Toxicology in Emergency Cardiovascular Care
Allan R. Mottram
Timothy B. Erickson
When the responsible toxic agent is known, the clinical picture is often predictable and directed therapy can follow standard initial resuscitation. In patients who present with an unknown or multiple drug ingestion, the clinical picture can become clouded by inconsistent physical findings that do not fit a particular toxidrome. Regardless of etiology, the initial resuscitation is similar to that of other critically ill patients and is guided by clinical presentation. But, the critically ill poisoned patient with shock or cardiac arrest may not respond to usual therapies and often requires nonstandard approaches. For example, these patients may require prolonged durations of cardiopulmonary resuscitation, medications not commonly used in resuscitation, larger doses of commonly used drugs, and avoidance of certain drug therapies.
-
General approach to the poisoned patient
-
Specific considerations for emergency cardiovascular care (ECC) providers on cardiovascular toxicities of poisons
-
How to use a methodical approach focusing on aggressive supportive care to optimize care of the critically ill poisoned patient
-
Periarrest and arrest evidence-based alterations to the principles of advanced cardiac life support (ACLS) for patients with suspected poisoning
Introduction
Prescribed and illicit xenobiotics can cause direct and indirect cardiovascular and cardiopulmonary toxicity when taken in overdose. When the responsible agent is known, the clinical picture is often predictable, and directed therapy can follow standard initial resuscitation. In patients who present with an unknown or multiple drug ingestion, the clinical picture can become clouded by inconsistent physical findings that do not fit a particular toxidrome.
Regardless of etiology, the initial resuscitation is similar to that of other critically ill patients and is guided by clinical presentation. Following provision of this care, obtaining a detailed history, a thorough physical examination, and review of available laboratory and diagnostic information can narrow the differential diagnosis. Attention can then be directed toward minimizing bioavailability of unabsorbed toxin, enhancing elimination, use of antidotes, and obtaining toxicology consultation.
The approach to the critically ill poisoned patient is guided by available evidence in the literature. Considering this, it is important to understand the limitations of that evidence. The nature of poisoning and overdose does not lend itself to well constructed clinical trials. The heterogeneity and relative infrequency of life-threatening overdose limits the accumulation of useful data. As such, the majority of literature upon which guidelines are based include case reports, short case series, retrospective analyses, and animal studies. With an awareness of these limitations, this chapter discusses the differences in the approach to the acutely poisoned critically ill patient as compared with the nonpoisoned critically ill patient and reviews the most commonly encountered cardiotoxins, highlighting key diagnostic, pathophysiologic, and management issues.
Approach to the Poisoned Patient
Aggressive supportive care in an intensive care environment that adheres to the principles of ACLS is essential to the management of critically ill poisoned patients. However, drugs taken in overdose often do not follow standard pharmacologic parameters. Absorption, distribution, and elimination may be accelerated or delayed. Patients who appear critically ill may recover quickly with aggressive supportive care; conversely, patients who initially appear well may rapidly decompensate. A select group of patients may benefit from advanced decontamination and enhanced elimination procedures such as activated charcoal, whole-bowel irrigation, multiple-dose activated charcoal, urinary alkalinization, and hemodialysis. A few selected cardiotoxic agents have specific antidotes that may be indicated in critically ill patients, but this is the exception rather than the rule.
The general approach to the diagnosis and management of the poisoned patient can be described using a two-pronged model as described in Figure 29-1.1 In practice, the two prongs occur simultaneously. The left side of the diagram begins with basic emergency medical care directed at the ABCs (airway, breathing, circulation). The mnemonic DONT stands for dextrose, oxygen, naloxone, and thiamine. In most potentially poisoned patients, a rapid blood glucose measurement should be obtained and any derangements corrected. Supplemental oxygen, naloxone, and thiamine should be provided in appropriate cases. The various methods of decontamination should be considered in any poisoned patient. The exact method used should be based on each individual clinical situation. Once a poisoning has been identified, methods of enhanced elimination should be considered. Focused therapy involves antidote administration when appropriate, with aggressive supportive care tailored to the poison in question. Finally, in managing any poisoned patient, it is prudent to consider early consultation with a toxicology service or regional poison center for further guidance. The right side of the diagram focuses on obtaining the patient’s history, performing a focused physical examination with attention to toxidrome recognition, and deciding on appropriate diagnostic testing. The two algorithms occur simultaneously and are integral to the diagnosis and management of the poisoned patient.2,3
In considering cardiotoxic xenobiotics, it is essential to obtain an electrocardiogram early following presentation. This step may uncover acute myocardial infarction related to cocaine, dysrhythmias related to cardioactive steroids, antihypertensives, or antidysrhythmic agents, and conduction abnormalities related to sodium, potassium, and calcium channel blockade. The remainder of this chapter reviews key presentations of poisoned patients, including the toxicologic differential diagnosis and appropriate interventions (see Sidebars 29-1 and 29-2). The chapter concludes with an in-depth review of specific commonly encountered drugs, poisons, and the appropriate treatments.
ACLS-Oriented Differential Diagnosis for the Poisoned Patient
|
ACLS-Oriented Interventions for the Poisoned Patient
|
General Considerations for the Poisoned Patient
Approach to Advanced Cardiac Life Support
The general principles of ACLS apply to the poisoned patient; however, there are fundamental differences. The critically ill poisoned patient with shock or cardiac arrest may not respond to usual therapies and often requires nonstandard approaches. For example, these patients may require prolonged durations of cardiopulmonary resuscitation, medications not commonly used in resuscitation, larger doses of commonly used drugs, and avoidance of certain drug therapies. Early consideration of invasive therapies such as dialysis and circulatory assist devices may be warranted.4

Airway Control
Airway control of the poisoned patient should be considered just as with nonpoisoned critically ill patients, with two caveats. In a patient with a clinically significant opioid overdose, the practice of routine endotracheal intubation before naloxone administration is not recommended.4 When opioid overdose patients have respiratory insufficiency with a detectable pulse, an attempt at opioid reversal before tracheal intubation is warranted provided that adequate ventilatory support can be provided with bag-valve-mask. Patients with opioid-induced cardiac arrest or impending cardiac arrest should have aggressive airway management, including intubation prior to consideration of naloxone. Finally, patients in whom gastric lavage is being considered should not be intubated solely to facilitate this procedure.
Dextrose
One of the most common causes of altered mental status is hypoglycemia. Prolonged hypoglycemia may result in significant morbidity, with permanent neurologic deficits. All patients with altered mental status require rapid bedside blood glucose determination and appropriate intervention. If rapid blood glucose testing is not available, it is acceptable to administer intravenous (IV) dextrose empirically. Following treatment of hypoglycemia, the blood glucose must be monitored for recurrence. This is a critical action in sedated or intubated patients, as they will not demonstrate the clinical clues that hypoglycemia has recurred.
