Fig. 7.1
Schematic: The dynamics of the initial response of various tissues following injury is often characterized by highly significant secondary cellular damage due to protracted ischaemia and a pro-inflammatory environment. (a) Tissue homeostasis as defined by the absence of hypoxia, acidosis or inflammation. (b) Relevant trauma will cause immediate cellular death (grey cells and areas) and haemorrhage. Also within few hours, pro-inflammatory activation of local cell populations (red cells) as well as the arrival of blood borne innate immune cells, predominately Fig. 7.1 (continued) of neutrophils, occurs. (c) Usually within 72 h post injury a progressive secondary tissue necrosis along with thrombosis and widespread inflammatory infiltrates is observed (‘second hit’). Also, loss of epithelial barrier function favours a microbial colonization of wounds increasing the risk of relevant infection. (d1) If tissue necrosis is extensive, it will become a burden requiring surgical debridement and eventually reconstructive measures. Strong evidence suggests that certain aspects of a pro-inflammatory innate immune response can prove detrimental to tissue survival by promoting, e.g. thrombosis or pro-apoptotic pathways. (d2) In contrast, if tissue necrosis is limited, a pro-inflammatory response will gradually give way to a recovery phase, characterized by the arrival of various, yet not well-defined cellular phenotypes on the scene. Conclusion: On top of the initial trauma significant secondary tissue damage commonly occurs. This opens a ‘window of opportunity’ for various strategies aimed at mitigating this secondary tissue damage, e.g. the modulation of an innate immune response
This may lead to a significant secondary tissue necrosis. It also induces tissue ischaemia, e.g. macro- or microvascular thrombosis, hypoperfusion, haemorrhage or relevant tissue swelling. A systemic acute-phase-response is largely defined by various humoral factors secreted by the liver and innate immune cells [2]. Typically within 72 h, a zone of demarcation within injured tissues has been established that essentially divides vital from non-vital tissue. Early on surgical debridement can provide a crucial role in mitigating a systemic inflammatory response (SIR) by reducing both the necrotic and microbial load of wounds. With sufficient perfusion established, wounds will soon enter a proliferative phase of wound healing primarily designed to achieve wound closure through wound contraction, newly formed connective tissue components and re-epithelialization, often at the cost of tissue function. Thus, scar tissue formation is a key feature of healing wounds in a remodelling phase. Clinical experience has shown that any measure that will shorten the recovery phase following tissue injury impairs functional and aesthetical outcome. Also, there has been a novel understanding of the inflammatory response. It clearly demonstrates that an abatement of inflammation is not just a decrease in pro-inflammatory stimuli. Successful wound healing requires an active, highly regulated process designed to counteract negative effects of prolonged pro-inflammatory signalling. A new type of phospholipid mediators have been described (resolvins and protectins) [3] and appear to affect pro-inflammatory and potentially harmful actions of polymorphonuclear leucocytes. Another important aspect has been the identification of various cellular phenotypes able to modulate pro-inflammatory responses. These enable tissue recovery and regeneration, most notably mesenchymal derived stem cell [4] and subpopulations of macrophages [5]. Cleary, one goal of future treatment strategies following relevant soft tissue injury should be to modulate an innate inflammatory response.
7.2 Challenges of Soft Tissue Injury Associated with Fractures
7.2.1 Principles and Classifications
Background
In general, any type of fracture is also associated with varying degrees of soft tissue injury. For the orthopaedic surgeon, accompanying soft tissue trauma is the most relevant factor to determine feasibility and indications for open vs. closed fracture reduction and fixation approaches. Over the last decades the principle of an anatomic fracture reduction has been changed to biological fixation techniques [6]. The term biological refers to leaving a zone of fracture unexposed to avoid further compromise surrounding soft tissue perfusion. Unstable fractures, maintain a state of soft tissue inflammation evidenced by continued swelling, pain and immobility.
Thus, from a plastic surgeon’s perspective, surgical incisions required for fracture reduction should be critically assessed, especially regarding the possibly of raising pedicled (e.g. arterial perforator-based) local tissue flaps later on.
