (33), has been adopted with enthusiasm for patients who do not awaken after ROSC. The general features of TTM are presented in Table 17.4 (26,27,30–33).
Who Benefits?
The original reports of benefit with mild hypothermia included cases of out-of-hospital cardiac arrest associated with VF/pulseless VT (31,32), and this condition was initially considered the only indication for mild hypothermia. However, because of the great promise with TTM, it is now a consideration for any patient who does not awaken following ROSC (26), regardless of the associated rhythm or location of the cardiac arrest. The only absolute contraindications to TTM are pre-existing hypothermia (<34°C), major bleeding, or cryoglobulinemia. Hemodynamic instability and cardiogenic shock are not considered absolute contraindications to TTM (26).
FIGURE 17.6 Clinical outcomes with mild hypothermia (32–34°C) and conventional management (normothermia) in comatose survivors of out-of-hospital cardiac arrest with VF/pulseless VT. Data from Reference 31.
The Method
Surface cooling has been the most popular method of TTM, but endovascular cooling is gaining favor because it creates less risk of shivering, and avoids the problem of erratic surface cooling caused by cold-induced vasoconstriction in the skin. The endovascular cooling method requires insertion of a specialized central venous catheter, but this is easily accomplished during the induction phase of cooling (see next). Both types of cooling are optimal when automated, and a number of automated systems are commercially available.
Patients who receive TTM are intubated and mechanically ventilated, and have thermistor-equipped bladder catheters to monitor body temperature. Although the patients are comatose, infusions of sedative agents (e.g., midazolam, propofol, or fentanyl) are often used to alleviate anticipated shivering. The cooling process in TTM is divided into 3 phases: induction, maintenance, and rewarming.
Table 17.4 Targeted Temperature Management
INDUCTION: Rapid cooling is advised with ice-cold (4°C) saline or lactated Ringers fluid infused in 500 mL aliquots every 10 minutes until the body temperature falls to 34°C or the infusion volume reaches 30 mL/kg. Shivering is common during the induction phase, and is counterproductive because it increases the body temperature. Shivering can be controlled with propofol (0.1–0.2 mg/kg/min IV), midazolam (0.02–0.1 mg/kg/hr IV), or fentanyl (25–100 ∝g/hr IV), while magnesium (5 grams IV over 5 hours) can also be effective (25). Refractory shivering is managed with neuromuscular blockade (e.g., cisatracurium, 0.15–0.2 mg/kg IV bolus, then 1–2 ∝g/kg/min, if needed).
MAINTENANCE: Following induction, automated surface cooling or endo-vascular cooling systems maintain the body temperature at 32–34°C for the next 24 hours. Bradycardia is common during hypothermia, but usually requires no intervention. Hypotension can occur as a result of cold-induced diuresis and cardiac depression, and is managed with volume infusions initially, followed by a vasopressor (e.g., norepinephrine) if needed. Hypokalemia is common (from potassium moving into cells), and should be treated cautiously to prevent hyperkalemia during rewarming. If available, continuous EEG recording is advised during TTM because nonconvulsive status epilepticus has been reported an about 10% of patients (34).
REWARMING: Slow rewarming (0.25–0.5°C/hr) is recommended, and is usually managed by the automated cooling system. Watch for hyperkalemia during rewarming (when potassium moves back out of cells), especially when aggressive potassium replacement was used during hypothermia.
After rewarming, it is important to discontinue sedation as soon as possible, because residual sedation can prolong the time to awaken. The evaluation of patients who fail to awaken soon after rewarming is described later.
Fever
As expected from the beneficial effects of hypothermia, fever following a cardiac arrest is associated with unfavorable neurologic outcomes (35). Therefore, prompt antipyretic therapy is advised for patients with fever who do not receive TTM, or for rebound fever that can follow TTM. Acetaminophen is the standard antipyretic agent, and is given in doses of 650 mg or 1000 mg IV or via feeding tube every 6 hours, with a maximum daily dose of 4 grams. Patients with hepatic insufficiency should not receive acetaminophen.
