Transtracheal Gas Insufflation, Transtracheal Oxygen Therapy, Emergency Transtracheal Ventilation: Introduction
Clinical evidence highlights the importance of limiting airway pressure during mechanical ventilation. In addition, experimental results suggest the importance of avoiding lung overdistension and cyclic end-expiratory airspace collapse and reexpansion, indicating that both phenomena promote mechanical damage and release of inflammatory mediators.1–3 Unfortunately, interventions that can attenuate the structural insult caused by mechanical ventilation, such as the use of low tidal volume, high positive end-expiratory pressure, and reduced respiratory rate, can limit total minute ventilation.4,5 In this context, transtracheal oxygen therapy and tracheal gas insufflation (TGI) could have a role as adjuncts to mechanical ventilation.6–10
Basic Principles
TGI attempts to minimize dead space by delivering fresh gas through an intratracheal catheter to flush the anatomic dead space free of CO2. During TGI, low-to-moderate flows of fresh gas introduced near the carina, either continuously or in phases, dilute the CO2 in the anatomic dead space proximal to the catheter tip. Because CO2 is washed out during expiration, less CO2 is recycled back into the alveoli during the subsequent inspiration. Any catheter flow during inspiration contributes to the inspired tidal volume (VT) but bypasses the anatomic dead space proximal (mouthward) to the catheter tip. At higher catheter flow rates, turbulence generated at the tip of the catheter by the jet stream can enhance gas mixing in regions distal to the catheter tip, thereby contributing to CO2 removal.11–14 The fresh gas stream exiting the catheter tip rapidly establishes an expiratory front beyond the catheter tip between CO2-rich alveolar gas and CO2-free fresh catheter gas.13 This front is practically abolished by inverting the catheter tip and directing the catheter jet mouthward, thus eliminating the distal effect of TGI.12 These observations indicate that the primary mechanism of CO2 elimination during TGI is expiratory washout, and the forward-directed TGI penetrates a substantial distance into the central airways, extending the compartment susceptible to CO2 washout with a smaller contribution of turbulence beyond the straight catheter tip. Consequently, partial pressure of arterial carbon dioxide (PaCO2) during TGI falls as a nonlinear function of catheter flow rate. Initially, modest flow rates achieve large decrements in PaCO2, but once the anatomic dead space is flushed free of CO2, the effect on PaCO2 diminishes as catheter flow rate increases.6,11
During TGI, fresh gas can be delivered continuously, or delivery can be timed to occur in phases during a specific portion of the respiratory cycle by gating a solenoid valve that either directs the flow to the catheter or diverts it to the atmosphere.15,16 During continuous TGI, closure of the expiratory valve during inspiration causes catheter flow to deliver variable portions of the inspired VT.17,18 Phasic inspiratory TGI can be used as the only source of fresh gas, thereby bypassing the anatomic dead space proximal to the catheter tip.16 It can also be combined with a conventional ventilator to augment alveolar ventilation. During continuous or phasic inspiratory TGI, the catheter-delivered portion of the inspired VT is a function of catheter flow rate and inspiratory time. During phasic expiratory TGI, catheter flow is timed to occur during all or part of expiration and does not contribute appreciably to the inspired VT.
The effect of insufflating fresh gas during specific phases of the respiratory cycle has been examined under different catheter-flow conditions. Catheter flow only during inspiration (inspiratory bypass) effectively avoids the “anatomic” dead space proximal to the catheter tip (extending from the ventilator’s Y piece). Insufflation during late expiration (expiratory washout) washes the proximal dead space free of CO2. Limiting TGI to the final 60% of expiration effectively reduces PaCO2 (not different from panexpiratory TGI) while limiting exposure of the trachea to TGI gas and reducing the potential for TGI-induced hyperinflation (Fig. 22-1).16,19,20 Although experimental studies suggest that restricting the flow of TGI gas to some portion of the expiratory phase preserves effectiveness, a rigorous engineering analysis showed that applying TGI flow solely within the final 50% of the expiratory phase yields a near maximal effect of expiratory TGI (Fig. 22-2), and this approach could simplify implementation and decrease adverse consequences.21
Figure 22-1
Simultaneous tracings during expiratory washout (EWO) at 10 L/min catheter flow. (Above) Plethysmographic lung volume relative to the end-expiratory lung volume measured without catheter flow. (Center) Flow tracing measured in the inspiratory and expiratory limbs of the external circuit. (Below) Proximal airway pressure. Note that lung volume and proximal airway pressure tracings show preinspiratory step changes. These deflections indicate that gas flows both antegrade (volume tracing) and retrograde (flow tracing) from the catheter tip during this period. (Used, with permission, Burke WC, Nahum A, Ravenscraft SA, et al. Modes of tracheal gas insufflation: comparison of continuous and phase-specific gas injection in normal dogs. Am Rev Respir Dis. 1993;148:561–568.)