Naloxone
Naloxone is the preferred reversal agent for opioid toxicity even though it has a shorter duration of effect (45–60 minutes) than heroin (4–5 hours). There is debate over which route of administration is preferred; however, if IV access is possible, it should be utilized. The endpoint objectives for opioid reversal are adequate airway reflexes and ventilation, not complete arousal. Acute, abrupt opioid withdrawal may increase the likelihood of complications, such as aspiration pneumonitis, pulmonary edema, ventricular arrhythmias, and severe agitation and hostility. Opioid-induced pulmonary edema occurs in 1% to 3% of patients with heroin overdose.5,6,7 The etiology is unclear but may be attributable to opioid-induced capillary leak or a sympathetic surge in the setting of profound hypoxia. Sympathetic surge has been demonstrated in opioid-addicted patients following naloxone administration, and animal studies have demonstrated this surge from hypercapnia, which is exacerbated by naloxone.5,6 This effect may be abated through ventilatory support prior to naloxone administration or by the use of graduated smaller doses of naloxone.7
When naloxone is given in incremental doses of 0.1 mg, the average naloxone reversal dose is 0.2 mg IV.7 In emergencies, a slow, incremental rate of administration is less satisfactory. For these cases, the recommended initial dose of naloxone is 0.4 to 0.8 mg IV or 0.8 mg intramuscularly (IM) or subcutaneously (SQ). In communities where abuse of naloxone-resistant opioids is prevalent, larger initial doses of naloxone may be appropriate. When opioid overdose is strongly suspected or in locations where fentanyl or its derivatives are prevalent, titration to total naloxone doses of 6 to 10 mg may be necessary.8 The majority of patients recover well following timely reversal of opioid intoxication with naloxone.9,10,11 The duration of action of naloxone is shorter than that of most opioids, and a subset of patients may have recurrent symptoms.12,13,14 Therefore, it is recommended that following arousal, all patients be observed beyond the duration of the naloxone effect to ensure their safety.
Flumazenil
Flumazenil is a competitive benzodiazepine receptor antagonist of limited utility in the overdose setting. It is generally understood to have the potential to induce seizures in the unknown overdose, as these patients may be benzodiazepine-dependent, have coingested a proconvulsant drug, or have an underlying seizure disorder. Healthy volunteers have experienced mild withdrawal symptoms in an experimental setting after only 1 week of nightly doses of diazepam (15 mg/70 kg), as have chronic low-dose benzodiazepine users.15,16 Animal studies have demonstrated withdrawal after 7 days, and seizure after 35 days, of daily benzodiazepine therapy followed by flumazenil.17 The use of flumazenil is not effective from a cost or resource-utilization perspective.18 In the undifferentiated comatose patient, flumazenil may resolve depressed mental status, but it carries a significant risk of seizure.19,20,21 Pediatric ingestions have been considered appropriate situations in which to utilize flumazenil. However, the same caveats that apply to adults apply to children. The restricted indications for flumazenil include iatrogenic overdose (except when the benzodiazepine was given for seizure), pediatric accidental ingestion, and paradoxical reaction to benzodiazepines.
Activated Charcoal
Activated charcoal is appropriate therapy within the first hour following ingestion for patients who have ingested a potentially toxic amount of drug and may be considered when such a patient presents beyond 1 hour postingestion, although efficacy decreases over time.22 It is generally considered safe and effective for gastrointestinal (GI) decontamination. However, routine administration in all ingestions is not indicated, as the administration of activated charcoal is not risk-free. Nausea, vomiting, aspiration, stercolith with intestinal perforation, and corneal abrasion have all been reported.23,24 Aspiration is the most significant complication and is usually related to altered mental status or seizure. Activated charcoal should be avoided in patients with depressed mental status, unprotected airway, hydrocarbon exposure, or GI hemorrhage. Other contraindications to the use of charcoal include ingestions of pesticides, hydrocarbons, acids, alkalis, iron, lithium, and solvents, as these agents are not well adsorbed.2
There are multiple activated charcoal products on the market. These are available with and without a cathartic, and the concentration of cathartic varies. Multiple doses of activated charcoal containing sorbitol have resulted in severe hypernatremic dehydration in both adult and pediatric patients.25,26,27,28 Therefore, in dosing activated charcoal in older children and adults, the first dose may contain sorbitol; subsequent doses should be sorbitol-free. Activated charcoal is commonly administered in a 50-g (or 1-g/kg) loading dose in adults. A more appropriate dose of charcoal is to provide at least a 10:1 ratio of activated charcoal to toxin.29,30,31 If this ratio cannot be achieved in a single dose, serial dosing may be required. Note that serial doses of activated charcoal to achieve an adequate total dose differs from multiple-dose activated charcoal (MDAC), which is discussed below in the section on enhanced elimination.
Gastric Lavage
Gastric lavage is an invasive procedure with significant inherent risk. There are appropriate indications for gastric lavage; however, one must weigh the risks and benefits carefully. Complications include aspiration, esophageal perforation, epistaxis, hypothermia, and death.32 The amount of toxin removed is variable and less successful over time. Even when implemented within one hour, the clinical benefit is unclear.33,34,35,36,37 Gastric lavage is contraindicated in patients with loss of airway protective reflexes, those with depressed mental status, following ingestion of corrosive agents or hydrocarbons, and those at risk for GI perforation or bleeding.32 It should never be used as a punitive measure, for nontoxic overdoses, or forced on combative or uncooperative patients. Patients should not be intubated solely to facilitate gastric lavage.
Gastric lavage may be considered in patients with potentially life-threatening ingestions (digitalis, verapamil, amitriptyline) that can be lavaged within 60 minutes of ingestion. When utilized, the procedure requires use of a large-bore (36–40 Fr) orogastric tube. Because the lumen of a standard nasogastric tube is of insufficient diameter, such a tube should not be used. The patient should be placed in the left lateral head-down position and 250-mL aliquots of warm fluid, such as tap water or normal saline, used to irrigate.2
Whole-Bowel Irrigation
Whole-bowel irrigation is based on the principle that large volumes of nonabsorbable polyethylene glycol traverse the length of the gut, decontaminating it by removing the gut contents via liquid stool.38 Volunteer studies show some evidence for decreased bioavailability and there are a number of case reports describing efficacy in the literature, although there are no well controlled clinical trials.39,40,41,42,43,44,45,46 Whole-bowel irrigation may be effective for ingestions of iron, heavy metals, lithium, drugs poorly adsorbed by activated charcoal, sustained-release or enteric-coated products, and drug-filled packets or other potentially toxic foreign bodies. Contraindications to whole-bowel irrigation are hemodynamic instability, unprotected or compromised airway, intractable vomiting, ileus, GI hemorrhage, and bowel obstruction.32 When whole-bowel irrigation is initiated, a polyethylene glycol solution is infused via nasogastric tube at a rate of 1 to 2 L/hr and continued until the rectal effluent is clear. In most cases, the gut should empty almost completely in 4 to 6 hours.2
Enhanced Elimination
Enhanced elimination is the removal of a drug from the body after absorption has occurred. It includes multiple-dose activated charcoal, urinary alkalinization, and extracorporeal measures such as hemodialysis, hemofiltration, and hemoperfusion.