Classifications
Various grading systems have been suggested with the intent of guiding clinical decision making, both in choice of surgical techniques as well as timing of reconstructive measures with an additional prognostic factor. Gustilo & Anderson classified ‘open fractures’ into three major types with additional subgroups [7]. Tscherne & Oestern et al. developed a more encompassing classification with a stronger focus on soft tissue injury which also includes ‘closed fractures’ [8]. In part for outcome comparability, more elaborate classification systems which account for various additional factors such as the extent of skin contusion, muscle injury, vascular and nerve injury et cetera in a check-list format have been continuously revised in recent years including the Hannover Fracture Scale (HFS) [9] and the AO/OTA Fracture and Dislocation Classification [10]. In addition, an appreciation of the ‘transferred energy’ leading to the observed pattern of injury can be highly useful in anticipating soft tissue recovery vs. the need for early intent reconstructive measures. Typically, a simple fall will generate forces around 100 (Ft/Lb, lbf = joule), a skiing accident up to 500 lbf, a gun projectile up to 2,000 lbf, and a motor vehicle accident at 18 miles/h (30 km/h) up to 100,000 lbf.
7.2.2 Hypoxia and Perfusion-related Complications
7.2.2.1 Tissue Hypoxia [11]
All molecular processes necessary for sustained cell survival require a steady generation of high energy transfer compounds, most notably of adenosine triphosphate (ATP). Depending on their metabolic activity, an eukaryotic cell poses limited capabilities of regenerating ATP in a non-oxygen–dependent anaerobic fashion. Prolonged hypoxia leads to metabolic decoupling (mitochondrial PaO2 <0.1–1 mmHg) and accumulation of acidic metabolites which in turn promote inflammation and tissue necrosis. Thus, a continuous monitoring of adequate oxygen supply to tissues in general following trauma is mandatory. Ischaemia (lack of perfusion), arterial Hypoxia (lack of lung dependent respiration), anaemia (lack of blood oxygen transport capacity) and intoxication (e.g. metabolic acidosis, CO-binding of haemoglobin, MetHb formation) have to be identified as different causes of tissue hypoxia. Limitations of compensatory mechanisms are reached in case of an acute drop of Hb values <10 g/dl, an arterial PaO2 <40 mmHg, a venous PaO2 <40 mmHg and oxygen HB-saturation values <85 %. From a surgical view point, control of haemorrhage outweighs all decision making immediately followed by re-establishment of arterial blood supply by means of vascular re-anastomosis and autologous or alloplastic grafts.
7.2.2.2 Compartment Syndrome
Sufficient tissue perfusion depends on an effective capillary perfusion pressure (P eff), defined as the difference (simplified) between a hydrostatic perfusion pressure (ΔP) to a colloid osmotic tissue pressure (Δπ) which in turn depends on a pressure gradient between a capillary (P c, π c) and an interstitial (P i, π i) space:
P eff typically ranges from 30 mmHg (4 kPa) at the post-arteriole entry level to −10 mmHg (−1.33 kPa) at the post-capillary venule level. In the poly-traumatized patient virtually all determinants of tissue perfusion, e.g. hydrostatic pressure, plasma protein levels will undergo significant changes resulting in overall interstitial fluid retention. A P eff above 30 mmHg usually results in an insufficient fluid return into the capillary system and thus soft tissue swelling. Soft tissue swelling itself will strongly increase Pi, and thus decrease ΔP and P eff. Although controversial [12], an estimate of P i can be directly measured using a puncture cannula attached to a pressure device. Pressure values above 35 mmHg are considered pathological by most authors and values above a systemic diastolic pressure inevitably will lead to severe tissue ischaemia. Muscles, especially of the lower leg are typically enclosed in strong fibrous sheets (syn.: fascia, loge, compartment) which will limit soft tissue swelling and thus are most susceptible to a relevant rise in P i, clinically termed compartment syndrome [13].