Glycemic Control
Hyperglycemia following cardiac arrest is associated with a poor neurologic outcome (36), although there is no evidence that glycemic control after cardiac arrest improves neurologic outcome. Strict glycemic control in ICU patients is associated with frequent episodes of hypoglycemia (37), so a higher-than-normal range of 144–180 mg/dL for blood glucose is considered a reasonable target (26). As an adjunct to this practice, it is wise to avoid dextrose-containing intravenous solutions whenever possible.
Predicting Neurologic Recovery
In patients who do not regain consciousness after CPR or induced hypo-thermia, the single most important determination is the likelihood of neurologic recovery. The traditional signs that predict a poor neurologic outcome are based on observations performed before hypothermia was adopted as a treatment modality (38,39), and these signs may not apply to patients who are treated with hypothermia (40–42). The following sections describe the signs that predict a poor neurologic outcome after CPR, and whether or not these signs also apply to patients treated with hypothermia. This information is summarized in Table 17.5
Table 17.5 Predictive Signs of a Poor Outcome in Patients Who Do Not Awaken After CPR or Induced Hypothermia
Time to Awaken
Following CPR, most (80–95%) of the patients who regain consciousness are awake after 72 hours (38,40), but it can take 7 days or even longer for all the patients to awaken (38). There is a perception that hypothermia prolongs the time to awaken, but this remains unproven. In one retrospective study (40), the median time to awaken was the same (2 days) after CPR and after hypothermia, and a larger percentage of the patients who received hypothermia were awake at 72 hours (91%) compared to patients who did not receive hypothermia (79%). In general, the time to awaken can be a poor predictor of neurologic outcome in the first week following CPR, with or without hypothermia. Residual sedation may play a role in prolonging the time to awaken, particularly in patients who are treated with hypothermia (40).
Brainstem Reflexes
Absence of brainstem reflexes is highly predictive of a poor outcome in patients who remain comatose after CPR and hypothermia. When papillary light reflexes or corneal reflexes are absent 3 days after CPR or hypothermia, none of the patients have a satisfactory neurologic recovery (39,41,42).
Best Motor Response
Patients who remain comatose for 72 hours after CPR have no chance of a satisfactory neurologic recovery if they show no motor response to pain, or an abnormal extensor response (i.e., decerebrate posturing) (38,39). However, when hypothermia is used, as many as 25% of patients who show no motor response at 72 hours will have a satisfactory recovery (43). Therefore, poor motor responses at 72 hours do not predict a poor outcome in patients treated with hypothermia.
Status Epilepticus
Myoclonic status epilepticus (repetitive, irregular movements of the face, trunk, and extremities) often appears in the first 24 hours after a cardiac arrest (41), and is a poor prognostic sign for recovery in all patients, including those who receive hypothermia (39,41,42). Generalized status epilepticus (repetitive, tonic-clonic movements of the face, trunk, and extremities), including nonconvulsive status epilepticus (no seizure-like movements), is a poor prognostic sign without hypothermia, but does not always indicate a poor outcome following hypothermia (41).
Sedation and Prognosis
The prognostic evaluation following hypothermia requires further study; however, one issue that is emerging is the possible interference of unsuspected sedation in the neurologic evaluation following hypothermia. The administration of opiates and other sedating drugs is commonplace during hypothermia and rewarming (which can last for 30 hours or longer), and slowed drug metabolism from hypothermia could result in prolonged sedation after the procedure. This could prolong the time to awaken, and create misleading signs of a poor neurologic recovery. Attention to limiting the use of sedating drugs, if possible, during hypo-thermia and rewarming will limit the risk of errors in interpreting persistent unresponsiveness, and can avoid the embarrassing situation where a patient wakes up after you have assured the family that there is little or no chance of awakening.