Multiple-dose activated charcoal has been shown in volunteer studies to reduce the elimination half-life of carbamazepine, dapsone, phenobarbital, quinine, and theophylline.47 It has been evaluated for a number of other drugs and the data are either insufficient or indicate lack of efficacy. The mechanism is understood to be interruption of enteroenteral and enterohepatic circulation.48 There are no controlled studies in poisoned patients to confirm efficacy in reducing morbidity and mortality. The initial dose should be 50 to 100 g followed by an average of 12.5 g/hr. This may be dosed as 12.5 g/hr, 25 g every 2 hours, or 50 g every 4 hours.49 Sorbitol may be used in the first dose but not in subsequent doses due to the risk of severe electrolyte abnormality and dehydration.25,26,27,28
Alkalinization of urine promotes excretion of weakly acidic agents. It is the therapy of choice in salicylate toxicity for those who do not meet criteria for dialysis.50 There is mixed evidence regarding the use of urinary alkalinization for phenobarbital. It does enhance elimination of phenobarbital, although it cannot be recommended as first-line therapy, as multiple-dose activated charcoal has been found to be superior.50
The traditionally proposed mechanism of alkalinization is ion trapping at the renal tubules; however, there are inconsistencies with this theory.51,52 Regardless of mechanism, urinary alkalinization with sodium bicarbonate may be useful in salicylate overdoses. To alkalinize the urine, the nonalkalemic patient is bolused with 1 to 2 mEq/kg of sodium bicarbonate followed by a bicarbonate infusion. Alkalemic patients should not receive the bolus component. The infusion is prepared by adding 150 mEq sodium bicarbonate to 1 L D5W with 20 to 40 mEq of potassium.53 The infusion is started at 150 to 250 mL/hr and adjusted to reach a urinary pH of 7.5 to 8.0.54 Serum potassium should be maintained in the high-normal range, as hypokalemia will prevent urinary alkalinization.55 Patients should be monitored to avoid volume overload, especially the elderly and those with renal insufficiency or heart failure.
Extracorporeal measures for toxin removal include hemodialysis, hemofiltration, and hemoperfusion. Criteria that make a drug ideal for extracorporeal therapy include a small volume of distribution, low molecular weight, low protein binding, and water solubility. However, there is evidence that modern high-flux dialyzers are effective in removing larger molecules such as vancomycin as well as those which are significantly protein-bound, such as carbamazepine and valproic acid.56,57,58,59,60,61,62 Charcoal hemoperfusion has traditionally been promoted for use in theophylline, barbiturate, and carbamazepine overdose. However, several factors have caused this modality to fall out of favor: the declining frequency of barbiturates and theophylline overdose; complications of hemoperfusion such as thrombocytopenia; and evidence that modern high-flux hemodialysis equipment is equally effective.63 Hemofiltration following an initial hemodialysis session may be effective for drugs such as lithium and valproic acid to prevent a rebound effect.64,65,66
A common concern is that hemodynamically unstable patients are unsuitable for dialysis due to fluid shifts exacerbating hypotension. However, hypotension related to hemodialysis is caused by removal of volume and urea resulting in fluid shifts. Unlike the chronic dialysis patient, the toxic patient does not have a large urea burden and volume loss is minimal. The benefit of removing the toxin responsible for hypotension outweighs the concern of hypotension exacerbated by dialysis. Drugs or toxins with a large volume of distribution, such as lithium, can be difficult to dialyze.
Toxins that are commonly considered amenable to dialysis include salicylate, lithium, theophylline, methanol, and ethylene glycol. Prior to initiating dialysis, it should be clear that there are no effective alternative therapies and that the patient is unlikely to recover fully with supportive care alone.
Thermal Regulation
Drug-induced hyperthermia may be secondary to sympathomimetic or anticholinergic syndrome, serotonin syndrome, or neuroleptic malignant syndrome. Drug intoxication alone can produce clinically significant hyperthermia.67 When extremes of environmental temperatures are present, drug-related thermal dysregulation can exacerbate morbidity and mortality.68 It is essential that patients with altered mental status have a core temperature recorded early and be cooled rapidly if they are hyperthermic. The preferred method of cooling is evaporative with water mist and high-power fans. In the absence of this modality, ice baths and ice packs should be utilized. Conversely, patients with alcohol- or sedative-related toxidromes may become hypothermic. Temperature measurement and appropriate warming methods are equally important in these settings.
Toxicology Consultation and Referral
Early consultation with a toxicologist or poison control center is strongly encouraged. In cases of known poisoning, such resources can assist in refining the treatment course. Patients with undifferentiated altered mental status or suspected poisoning can benefit from the expertise of these resources, especially for unusual or severe cases. Referral to regional centers with capability for specialized care should be pursued early in the course of critically ill patients, such as those who may require dialysis, specific antidote therapy, hyperbaric oxygen treatment, or consideration for organ transplantation.
Pharmaceuticals Causing Cardiovascular Toxicity
Beta-Adrenergic Blocking Agents
Toxicology
The case mortality rate following beta-blocker overdose is much lower than that for calcium channel antagonists or digoxin; but in terms of absolute numbers, they are the second leading cause of death from cardiovascular medications.69 Beta1– and beta2-receptor antagonism, intrinsic sympathomimetic activity, and membrane-stabilizing activity are responsible for the clinical effects of these drugs. Alpha-antagonist activity is seen only with labetalol.70
The pharmacologic and toxicologic effects of beta-blocking drugs are mediated through indirect modulation of calcium secondary to inhibited adrenergic activation.71 Beta1 antagonism causes decreased cardiac contractility and conduction. Beta2 antagonism causes increased smooth muscle tone, which manifests as bronchospasm, increased peripheral vascular tone, and increased gut motility. Although many beta-blockers are beta1-selective at therapeutic doses, these drugs have both beta1 and beta2 effects in overdose.
Intrinsic sympathomimetic properties of some beta-blockers causes agonist–antagonist activity, which may blunt the bradycardic response in some patients.70,72 Drugs with intrinsic sympathomimetic activity include acebutolol, carteolol, oxprenolol, penbutolol, and pindolol. The membrane-stabilizing activity characteristic of some beta-blockers is a quinidine-like effect, resulting in inhibition of fast sodium channels, decreased contractility, and ventricular arrhythmias.73 This effect is additive to the beta1 toxic effects.
Review of cases in the literature suggests that beta-blockers with increased intrinsic sympathomimetic activity and decreased membrane stabilizing properties demonstrate less toxicity. Conversely, those with increased membrane stabilizing properties show greater toxicity.73,74,75 In fact, ingestion of a beta-blocker with membrane-stabilizing activity is second only to coingestion of a second cardioactive drug in predicting cardiovascular morbidity.76 Propranolol, a drug with significant membrane-stabilizing properties, may induce seizures in overdose.77 Additional drugs with membrane-stabilizing properties include acebutolol, betaxolol, and oxprenolol and to a lesser degree labetalol, metoprolol, and pindolol.78
Sotalol is a beta-blocker that has class III antiarrhythmic properties, and lacks intrinsic sympathomimetic or membrane stabilizing properties.79 In overdose, it can prolong the QT interval via inhibition of the delayed rectifier potassium current. This may result in ventricular arrhythmias including torsades de pointes. Each different beta-blocker may have only some of the described activities, and the clinical manifestations may vary.
The absorption, distribution, and elimination of beta- blockers vary with the preparation. Beta-blockers are available in both immediate- and extended-release preparations. As with all extended-release preparations, the onset of toxic effects may be delayed. Beta-blockers are rapidly absorbed, with 30% to 90% bioavailability. The elimination half-life varies from 2 to 24 hours, depending on the drug. In many cases, the half-life is significantly increased in overdose. Due to the rapid absorption of many beta-blockers, the onset of symptoms may be as soon as 30 minutes after ingestion, but it most commonly occurs within 1 to 2 hours.