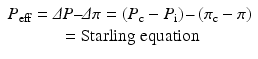
7.2.2.3 Venous Tissue Congestion and Thrombosis
Insufficient venous return is an often underestimated cause of serious sequels in the poly-traumatized patient. Venous congestion with a disturbed blood circulation between a superficial and a deep, inter-muscular vascular system, especially of the legs is a common feature accompanying post-traumatic tissue swelling. Although mechanisms of thrombosis formation are complex [14], three major contributing factors, originally defined by Virchow (1856), remain valuable in guiding a clinical rational: (1) post-traumatic state of heightened coagulability, (2) decreased venous velocity and (3) traumatic vascular lesions.
For vascular repair following traumatic vascular transection, based on a literature overview, venous re-anastomosis using standard end-to-end suture techniques can be considered the gold standard opposed to end-to-side techniques. Regarding post-surgery anti-coagulative therapy following free-flap tissue transfer, to the authors’ knowledge, various recommendations [15] but no widely evaluated and accepted guidelines exist. However, according to a survey by Xipoleas et al. [16], around 85 % of members of the American Society of Plastic Surgeons routinely use anti-coagulative therapy post-operatively, either low-fractionated heparin and/or aspirin. To the authors’ knowledge, no meta-analysis exists which supports the notion of clinically relevant anti-thrombotic effects of colloidal, e.g. dextrane-based i.v. therapy.
Regarding a generally increased risk of thrombotic events of poly-traumatized patients, the incidence of unnoticed below-knee deep venous thrombosis (BKDVT) has been stated between 40 and 80 % according to AWMF-consensus guidelines [17]. These result in an overall rate of about 10 % of above-knee propagation. Anti-thrombotic therapy, e.g. using low-fractionated heparin, hirudin, Danaparoid®, Fondaparinux®, Rivaroxaban® but not aspirin is considered as an effective prophylaxis. With heparin-derivatives, there is a need for a regular screening for heparin-induced thrombocytopenia (HIT I/II) and renal function. Activation of the ‘muscular pump’ by physiotherapy, active or passive early-functional movement of joints, compression stockings and various measures designed to reduce swelling have been shown to be effective in clinical level three studies or lower.
7.2.2.4 Decubitus
Peri- [18] and post-operative immobility exposes the poly-traumatized to a high, avoidable risk of pressure ulcers caused by prolonged periods of B.E.: ischaemic tissue compression around typical anatomical landmarks. These include the occipital region, shoulder blades, elbow region, sacral region, ischial tuberosity, trochanter major, fibula head and the heel. Repositioning measures every 2 h to avoid prolonged, localized tissue compression has been shown to significantly decrease the incidence of pressure sores [19]. Thus, measures of appropriate pressure distribution throughout periods of prolonged immobility is a mandatory part of patients’ care with national guidelines for diagnosis (stage I-IV) and management (NPUAP [20] or EPUAP) being revised on a regular basis. If manifested, surgical debridement and secondary defect coverage offers many challenges in terms of peri- and post-operative care due to a relatively high risk of relapse [21]. Additional surgical measures such as colostomy [22] prior to attempting a definite defect closure of sacral decubiti in order to reduce a bacterial load of wounds have been widely promoted.
7.2.3 Life Threatening Early Wound Infections
Even in skin abrasions, a significant rise in bacterial swab colony forming units is to be expected within 24 h following trauma [23]. Thus, in the poly-traumatized patient with fractures, an initial microbial swab evaluation is useful for the anticipation of (1) infections caused by environmental pathogens. These include Bacillus, Clostridium, Corynebacter, Pseudomonas or Actinobacter species, (2) fungal infections, e.g. Aspergillus species, (3) early onset bacterial infections, e.g. Streptococcus and Staphylococcus species or (4) pre-existing multi-drug resistant bacteria strain carriers, e.g. methicillin-resistant staphylococcus (MRSA). A switch from initially present body surface colonization or environmental wound contamination to nosocomial, hospital acquired bacterial strains (e.g. Staphylococcus, Escherichia coli, Proteus, Enterococcus, Bacteroides species) can be typically expected within 72 h post trauma, again advocating early definite surgical closure of wounds. However, to the authors’ knowledge, related to a risk assessment for possible wound infection, no evidence-based data exists which dictates delayed vs. primary wound closure [24]. Overall, staphylococcus species remain the most common cause of surgical wound infections [25].