A FINAL WORD
Perception
Cardiopulmonary resuscitation has always enjoyed a popularity far greater than deserved. This is evident in surveys of the general public, where 95% of respondents have unrealistic expectations about CPR (44), including the perception that more than half of cardiac arrest victims not only survive, but return to daily life with no residual effects (45). Television shows mirror this perception, where CPR is portrayed as a success in 67 to 75% of cases (46).
Reality
The reality of CPR is far removed from perception; i.e., on average, less than 10% of patients who receive CPR survive to leave the hospital (47), and when the responsible rhythm is asystole or PEA, as few as 2% of patients have a satisfactory recovery (see Table 17.1). Thus, the reality of CPR is that it doesn’t produce satisfactory results in most instances, even when trained personnel are immediately available.
Why is this discrepancy between perception and reality so important? Because perception is dictating CPR practices; i.e., patients decide whether CPR is performed, not clinical practitioners.
REFERENCES
1. Kouwenhoven WB, Ing, Jude JR, Knickerbocker GG. Closed-chest cardiac massage. JAMA 1960; 173:1064–1067.
Clinical Outcomes
2. Nadkarni VM, Laarkin GL, Peberdy MA, et al, for the National Registry of Cardiopulmonary Resuscitation Investigators. First documented rhythm and clinical outcome from in-hospital cardiac arrest among children and adults. JAMA 2006; 295:50–57.
3. Yasanuga H, Horiguchi H, Tanabe S, et al. Collaborative effects of bystander-initiated cardiopulmonary resuscitation and prehospital advanced cardiac life support by physicians on survival of out-of-hospital cardiac arrest: a nationwide population-based observational study. Crit Care 2010; 14:R199–R210.
American Heart Association Guidelines
4. 2010 American Heart Association Guidelines for Cardiopulmonary Resusci-tation and Emergency Cardiovascular Care Science with Treatment Recom-mendations. Circulation, volume 122, issue 16, supplement 2, October 16, 2010. (Available online @ http://circ.ahajournals.org/content/122/16_suppl_2.toc)
5. Advanced Cardiovascular Life Support Provider Manual. Dallas, TX: Amer-ican Heart Association, 2011.
Basic Life Support
6. Berg RA, Hemphill R, Abella BS, et al. Part 5: Adult basic life support: 2010 American Heart Association Guidelines for Cardiopulmonary Resuscitation and Emergency Cardiovascular Care. Circulation 2010; 122 (suppl 3):S685–S705.
7. Hupfl M, Selig H, Nagele P. Chest compression-only CPR: a meta-analysis. Lancet 2010; 376:1552–1557.
8. Wit L, Kramer-Johansen J, Mykelbust H, et al. Quality of cardiopulmonary resuscitation during out-of-hospital cardiac arrest. JAMA 2005; 293:299–304.
9. Jost D, Degrance H, Verret C, et al. DEFI 2005: a randomized controlled trial of the effect of automated external defibrillator cardiopulmonary resuscitation protocol on outcome from out-of-hospital cardiac arrest. Circulation 2010; 121:1614–1622.
10. Aufderheide TP, Lurie KG. Death by hyperventilation: A common and life-threatening problem during cardiopulmonary resuscitation. Crit Care Med 2004; 32(Suppl):S345–S351.
11. Abella BS, Alvarado JP, Mykelbust H, et al. Quality of cardiopulmonary resuscitation during in-hospital cardiac arrest. JAMA 2005; 293:305–310.
Advanced Life Support
12. Neumar RW, Otto CW, Link MS, et al. Part 8: adult advanced cardiovascular life support: 2010 American Heart Association Guidelines for Cardiopul-monary Resuscitation and Emergency Cardiovascular Care. Circulation 2010; 122 (suppl 3):S729–S767.
13. Link MS, Atkins DL, Passman RS, et al. Part 6: electrical therapies: automated external defibrillators, defibrillation, cardioversion, and pacing: 2010 American Heart Association Guidelines for Cardiopulmonary Resuscitation and Emerg-ency Cardiovascular Care. Circulation 2010; 122 (suppl 3):S706–S719.