The clinical presentation of acute beta-blocker overdose is suppression of the cardiovascular system. Deaths from beta-blocker toxicity are associated with bradydysrhythmias and asystole; ventricular arrhythmias are less common. Respiratory compromise during beta-blocker overdose can result from cardiogenic shock, decreased respiratory drive, or beta2-antagonist effects. Beta2 blockade causes bronchospasm and usually affects patients with previously diagnosed bronchospastic disease. Patients may present with noncardiogenic pulmonary edema, cardiogenic pulmonary edema, or exacerbation of asthma. Hypoglycemia has been reported and may occur secondary to beta2-mediated decrease in glycogenolysis and gluconeogenesis; however, it is not common unless the case is complicated by comorbidities or coingestants.80 Central nervous system depression may be caused by direct toxicity, hypoxia, hypoglycemia, or shock and may result in seizure.
Electrocardiographic manifestations of toxicity include sinus bradycardia and prolongation of the PR interval, second and third-degree atrioventricular (AV) blockade, and junctional and interventricular conduction delays.74,81 The QRS interval may be prolonged with ingestions of beta-blockers with membrane-stabilizing effects. Again, sotalol is unique among these agents in prolonging the QT interval via potassium channel effects. Propranolol and sotalol have been associated with ventricular arrhythmias.81
Management
Beta-blockers attenuate adrenergic tone and cAMP-dependent protein kinase pathways, resulting in disruption of cellular calcium flux. The consequences may range from negligible to life-threatening. Standard ACLS resuscitation followed by decontamination and enhanced elimination techniques apply, but attention must quickly turn to focused therapy.
Glucagon is the agent of choice for symptomatic beta-blocker ingestions resulting in hypotension or bradycardia.82,83 Glucagon binds to its own receptor site, triggering cAMP signaling pathways, resulting in L-type calcium channel activation. Thus, glucagon bypasses the cellular problem at the beta receptor.84 An initial bolus of glucagon is administered IV at a dose of 3 to 5 mg over 1 minute. If symptoms recur, a repeat bolus is given. An infusion may be started following the bolus dose, with the effective bolus dose being infused per hour. The initial effect is seen within several minutes, and should persist for 10 to 15 minutes. The pharmacodynamics of glucagon differ significantly from those of other commonly used adrenergic agents. This may be explained by compartmentalization of specific phosphodiesterases with different G-coupled receptors (i.e., glucagon receptor–coupled G proteins versus beta receptor–coupled G proteins), such that the cAMP generated by each receptor type is regulated in a unique way.85 Note that nausea and vomiting are common side effects of glucagons, particularly if administered rapidly.
Sodium bicarbonate has narrow applicability in the setting of beta-blocker toxicity. With toxicity due to drugs with membrane-stabilizing properties, blockade of fast sodium channels may prolong the QRS duration. Although there is a paucity of case reports and a lack of prospective data to support its use, sodium bicarbonate may be effective in select cases.86 It should be considered with QRS durations >120 ms and a suspected ingestion of a beta-blocking drug.87
Adrenergic agents are often effective in increasing heart rate, contractility, and peripheral vascular resistance. In cases of severe cardiovascular drug toxicity, large doses may be required.87 If the response to glucagon is inadequate, epinephrine and dopamine may improve both heart rate and blood pressure.77 Norepinephrine is effective in situations with low systemic vascular resistance; however, with the myocardial depression seen with severe beta blockade, alternative agents may be more efficacious.87
Hyperinsulinemia–euglycemia (HIE) therapy is an essential component of moderate to severe calcium channel blocker toxicity. Evidence supporting its use is derived from animal studies and a growing list of case reports and case series. Data supporting its use in beta-blocker toxicity is more limited. HIE has proven effective in both swine and dog models of propranolol toxicity.88,89 However, there is a paucity of human case reports demonstrating efficacy.90 Despite these limitations, HIE might be considered early in the course of resuscitation for patients with depressed myocardial function secondary to beta blockade who are refractory to other therapies.78,87 Adverse reactions are predictable and treatable; they include hypoglycemia, hypokalemia, and hypophosphatemia.
Bradycardia and hypotension refractory to pharmacologic intervention may benefit from temporary pacing through improvement in heart rate, although this intervention will not reverse the cardiac depression of severe beta-blocker overdose.91,92 Interventions such as intra-aortic balloon pump, extracorporeal membrane oxygenation (ECMO), or cardiac bypass are considerations for patients with toxicity refractory to all other therapy.92,93 Hemodialysis, hemofiltration, and hemoperfusion are rarely useful in the setting of beta-blocker overdose. Most of the beta-blockers have a large volume of distribution and are highly protein-bound, making drug removal by hemodialysis impractical. A few drugs, such as nadolol, sotalol, atenolol, and acebutolol, can be dialyzed; but information is limited to case reports.93,94,95 Hemodialysis can be considered in the setting of renal failure and hemodynamic instability due to a drug with low volume of distribution and low protein binding.
A patient with a history of immediate-release beta-blocker ingestion is observed on a cardiac monitor for 6 hours after ingestion.76,96 Patients who have signs of cardiovascular, respiratory, or central nervous system (CNS) toxicity are admitted to an intensive care setting. Patients with a history of ingestion of extended-release preparations or sotalol are admitted and monitored for 24 hours. A patient who ingested an immediate-release beta-blocker can be medically cleared after the 6-hour observation period if there are no signs of toxicity found by clinical examination, electrocardiography (ECG), or cardiac monitoring.
Calcium Channel Antagonists
Toxicology
Calcium channel antagonists account for more poisoning deaths than any other cardiovascular medication.69 This broad class of drugs is further classified as the dihydropyridines, phenylalkylamines, and benzothiazepines. Dihydropyridines include nifedipine, isradipine, amlodipine, felodipine, nimodipine, nisoldipine, and nicardipine. Verapamil is the sole phenylalkylamine, and diltiazem is the only benzothiazepine available.
The majority of available calcium channel antagonists work at the L-type calcium channel.97 These channels affect automaticity at the sinoatrial node, conduction through the AV node, excitation–contraction coupling in cardiac and smooth muscle, as well as pancreatic insulin secretion.71
Their clinical effects differ due to variations in binding location on the alpha1-receptor subunit, preferential binding at different resting cell membrane potentials, and as a function of channel state (activated, inactivated, resting).98,99,100 This receptor selectivity has clinical implications in that the dihydropyridines primarily result in vasodilation, whereas the nondihydropyridines have a more pronounced effect on cardiac conduction. Specifically, verapamil affects both the myocardium and the peripheral arterioles, causing decreased contractility, AV-node conduction, and peripheral vascular resistance. Diltiazem has less effect on peripheral vasodilatation and myocardial contractility than verapamil. Diltiazem slows AV-node conduction and causes coronary artery dilatation. Nifedipine, representative of the dihydropyridine class, has the greatest effect on peripheral vascular resistance, and also decreases contractility, with minimal effect on AV-node conduction. The unique properties of each drug define their therapeutic and toxic effects. However, in overdose, any of these drugs can cause peripheral vasodilatation, decreased AV conduction, and decreased myocardial contractility.
The various calcium channel blockers have slightly different pharmacokinetic properties. In general, bioavailability ranges from 20% to 85%, they undergo hepatic metabolism with extensive first-pass effect, are extensively protein bound, and with the exception of nifedipine, nimodipine, and nicardipine, have a large volume of distribution.97,101 The onset of action is 30 minutes and the half-life varies from 3 to 7 hours, but it can be greatly increased in the setting of overdose. It is important to be aware that sustained-release preparations can have a delayed onset of life-threatening effects due to their prolonged absorption time.