Figure 22-2
Partial pressure of CO2 in alveolar gas (PaCO2) vs. duration of tracheal gas insufflation (TGI) as a percentage of expiratory time. Comparison of experimental data (boxes) with mathematical model (line). VT = 500 mL, Re = 5 cm H2O · L-1 · sec-1, and D = 0.33. VT, tidal volume; Re, compartmental resistance during expiration. Decreasing compliance (C), elevated dead space (VDan), and higher catheter flow (Qc) all magnify the effect of TGI on CO2 clearance. Of particular note is that, with rare exception, the maximal decrease in PaCO2 is observed at catheter flow durations between 40% and 60% of the expiratory phase, suggesting that more prolonged application of TGI accrues little additional benefit with regards to CO2 clearance over a broad range of impedance variables. (Used, with permission, Hota S, Crooke PS, Adams AB, Hotchkiss JR. Optimal phasic tracheal gas insufflation timing: an experimental and mathematical analysis. Crit Care Med. 2006;34:1408–1414.)
Continuous TGI increases alveolar ventilation more than inspiratory bypass or late-expiratory washout.16 Bidirectional continuous TGI delivery produces less hyperinflation than antegrade delivery, even with small diameter endotracheal tubes (ETT).22
Physiologic Effects
TGI reduces anatomic dead space and increases alveolar ventilation for a given frequency and VT combination. TGI’s main effect is to enhance CO2 removal by flushing the dead space from the carina to the Y of the ventilator circuit. The catheter and the TGI jet effect, however, oppose expiratory flow and favor air trapping at end-expiration and auto–positive end-expiratory pressure (auto-PEEP).6,11,23–28
TGI reduces PaCO2 during hypoventilation16,29–32 although TGI’s efficacy in lowering PaCO2 diminishes when an increased alveolar component dominates the total physiologic dead space.18,32,33 An inverse correlation between respiratory rate and PaCO234 indicates that lower breathing frequencies (or longer expiratory times) favor TGI efficiency (reductions in PaCO2 and physiologic dead space).
Increase in lung volume is a serious limitation of TGI and should be avoided. Solutions to minimize expiratory TGI-induced auto-PEEP include using lower TGI flows, delivering TGI during pressure-controlled ventilation (PCV), and optimizing mechanical ventilation during TGI. During PCV, a TGI-induced increase in airway pressure automatically results in decreased VT, and the lack of expiratory TGI-induced auto-PEEP is associated with less efficient CO2 elimination. Likewise, reducing TGI flow reduces CO2 clearance.27,35–37
Indications and Contraindications
Transtracheal oxygen therapy (TTO) administers gas directly into the trachea in nonintubated patients (Fig. 22-3). Long-term oxygen therapy is an established treatment for chronic lung disease with hypoxemia. Oxygen is usually delivered via a nasal cannula, irritating the nasal mucosa and the skin of the upper lip and ears. In 1982, Heimlich described a method for delivering oxygen directly into the trachea, bypassing the anatomic dead space and using the main airways as a reservoir.38 Numerous studies have since found clear clinical benefits for TTO, including reductions in hematocrit,39 pulmonary vascular resistance,40 work of breathing,41 dyspnea,42 and incidence of cor pulmonale.39 Other advantages are decreased oxygen cost and air pollution, decreased frequency of hospitalization, and improved exercise tolerance with better physical, psychological, and social function.43
The Spofford-Christopher Oxygen Optimizing Program is a four-step protocol for TTO:44
Patient evaluation, selection, and procedure preparation. Refractory hypoxemia and discomfort during maximal nasal cannula therapy are specific indications. Patients with chronic obstructive pulmonary disease (COPD) must also guarantee adequate bronchial hygiene and good pharmacologic control of airway activity throughout the program.