14. Larsen MP, Eisenberg M, Cummins RO, Hallstrom AP. Predicting survival from out of hospital cardiac arrest: a graphic model. Ann Emerg Med 1993; 22:1652–1658.
15. Sun S, Tang W, Song F, et al. The effects of epinephrine on outcomes of mormothermic and therapeutic hypothermic cardiopulmonary resuscitation. Crit Care Med 2010; 38:2175–2180.
16. Herlitz J, Ekstrom L, Wennerblom B, et al. Adrenaline in out-of-hospital ventricular fibrillation. Does it make any difference? Resuscitation 1995; 29:195–201.
17. Aung K, Htay T. Vasopressin for cardiac arrest: a systematic review and meta-analysis. Arch Intern Med 2005; 165:17–24.
18. Kudenchuk PJ, Cobb LA, Copass MK, et al. Amiodarone for out-of-hospital cardiac arrest due to ventricular fibrillation. New Engl J Med 1999; 341:871–878.
19. Dorian P, Cass D, Schwartz B, et al. Amiodarone as compared to lidocaine for shock-resistant ventricular fibrillation. New Engl J Med 2002; 346:884–890.
Resuscitation Monitoring
20a. Ochoa FJ, Ramalle-Gomara E, Carpintero JM, et al. Competence of health professionals to check the carotid pulse. Resuscitation 1998; 37:173–175.
20b. Kolar M, Krizmaric M, Klemen P, Grmec S. Partial pressure of end-tidal carbon dioxide predicts successful cardiopulmonary resuscitation – a prospective observational study. Crit Care 2008; 12:R115. Full text available on PubMed; accessed on 10/15/2012
21. Sanders AB, Kern KB, Otto CW, et al. End-tidal carbon dioxide monitoring during cardiopulmonary resuscitation. JAMA 1989; 262:1347–1351.
22. Wayne MA, Levine RL, Miller CC. Use of end-tidal carbon dioxide to predict outcome in prehospital cardiac arrest. Ann Emerg Med 1995; 25:762–767.
23. White RD, Goodman BW, Svoboda MA. Neurologic recovery following prolonged out-of-hospital cardiac arrest with resuscitation guided by continuous capnography. Mayo Clin Proc 2011; 86:544–548.
24. Rivers EP, Martin GB, Smithline H, et al. The clinical implications of continuous central venous oxygen saturation during human CPR. Ann Emerg Med 1992; 21:1094–1101.
Post-Resuscitation Period
25. Nolan JP, Neumar RW, Adrie C, et al. Post-cardiac arrest syndrome: epidemiology, pathophysiology, and prognostication. A scientific statement from the International Liaison Committee on Resuscitation; the American Heart Association Emergency Cardiovascular Care Committee; the Council on Cardiovascular Surgery and Anesthesia; the Council on Cardiopulmonary, Perioperative, and Critical Care; the Council on Clinical Cardiology; the Council on Stroke. Resuscitation 2008; 79:350–379.
26. Peberdy MA, Callaway CW, Neumar RW, et al. Part 9: post-cardiac arrest care. 2010 American Heart Association Guidelines for Cardiopulmonary Resuscitation and Emergency Cardiovascular Care. Circulation 2010; 122 (suppl 3):S768–S786.
27. Huet O, Dupic L, Batteux F, et al. Post-resuscitation syndrome: potential role of hydroxyl radical-induced endothelial cell damage. Crit Care Med 2011; 39:1712–1720.
28. Karibe H, Chen SF, Zarow GJ, et al. Intraischemic hypotherma suppresses consumption of endogenous antioxidants after temporary focal ischemia in rats. Brain Res 1994; 649:12–18.
29. Fay T. Observations on prlonged human refrigeration. NY State J Med 1940; 40:1351–1354.
30. Williams GR, Spencer FC. The clinical use of hypothermia after cardiac arrest. Am Surg 1959; 148:462–468.
31. The Hypothermia After Cardiac Arrest Study group. Mild therapeutic hypo-thermia to improve the neurologic outcome after cardiac arrest. N Engl J Med 2002; 346: 549–556.