The clinical effects of calcium channel blocker overdose can be life-threatening. Slowing of the sinus node causes bradycardia. Slowing of conduction can cause heart blocks or asystole. Decreased contractility can cause heart failure and shock. Lowered peripheral vascular resistance leads to hypotension, which may exacerbate the hypotension associated with bradycardia, bradyarrhythmias, and heart failure. Hyperglycemia occurs frequently with significant overdoses and may correlate with the severity of poisoning.102 Patients with cardiac disease and those on other medications that suppress heart rate and contractility may develop severe toxic effects after mild overdose, or even at therapeutic doses. Neurologic and respiratory findings are usually secondary to cardiovascular toxicity and shock. Respiratory effects include decreased respiratory drive, pulmonary edema, and acute respiratory distress syndrome. Neurologic sequelae include depressed sensorium, cerebral infarction, and seizures. Nausea, vomiting, and constipation can occur. The most important GI consequence to recognize is a concretion of sustained-release capsules. In addition to causing bowel obstruction, the concretion can be a source of continued toxicity. Evacuation of sustained-release capsules from the GI tract may decrease morbidity and mortality if performed early in the clinical course.
Management
To effectively manage calcium channel antagonist overdose, it is essential to understand the location of the cellular lesion. Calcium flux is the central component regulating cardiac conduction and excitation–contraction coupling. The majority of interventions used to combat calcium channel aberrancies focus on modulation of cellular processes proximal to the lesion at the calcium channel. These interventions may reduce vagal tone, increase calcium concentrations, augment alpha- and beta-adrenergic tone, or increase cAMP via phosphodiesterase inhibition, all in an effort to enhance calcium channel function.90,103,104,105 However, when the calcium channel is severely poisoned, these upstream interventions will be minimally effective at best. Early consideration of HIE therapy in the nonresponding patient is essential.
Of the standard antidotes, IV calcium salts are readily available and easy to use. Increasing the extracellular calcium concentration may increase flux of calcium into the cell, thereby increasing cardiac conduction and contractility. Calcium is recommended as a first-line agent, although human data are mixed and the effect is inadequate in more severe poisonings.90,105,106,107,108,109,110,111 Two calcium salts are available: calcium gluconate and calcium chloride. Both of these are supplied in 10% solutions, but each contains a different quantity of calcium. Calcium chloride contains 1.3 mEq/mL of calcium and calcium gluconate contains 0.45 mEq/mL of calcium. The dose is repeated in 10 to 15 minutes for patients with persistent hypotension or bradycardia. Calcium salts primarily reverse hypotension due to vasodilation and may have little or no effect on heart rate or conduction.
For patients with symptomatic bradycardia or heart block, atropine may be helpful. However, accompanying hypotension is often related to peripheral vasodilation and will not respond to an increase in heart rate. Conversely, in a patient with stable blood pressure despite bradycardia, atropine will be of little benefit.
As with calcium salts and atropine, a trial of glucagon is indicated early in the course of resuscitation. However, in contrast to beta-blocker poisoning, glucagon does not bypass the cellular lesion but simply triggers cAMP signaling pathways in an attempt to overcome L-type calcium channel inactivation. The end result is that glucagon is not as effective in calcium channel antagonist poisoning as it is with beta-blocker poisoning. When used, the initial bolus is 3 to 5 mg IV over 1 minute. Clinical improvement will be seen in several minutes if it is going to be effective and will persist for 10 to 15 minutes. If symptoms recur, a repeat bolus and infusion may be used. Nausea and vomiting are frequent side effects of glucagons with rapid administration.
Vasopressors should be utilized early for patients who do not respond quickly to simple interventions such as IV fluid, calcium, atropine, and glucagon. Ideally, a vasopressor would be selected to counteract myocardial depression, vasodilation, or both, as determined by the patient’s hemodynamics. In a resuscitation scenario, this is challenging to do; and an agent with combined alpha and beta effects, such as dopamine or norepinephrine, is a more logical approach. More than one agent may be required.
HIE therapy is a novel approach to treating calcium channel antagonist overdose. The efficacy of HIE therapy is likely attributable to the metabolic effects of insulin. Animal models of calcium channel antagonist toxicity have demonstrated hyperglycemia, insulin resistance, blunted insulin secretion, impaired fatty acid utilization, and lactic acidosis.112,113,114 A shift in cardiovascular metabolism to favor glucose utilization seems to accompany marked improvements in hemodynamics, systolic and diastolic myocardial performance, and survival time.115 In humans, the evidence in support of HIE therapy is limited to case reports and case series.90,116,117,118,119,120,121 Despite this limitation, given the striking efficacy that has been observed with HIE, it should be utilized early in the course of resuscitation for severe calcium channel antagonist overdose.87,117,122
The therapy guideline for HIE is 1 U/kg of insulin as an IV bolus followed by 0.5 U/kg/hr as an IV infusion, with upward titration every 30 minutes as required.87 An IV dextrose bolus of 25 g followed by an infusion of 0.5 g/kg/hr should accompany the insulin infusion. If the patient is markedly hyperglycemic, the bolus may be omitted. Serial blood sugar determinations should be followed and the dextrose infusion adjusted accordingly. Potassium should be monitored and replaced to maintain serum potassium levels at 2.8 to 3.2 mEq/L.
Interventions such as intra-aortic balloon pump, extracorporeal membrane oxygenation (ECMO), or cardiac bypass are considerations for patients with toxicity refractory to all other therapy.123,124,125 However, it should be noted that the case reports in which these measures were used occurred prior to the advent of HIE therapy. Hemodialysis, hemofiltration, and hemoperfusion are not reported for calcium channel blocker overdose. Most calcium channel antagonists have a large volume of distribution, are highly protein-bound, and are subject to hepatic metabolism, making them poor candidates for extracorporeal removal.
Patients who have signs of cardiovascular, respiratory, or CNS compromise are admitted to an intensive care unit (ICU). Patients with a history of sustained-release ingestion are observed with cardiac monitoring for at least 24 hours. Those patients with no signs of toxicity, no history of sustained-release ingestion, and no ECG abnormalities can be observed for 6 to 8 hours after the time of ingestion. If they do not develop any signs of toxicity or ECG abnormalities during this period, they may be medically cleared.