A tracheocutaneous fistula is created by a modified Seldinger technique or a surgical Lipkin procedure. Low predicted compliance with treatment (as in anxious patients) and neck anatomic alterations (as in severely obese patients) are strong contraindications to the tracheocutaneous fistula. The Lipkin method has fewer complications than modified Seldinger technique.45
Tract maturation management. Patients must learn to clean the catheter and prevent inadvertent catheter displacement.
Mature tract management. This phase aims to prevent complications and educate the patient.
The choice of equipment for oxygen supply and delivery depends on the setting (hospital or home) and on the patient’s ability to move; choices for home treatment include compressed gas cylinders, liquid oxygen, and molecular sieve oxygen concentrators. TTO usually requires an oxygen flow rate ranging from 0.25 to 1.5 L/min, but flow rates up to 2.9 L/min can be delivered through larger catheters to guarantee adequate oxygenation in severe refractory hypoxemia.46
Oxygen flow requirements with TTO are 25-50% lower than with continuous flow therapy via a nasal cannula; 0.5 L/min of oxygen by the catheter is equivalent to 4 L/min by nasal prongs, because the tracheal effect acts as an anatomic reservoir that stores oxygen during the last part of exhalation. Oxygen flow requirements during exercise are also reduced by approximately 30% with concomitant reduction in patient sense of dyspnea. Moreover, the equipment is lighter and mobility may be improved.
The main complications are subcutaneous emphysema, barotrauma, and bleeding from catheter misplacement. Patients receiving long-term TTO are at risk for catheter lumen occlusion by inspissated secretions, inadvertent catheter removal (particularly dangerous during the maturation phase), and chronic tract problems like infections and keloids.43
In the rare emergency situation in which it is impossible to guarantee adequate ventilation by facial mask, supraglottic device, or endotracheal intubation,47 usually because of laryngeal stenosis, foreign bodies, tumors, or facial trauma, transtracheal ventilation is recommended.48
Since the first report by Jacobs et al of a series of patients who were successfully ventilated in emergency conditions using a catheter placed through the cricothyroid membrane,49 many transtracheal emergency ventilation strategies have been reported.50 Current techniques consist of directly inserting a 14- to 18-gauge intravenous catheter between the tracheal rings or through the cricoid membrane. The device is connected to a syringe and advanced through the skin until the airway lumen is punctured. After air aspiration confirms placement, the cannula is connected to the oxygen delivery system. Percutaneous transtracheal emergency ventilation is performed; dedicated kits51 or self-made devices can also be used.52
Although manual or automatic jet ventilation is the reference standard for oxygen administration during transtracheal emergency ventilation, many techniques have been proposed to deliver oxygen with or without the aid of a jet valve. Commercially available resuscitation bags require great effort to ventilate through a 14-gauge catheter and make it impossible to guarantee adequate VT.50 Tubing that supplies oxygen can be coupled with a three-way stopcock, effectively creating a manual jet valve; however, tank and wall oxygen using flow regulators do not provide sufficient flow rates and, consequently, despite acceptable oxygenation, lead to hypercapnia. Only when the flow regulator is placed in the “wide open” position, an estimated flow of 65 L/min can be generated and physiologic VT can be delivered.50 Experimentally, both bidirectional manual respiration valves and active expiration using ejector-based expiratory ventilation assistance have proved useful in conditions of high expiratory resistance and occluded upper airway.53–55
Complications of transtracheal emergency ventilation mainly result from the need to operate under emergency conditions and ensure ventilation as soon as possible.56 Bleeding and posterior tracheal wall lesions are the most common, although kinking and inability to ventilate patients have also been reported when standard intravenous catheters are used.57 Moreover, catheters equipped with safety systems sometimes do not allow a syringe to be connected. Other complications can arise with chronic therapy (e.g., infection and tracheal stenosis).43
In conclusion, TTO can be an effective and safe alternative to nasal prongs for long-term home oxygen administration, and transtracheal ventilation using commercially available intravenous catheters and manual jet ventilation valves or wall oxygen supply can ensure emergency lung ventilation.