32. Bernard SA, Gray TW, Buist MD, et al. Treatment of comatose survivors of out-of-hospital cardiac arrest with induced hypothermia. N Engl J Med 2002; 346:557–563.
33. Holzer M. Targeted temperature management for comatose survivors of cardiac arrest. N Engl J Med 2010; 363:1256–1264.
34. Rittenberger JC, Popescu A, Brenner RP, et al. Frequency and timing of nonconvulsive status epilepticus in comatose, post-cardiac arrest subjects treated with hypothermia. Neurocrit Care 2012; 16:114–122.
35. Zeiner A, Holzer M, Sterz F, et al. Hyperthermia after cardiac arrest is associated with an unfavorable neurologic outcome. Arch Intern Med 2001; 161:2007–2012.
36. Calle PA, Buylaert WA, Vanhaute OA. Glycemia in the post-resuscitation period. The Cerebral Resuscitation Study group. Resuscitation 1989; 17 (suppl):S181–S188.
37. Marik PE, Preiser J-C. Towards understanding tight glycemic control in the ICU. A systematic review and meta-analysis. Chest 2010; 137:544–551.
38. Levy DE, Caronna JJ, Singer BH, et al. Predicting outcome from hypoxic-ischemic coma. JAMA 1985; 253:1420–1426.
39. Wijdicks EFM, Hijdra A, Young GB, et al. Practice parameter: Prediction of outcome incomatose survivors after cardiopulmonary resuscitation (an evidence-based review). Report of the Quality Standards Subcommittee of the American Academy of Neurology. Neurology 2006; 67:203–210.
40. Fugate JE, Wijdicks EFM, White RD, Rabinstein AA. Does therapeutic hypothermia affect time to awakening in cardiac arrest survivors? Neuro-logy 2011; 77:1346–1350.
41. De Georgia M, Raad B. Prognosis of coma after cardiac arrest in the era of hypothermia. Continuum Lifelong Learning Neurol 2012; 18:515–531.
42. Fugate JE, Wijdicks EFM, Mandrekar J, et al. Predictors of neurologic outcome in hypothermia after cardiac arrest. Ann Neurol 2010; 68:907–914.
43. Rosetti AO, Oddo M, Logroscino G, et al. Prognostication after cardiac arrest and hypothermia. A prospective study. Ann Neurol 2010; 67:301–307.
A Final Word
44. Jones GK, Brewer KL, Garrison HG. Public expectations of survival following cardiopulmonary resuscitation. Acad Emerg Med 2000; 7:48–53.
45. Marco CA, Larkin GL. Cardiopulmonary resuscitation: knowledge and opinions among the U.S. general public. State of the science-fiction. Resuscitation 2008; 79:490–498.
46. Diem SJ, Lantos JD, Tulsky JA. Cardiopulmonary resuscitation on television. Miracles and misinformation. N Engl J Med 1996; 334:1578–1582.
47. Bohm K, Rosenqvist M, Herlitz J, et al. Survival is similar after standard treatment and chest compressions only in out-of-hospital bystander cardiopulmonary resuscitation. Circulation 2007; 116:2908–2912.