Digoxin
Toxicology
Digoxin functions as a positive inotrope, increasing the force and velocity of myocardial contraction. In the failing heart, it can increase cardiac output, reduce pulmonary capillary wedge pressure, and decrease elevated ventricular end-diastolic pressures.126 As with many cardiovascular medications, this effect is mediated through changes in intracellular calcium.127
Digoxin inhibits the Na/K/ATPase, resulting in increased intracellular sodium, which in turn inhibits calcium efflux through the Na/Ca exchanger.128 Elevated intracellular calcium concentration resulting from supratherapeutic digoxin levels is likely responsible for the toxic effects.129 This may lead to dysrhythmias and a decrease in conduction through the sinoatrial and AV nodes.130 In addition, digoxin may augment parasympathetic stimulation, compounding the effect on sinoatrial and AV conduction.131,132 There is evidence for a sympathoinhibitory effect in patients with heart failure, and a sympathoexcitatory effect in normal controls.133
Potassium balance is a critical component of digoxin toxicity. In chronic toxicity, hypokalemia increases the probability of digoxin binding to the Na/K/ATPase secondary to phosphorylation and resultant conformational changes, thus exacerbating toxic effects at a given digoxin level. Severe hypokalemia (2.5 mEq/L) compounds the effect by inhibiting ion flux.128,134 In acute digoxin toxicity, the effects on the Na/K/ATPase predominate, with hyperkalemia resulting from inhibition of potassium influx.135 Hypomagnesemia, hypercalcemia, and hypothyroidism may exacerbate these effects.136
The onset of action of digoxin is within 30 minutes for IV formulations, with the peak effect seen in 1.5 to 5 hours and a half-life of 36 to 48 hours.137 It is primarily renally excreted, with limited gut metabolism. It is 23% protein-bound and has a large volume of distribution.101
Risk factors for digoxin toxicity vary based on age. Children are at risk from accidental ingestion, and dosing errors may occur when digoxin is taken therapeutically. The majority of adult patients will suffer toxicity related to an intentional ingestion.138 The elderly are at risk for chronic toxicity due to age-related alterations in pharmacokinetics, drug interactions, and comorbidities. Specifically, age-related declines in volume of distribution, renal insufficiency, hypoalbuminemia, and diuretic-related hypokalemia may result in digoxin toxicity.139
The clinical signs and symptoms of chronic digoxin toxicity may be very subtle. Fatigue, anorexia, nausea, and vomiting are the most common presenting symptoms, followed by dizziness, visual disturbances, abdominal pain, and diarrhea.140,141 Initial symptoms of an acute ingestion are typically nausea, vomiting, abdominal pain, weakness, confusion, and lethargy. Bradycardia, hyperkalemia, and vomiting are most predictive of significant ingestion in patients with acute digoxin intoxication.
It is important for the clinician to distinguish between the acute and chronic forms of toxicity. Acute toxicity generally responds well to therapy, while chronic toxicity is more refractory to treatment. The key is for the clinician to have a high index of suspicion. Chronic toxicity should be suspected in those patients presenting with vague, nonspecific symptoms who are taking or potentially taking digoxin. Malaise, headache, fatigue, and mild GI symptoms are subtle presenting symptoms commonly associated with digoxin toxicity.
Cardiovascular manifestations are the most important factor in determining morbidity and mortality. Symptoms include palpitations and dizziness, usually secondary to hypotension. A multitude of cardiac conduction aberrancies are commonly described. Ventricular ectopy and various AV blocks may be seen.140,141,142 Although no rhythm is pathognomonic, additional rhythms that have been observed include junctional rhythms, sinus bradycardia, sinus pause, atrial fibrillation, paroxysmal atrial tachycardia, ventricular tachycardia, and ventricular fibrillation. The often cited pathognomonic bidirectional ventricular tachycardia has also been reported with aconite poisoning.143
Therapeutic digoxin levels are between 0.5 and 2 ng/mL. Levels are obtained upon admission and again at 6 hours after ingestion to account for full distribution of the drug. Serum digoxin concentrations obtained between 6 to 12 hours postingestion usually correlate with the clinical course of intoxication. However, even when distribution and acute versus chronic intoxication is accounted for, it is difficult to predict who will benefit from antidotal therapy solely based on a single serum digoxin level.144 Given this difficulty, digoxin levels should be obtained for all patients who are or may be taking digoxin. The diagnosis of digoxin toxicity is considered if the serum level is >2 ng/mL in conjunction with clinical signs of toxicity. In the acute setting, the patient will have more dramatic clinical and laboratory findings than in the chronic setting. Patients with chronic toxicity will be more symptomatic, with lower corresponding digoxin serum levels. Key prognostic factors indicating increased risk for death are age >55, atrioventricular block, and potassium >4.5 mEq/L.145
Management
Patients with possible digoxin toxicity should be managed aggressively owing to the potential for morbidity and mortality.144 All patients should have IV access, frequent assessment of vital signs, and continuous cardiac monitoring. If the patient’s mental status is adequate, activated charcoal can be administered as a single dose. Multiple doses of charcoal have been reported to be of value for digitoxin or digoxin preparations and may be useful if antidotal therapy is not available.146,147
Digoxin immune Fab fragments are specific antidigoxin antibodies raised in sheep. Only the Fab fragment is used in order to decrease the risk of immunogenicity. This antidote has been used successfully in adults since the 1970s. Administration of the Fab fragments is indicated in cases of severe digitalis intoxication that is suspected by history, by a high level, or in those manifesting significant signs and symptoms of toxicity. Specific indications are based on absolute level, amount ingested, potassium level, and symptoms, as generated from case series and observational studies.148,149,150,151,152,153 In general, those with potentially life-threatening dysrhythmias, significant GI symptoms, altered mental status, or a potassium >5 mEq/L in the setting of elevated digoxin levels are candidates for antidote administration. Markedly elevated 6-hour levels even in the absence of symptoms should also prompt consideration of antidotal therapy.
Most patients who respond to Fab fragment therapy do so within 1 hour and have a complete response by 4 hours.149 Similarly, the effect on potassium concentration is delayed, which prompts the need for standard interventions for hyperkalemia in addition to Fab fragments. Glucose, insulin, and bicarbonate are appropriate. Calcium salts should be avoided because of the risk of exacerbating cardiotoxicity and precipitating cardiac arrest, although this risk has recently been disputed.154,155
Dosage of Fab fragments is based either on the acute ingestion of a known amount or on the steady-state serum concentration. Guidelines are available in the package insert or at http://us.gsk.com/products/assets/us_digibind.pdf. It is important to note that following treatment with Fab total digoxin levels will be markedly elevated. Free digoxin levels may be obtained that will correlate with the amount of drug that is biologically active.
Fab fragments are the cornerstone of therapy for the digoxin-toxic patient. However, the time to onset of Fab fragments mandates use of adjunctive therapies in the unstable patient. Atropine is appropriate for symptomatic bradycardia. The class Ib antiarrhythmics phenytoin and lidocaine may be effective. Phenytoin was first proposed as therapy for digoxin toxicity in the 1950s and was widely used until the advent of Fab fragments.156,157 Both suppress ventricular automaticity without affecting conduction, and phenytoin improves AV conduction.158
Hypokalemia should be corrected with careful attention to avoiding overcorrection. Magnesium therapy has been reported as efficacious in refractory ventricular arrhythmias.159,160 It should be repleted in deficient states, as hypomagnesemia may lead to refractory hypokalemia; but care should be taken in cases of renal insufficiency to avoid toxicity.
Cardiac pacing has not been shown to decrease mortality when compared with maximal medical therapy that includes Fab fragments, and it carries a high rate of complications.152,161 Cardiac pacing should only be used as a therapy once other interventions have proven futile or are not available. Hemodialysis, hemofiltration, and hemoperfusion do not aid in the management of digoxin toxicity.
Patients who are asymptomatic, without dysrhythmias, or with minor ingestions or undetectable to therapeutic levels 6 hours after ingestion may be medically cleared. Any patient that has signs and symptoms of digoxin toxicity should be admitted to a monitored bed. All patients with significant signs and symptoms and in whom the use of Fab fragments is being considered should be admitted to an ICU. Consultation with the poison control center, toxicologist, or cardiologist familiar with the treatment of these cases is recommended.