In patients with end-stage pulmonary disease and chronic CO2 retention, continuous insufflation of fresh gas (oxygen and/or air) through an intratracheal catheter has been used to provide continuous oxygen therapy, decrease oxygen flow requirements,7–9,58 provide a method for oxygen delivery, decrease dyspnea, and increase exercise tolerance.59 A continuous low flow (4 to 5 L/min) delivered to the tracheostomy tube reduces dead space, VT, and minute ventilation without affecting PaCO2 in the acute state and maintains or reduces PaCO2 in the chronic state, presumably by reducing dead space. In patients with the most severe forms of COPD, TGI resulted in oxygen consumption and CO2 production, as well as a less-demanding respiratory pattern.9
Patients with chronic respiratory failure may experience dynamic hyperinflation that may be relieved when minute ventilation decreases and expiratory time increases during high-flow insufflation. Brack et al60 found an almost immediate decrease in end-expiratory lung volume during high-flow insufflation compared to low-flow oxygen insufflation. To investigate whether this drop in end-expiratory lung volume was caused by active expiration, they performed a Konno-Mead analysis of rib cage-abdominal volume loops. The absence of a systematic change in loop configuration and the unaffected inspiratory and expiratory asynchrony indices did not suggest increased abdominal expiratory muscle recruitment during high-flow insufflation. Therefore, the drop in end-expiratory lung volume coinciding with the reduction in minute ventilation, respiratory rate, and prolongation of expiratory time after transition to high-flow insufflation was most likely caused by the reversal of dynamic hyperinflation (Fig. 22-4).
Figure 22-4
Time series of inductive plethysmographic rib cage (RC), and abdominal (AB) signals along with instantaneous lung volume (Sum) during transtracheal insufflation of oxygen at 1.5 L/min (low-flow insufflation, top left, A) and with an oxygen-air mixture at a rate of 15 L/min (high-flow insufflation; top right, B). Note the marked reduction of respiratory rate and end-expiratory lung volume (vertical line with arrow) during high-flow insufflation. For two breaths during low-flow insufflation and high-flow insufflation (marked with asterisks, respectively) rib cage versus abdominal volume loops (bottom left, C), and flow-volume loops (i.e., time derivative of the sum volume vs. the sum volume loops) (bottom right, D) are plotted. The loops at lower volumes in bottom left, C, and bottom right, D, correspond to high-flow insufflation. In bottom left, C, the major downward and minor leftward displacement of the loops with high-flow insufflation indicates that the drop in lung volume was predominantly related to deflation of the rib cage. The closed and open circles in bottom left, C, correspond to end-expiration and end-inspiration, respectively. During high-flow insufflation (top right, B), the expiratory flow approaches zero at end-expiration, as indicated by the small, horizontal arrow in bottom right, D. In contrast, during low-flow insufflation (top left, A), inspirations commence before expiratory flow has ceased (large arrow, bottom right, D), suggesting dynamic hyperinflation. (Used, with permission, Brack T, Senn O, Russi EW, Bloch KE. Transtracheal high-flow insufflation supports spontaneous respiration in chronic respiratory failure. Chest. 2005;127:98–104.)