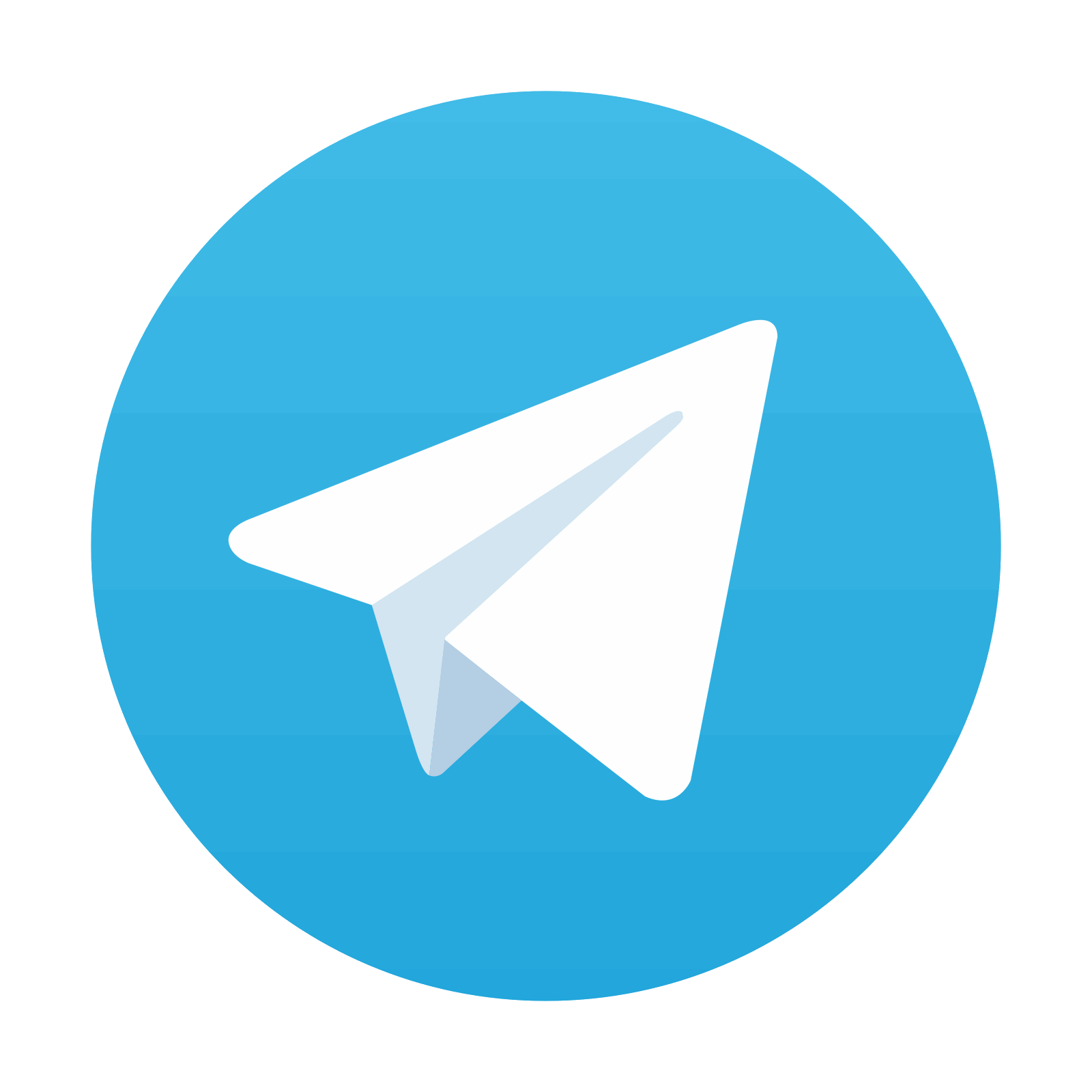
Stay updated, free articles. Join our Telegram channel

Full access? Get Clinical Tree
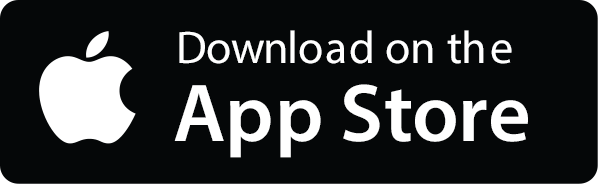
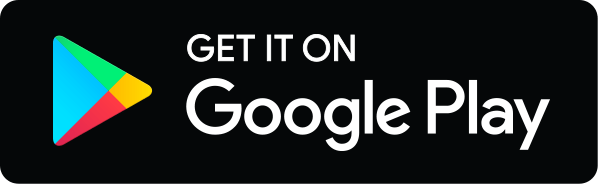