Plants Containing Cardiac Glycosides
Foxglove (Digitalis purpurea), lily of the valley (Convallaria majalis), common oleander (Nerium oleander), and yellow oleander (Thevetia peruviana) all contain digitalis-like glycosides. Ingestion of yellow oleander results in the most significant degree of toxicity. Of all varieties of oleander, yellow oleander has been responsible for the greatest number of fatal poisonings worldwide. All parts of the plant contain several cardiac glycosides and can produce a syndrome similar to digoxin poisoning when ingested.
The patient may manifest abdominal pain, nausea, vomiting, and diarrhea as well as weakness, bradycardia, and AV block. Because some of the plant glycosides are structurally similar but not identical to digoxin, a serum digoxin level may not be accurate.
Treatment with digoxin immune Fab fragments is safe and effective for toxicity caused by yellow oleander.162 The exact dose required is unknown, but a recent randomized controlled trial suggests that much higher doses may be needed than those used to treat digoxin overdose.162 As with many modern therapeutic agents, a lack of availability in the developing world has resulted in increased morbidity from this treatable illness.163 Fortunately, as an alternative, multiple-dose activated charcoal can be efficacious in this setting.164
Anticholinergic Agents
Toxicology
Acetylcholine is the neurotransmitter involved in the activation of both nicotinic and muscarinic cholinergic receptors. Nicotinic receptors are ligand-gated sodium and calcium channels. Muscarinic receptors are G protein–coupled.165 Three of these muscarinic receptors are stimulatory, increasing cAMP and intracellular calcium; two are inhibitory, reducing cAMP and intracellular calcium.
The anticholinergic toxidrome results from competitive inhibition of acetylcholine at muscarinic receptors. Muscarinic receptors are found throughout the CNS and in sympathetic and parasympathetic ganglia. This toxidrome is quite common and may result from both pharmaceutical preparations and various plant species.
Anticholinergic or antimuscarinic toxicity may be divided into central and peripheral effects. Central effects include alteration in mental status including delirium, hallucinations, agitation, and seizures. Peripheral effects include mydriasis, tachycardia, flushing, dry skin and mucous membranes, urinary retention, and decreased GI motility.
The onset of anticholinergic toxicity varies depending on the particular toxin but usually occurs within several hours. Although central and peripheral anticholinergic effects are commonly seen simultaneously, the central effects may occasionally persist after the peripheral effects have resolved. An often-used mnemonic to remember the clinical manifestations of anticholinergic toxicity is “Hot as a hare, Blind as a bat, Dry as a bone, Red as a beet, Mad as a hatter.”
The more significant components of anticholinergic toxicity is blockade of muscarinic receptors in the brain. The excessive CNS excitation that results clinically manifests as agitation, anxiety, delirium, lethargy, drowsiness, coma, and occasionally seizures. Peripheral blockade of muscarinic receptors also leads to cardiovascular effects such as tachycardia from antagonism of vagal tone, mydriasis with the inability to accommodate, decreased activity of sweat glands, hyperthermia, urinary retention, and reduced gut motility.
Drugs that may cause anticholinergic symptoms include antihistamines, antipsychotics, tricyclic antidepressants, and atropine. The antihistamine diphenhydramine is a common cause. In addition to its anticholinergic properties, it has type IA antidysrhythmic effects. A prolonged QRS interval with a subsequent prolonged QT may result, and severe cardiac dysrhythmias can occur.166,167,168 Cases of torsades de pointes have also been reported.169 Tricyclic antidepressants, which are discussed in a later section, behave similarly, although their cardiotoxic effects are more severe.