One of the most important features of TGI is that it can maintain normocapnia or a given level of PaCO2 while VT is decreased, allowing a reduction in minute ventilation. Therefore, TGI can be used to decrease the forces acting on the lung and thereby minimize ventilator-induced lung injury in patients with acute respiratory distress syndrome (ARDS).36,61–63
Experimental studies on lung injury show that TGI results in lower ventilator requirements (airway pressures, VT, and dead space), more favorable alveolar surfactant composition, and a more favorable histologic trend than conventional mechanical ventilation.64,65 Similarly, in experimental bilateral or unilateral lung injury, the combination of TGI and partial liquid ventilation is more effective than conventional mechanical ventilation or either modality alone (Fig. 22-5).66–69 In this regard, TGI helps partial liquid ventilation to offset diffusional issues, remove partial pressure of arterial carbon dioxide (PaCO2), and decrease the demands for ventilator gas pressures and VT. Perfluorocarbon liquids provide cytoprotection and low-pressure mechanical support to the atelectatic lung, and these combined effects may offset lung inflammation associated with higher VT. The combined application of TGI and partial liquid ventilation, however, is still in the early experimental stages.
Figure 22-5
Representative photomicrographs (×100) of lung sections obtained at 4 hours after lung injury and stained with hematoxylin and eosin. The samples were from nondependent (NDep) and dependent (Dep) regions for mechanical ventilation (CMV, A and B), partial liquid ventilation (PLV, C and D), continuous tracheal gas insufflation (TGI, E and F), and combination (PLV + TGI, G and H) groups. Intraalveolar and interstitial inflammation and hemorrhage, atelectasis, edema, and exudation were severe, especially in the dependent regions in CMV group. Dependent region damage was significantly less in the PLV and in PLV+TGI groups. (Used, with permission, Guo ZL, Liang YJ, Lu GP, Wang JC, Ren T, Zheng YH, Gong JY, Yu J. Tracheal gas insufflation with partial liquid ventilation to treat LPS-induced acute lung injury in juvenile piglets. Pediatr Pulmonol. 2010;45:700–707.)
In patients with ARDS who are ventilated with a permissive hypercapnia strategy,63 the combination of increasing respiratory rate to the limit of inducing auto-PEEP, elimination of unnecessary instrumental dead space, and reduction in external PEEP when TGI-induced auto-PEEP increases (to maintain the total PEEP constant) seems a suitable pressure-limited ventilator strategy in combination with TGI36,70 (Fig. 22-6).
Figure 22-6
Changes in PaCO2, inspiratory plateau airway pressure (PPLAT), PEEP, and PaO2 induced by optimized mechanical ventilation (OPTIMV), expiratory washout (EWO), and the combination of OPTIMV and EWO in six patients with severe acute respiratory distress syndrome. Extrinsic PEEP had to be reduced by 5.3 ± 2.1 cm H2O during EWO and by 7.3 ± 1.3 cm H2O during the combination of OPTIMV and EWO, whereas it remained unchanged during OPTIMV alone. Plateau pressure did not change significantly, suggesting that lung hyperinflation was not produced. In patients with severe ARDS, the combination of OPTIMV and EWO has additive effects and resulted in partial pressure of arterial carbon dioxide (PaCO2) levels close to normal values. (Used, with permission, from Richecoeur J, Lu Q, Vieira SRR, et al. Expiratory washout versus optimization of mechanical ventilation during permissive hypercapnia in patients with severe acute respiratory distress syndrome. Am J Respir Crit Care Med. 1999;160:77–85.)