Serum drug concentrations are neither readily available nor helpful in the clinical setting. In overdoses, a screening acetaminophen level is indicated because products containing a combination of acetaminophen and antihistamine are common. Patients with severe psychomotor agitation and seizures should have a serum creatine kinase assessed because of the possibility of rhabdomyolysis. Patients who ingest the antihistamine doxylamine are particularly at risk for rhabdomyolysis.170,171,172 Injury is likely due to direct drug injury to striated muscle.173 Although most anticholinergic toxicity will result from ingestion, systemic anticholinergic toxicity has been reported from cutaneous absorption and from ocular exposure.174,175
Management
In most cases of anticholinergic toxicity, supportive care (i.e., benzodiazepines to treat agitation or seizures; cooling for hyperthermia) will be adequate therapy. Activated charcoal should be considered. Other associated complications, such as rhabdomyolysis from doxylamine, are also treated supportively with good outcome. However, cases of doxylamine-related renal failure requiring hemodialysis are reported.173 Wide-complex cardiac dysrhythmias from severe diphenhydramine toxicity should be treated with boluses (1–2 mEq/kg) of IV sodium bicarbonate to reverse the sodium channel effects.168,176,177
For patients demonstrating profound central anticholinergic toxicity (delirium, hallucinations, tachydysrhythmias, or seizures) unresponsive to traditional doses of benzodiazepines, physostigmine may be used as antidotal therapy. Physostigmine is an anticholinesterase agent that antagonizes the effects of anticholinergic agents. It is sufficiently lipophilic to cross the blood–brain barrier and can counteract both peripheral and central effects. The onset of action is within several minutes, and the half-life of the drug is approximately 15 minutes. For agents that have a prolonged anticholinergic effect on the CNS, such as scopolamine, redosing may be necessary. Physostigmine has demonstrated efficacy for severe anticholinergic syndromes and has proven to be more effective than benzodiazepines.178,179,180,181
Physostigmine was a first-line agent for anticholinergic poisoning in the 1970s, even in cases of cyclic antidepressant ingestions.182 In 1980, Pentel and Peterson published two cases of asystolic cardiac arrest following physostigmine use in the setting of tricyclic overdose; as a result, it became a rarely used drug.183 Currently, physostigmine is considered appropriate therapy only in patients with anticholinergic syndromes who meet strict criteria: normal QRS and QTc intervals, clear historical evidence that tricyclic antidepressants have not been ingested, and absence of mechanical bowel and/or urogenital tract obstruction. Relative contraindications are reactive airways disease, seizure, cardiac conduction abnormalities, and peripheral vascular disease.184
Adverse effects of physostigmine include cholinergic crisis with increased bronchial secretions, bradycardia, and seizures. Although seizures have been reported with physostigmine, they are also a complication of severe anticholinergic syndrome. The association between physostigmine, tricyclic antidepressants, and seizures is well reported in the literature.185,186,187
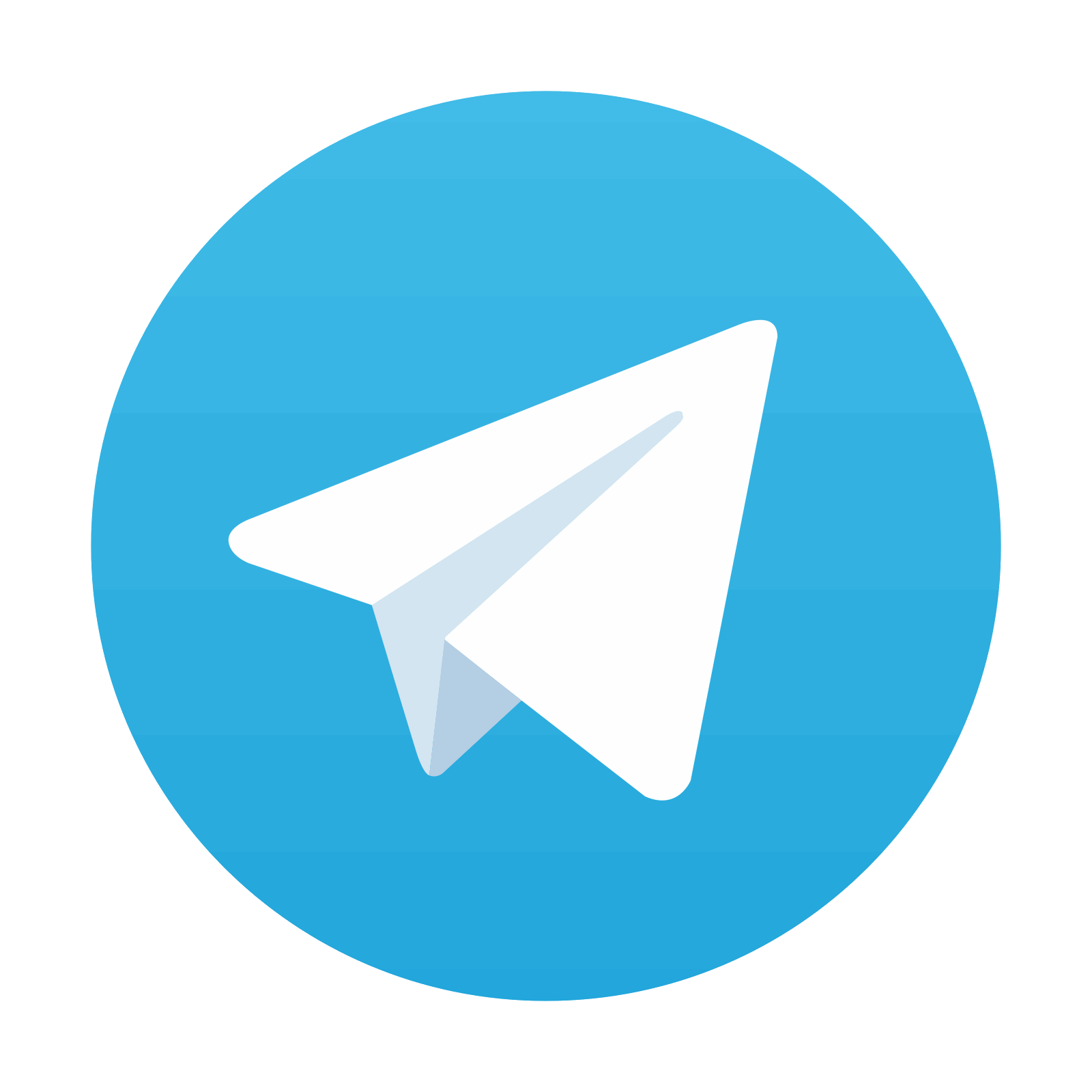
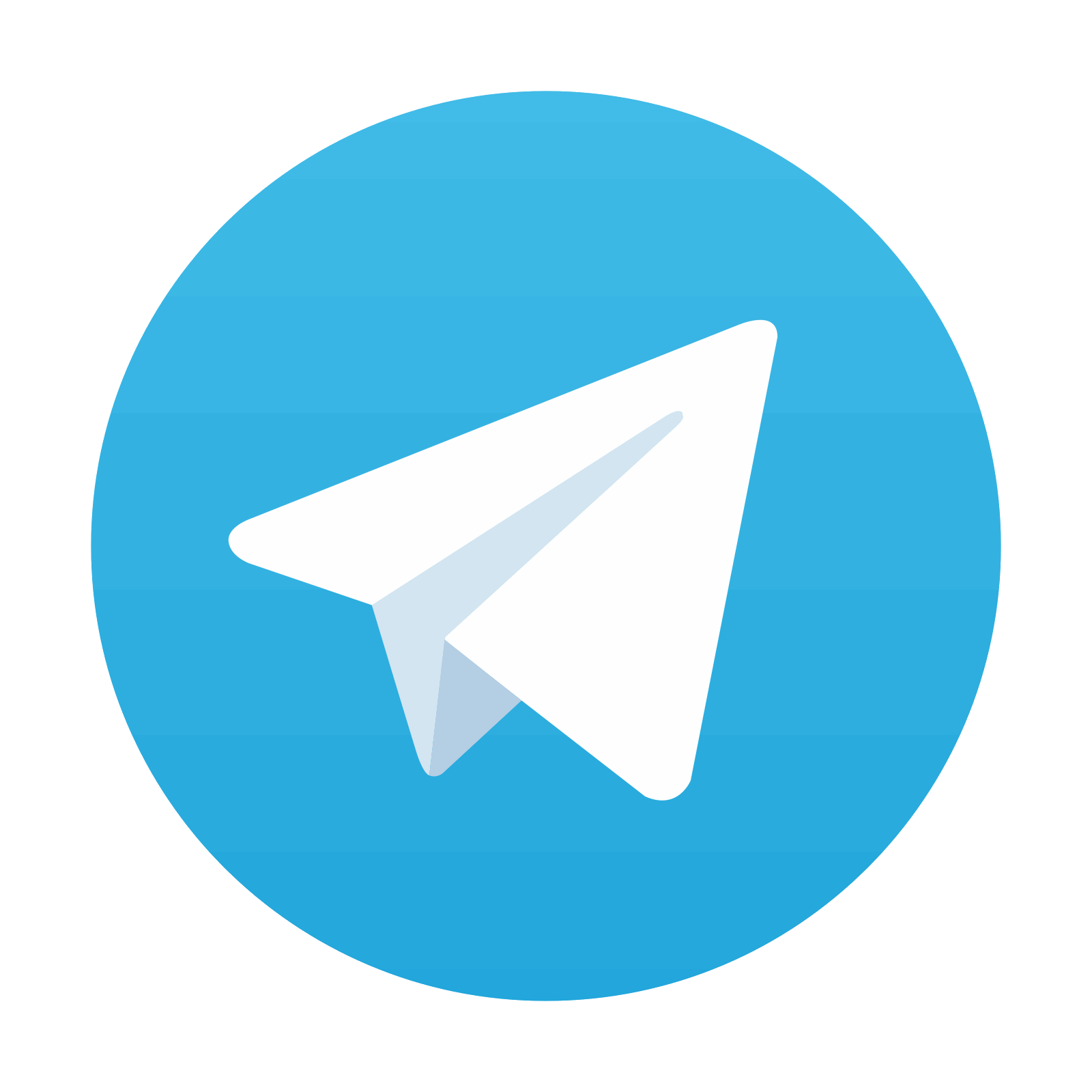
Stay updated, free articles. Join our Telegram channel

Full access? Get Clinical Tree
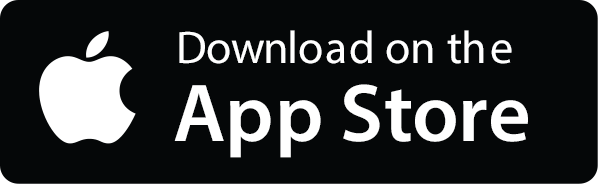
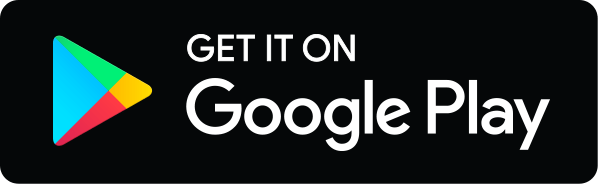