In ARDS, high-frequency oscillation (HFO) improves oxygenation relative to conventional mechanical ventilation. Mentzelopoulos et al recently examined whether the combination of HFO and TGI (HFO-TGI) results in better gas exchange than standard HFO and conventional mechanical ventilation.71
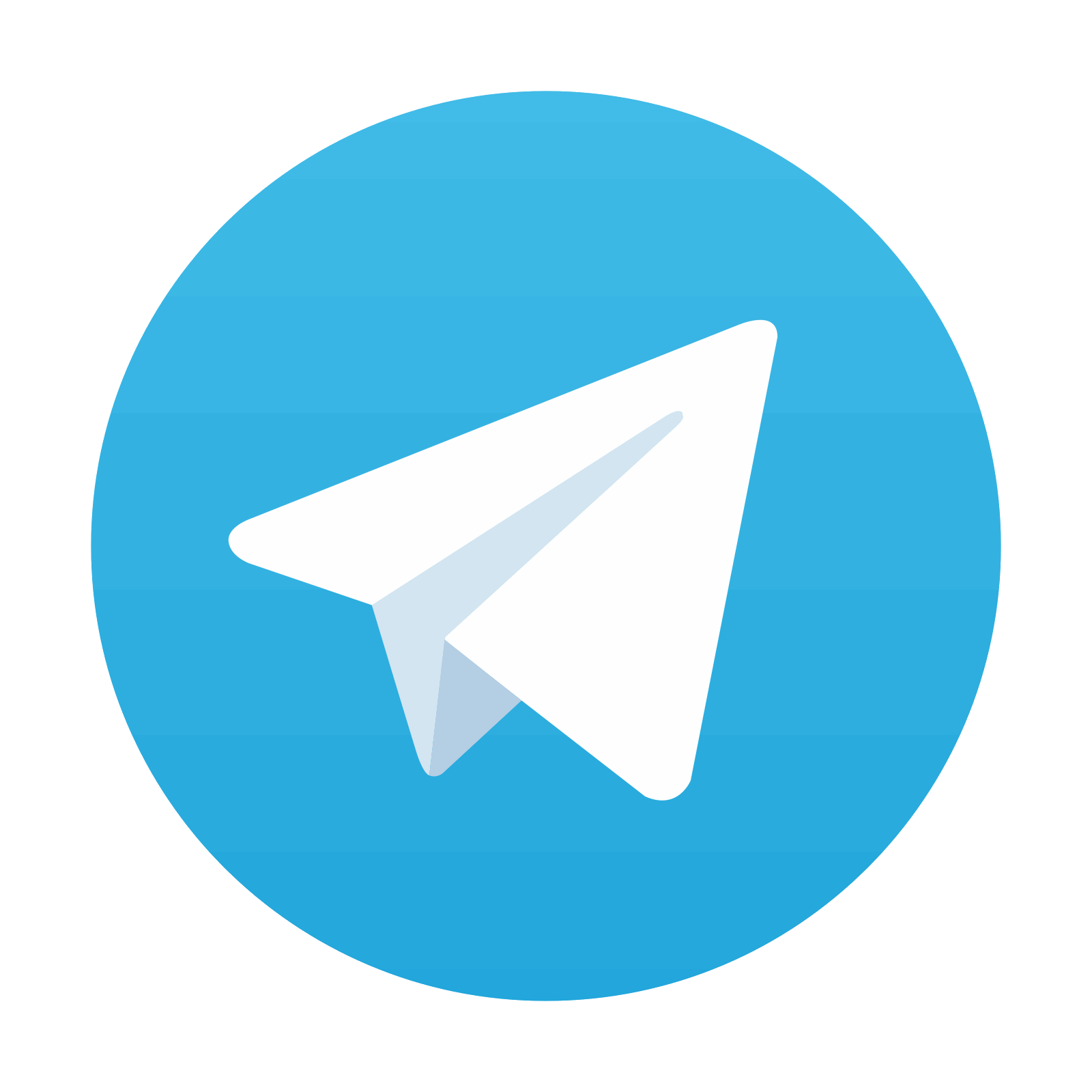
Stay updated, free articles. Join our Telegram channel

Full access? Get Clinical Tree
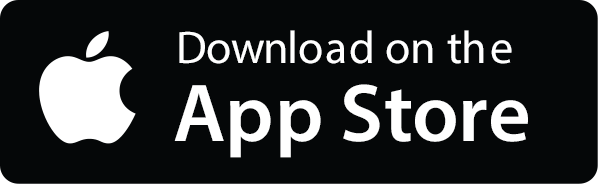
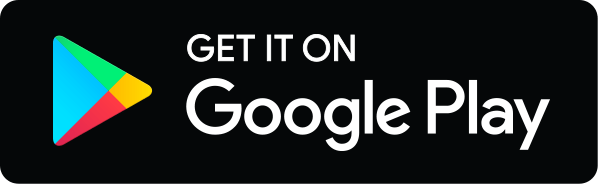