Live threatening infections related to initial wound contamination are typically characterized by a sudden onset and rapid progression of soft tissue inflammation and necrosis. Thus, surgical decision making often is solely based on clinical presentation alone. They include: (1) Gas gangrene [26] (e.g. Clostridium perfringens), more defined by tissue-lytic toxin effects (e.g. alpha toxin) rather than bacterial load, can be anticipated in patients with an initial wound contamination with soil as well as with a diabetic, alcoholic and peripheral artery disease (PAD) predisposition. Here, the avoidance of ischemic wound conditions is paramount. A fulminant course of tissue necrosis, intra-tissue gas formation and emergency gram-staining should motivate early and extensive surgical debridement or amputation along with a supportive therapy, e.g. hyperbaric oxygen therapy and antibiotics. With manifestation in the genital region, usually a mixed infection with aerobic and anaerobic bacteria is present, termed Fournier’s gangrene (1883). (2) Necrotizing fasciitis [27] (e.g. Streptococcus pyogenes, Staphylococcus aureus, Clostridium perfringens, Bacteroides fragilis, Aeromonas hydrophilia) shares features with gas gangrene in that toxin effects will cause a sudden, fulminant progression of disease on a subcutaneous, epifascial plane but initial soft tissue reaction is often bland with only minor swelling or redness that does not correlate well with severe pain in the conscious patient. Early surgical intervention is mandatory with diagnosis usually confirmed by tissue histology. (3) Tetanus [28] (Clostridium tetani), due to extensive passive anti-toxin vaccination programmes is a rare condition in developed countries. However, because of its potential lethal cause; checking up-to-date vaccination remains a mandatory part of any initial wound management. (4) Botulism [29] (Clostridium botulinum), a lethal, toxin-defined disease is avoided by sufficient initial wound de-contamination and mitigated by early diagnosis with passive vaccination. (5) Erysipelas [30] (Streptococcus pyogenes) caused by a superficial, non-pyogenic infection of upper layers of the skin by streptolysin O/S exotoxin expressing strains can be readily treated following early diagnosis using antibiotics combined with antiseptic dressing regimes. A more severe, ‘bullous’ from that often requires secondary defect coverage can be distinguished based on the appearance of large areas of toxic epidermolysis [31].
7.3 Surgical Management
In contrast to an initial acute phase following trauma with a focus on fostering soft tissue recovery and preventing secondary tissue damage, both on a local and a systemic level, in a post-acute phase clinical reevaluation mandates a timely surgical approach of defects or soft tissue insufficiencies.
7.3.1 Acute Surgical Management
Radical surgical debridement of contaminated or critically damaged, non-viable tissue represents a widely accepted, mandatory part of any surgical first intent strategy. Following initial stabilization of vital body functions, both through intensive care management and surgical control of haemorrhage or acute brain damage, a close multidisciplinary approach is required.
Acute phase surgical measures comprise (1) the avoidance of prolonged ischaemia by securing sufficient arterial perfusion and venous return through re-anastomosis, evacuation of hematoma or decompression of muscular compartments; (2) decontamination of wounds using techniques such as excision of wound edges according to Friedrich (1889) [32], bursectomy, jet-lavage [33], versa-jet [34], open aseptic wound management [34] or the application of occlusive, vacuum-based dressings [35]; (3) additional measures to ensure a viable environment for the primary or secondary reconstruction of nerves, tendons or ligaments.
Soft tissue injury to the upper and lower extremities has been shown to be most susceptible to early infectious complications opposed to soft tissue injuries of the head, neck and genital region [36]. In general, primary wound closure should be attempted within 6 h following trauma which also coincides with an expected peak in tissue swelling.
7.3.2 Post-acute Surgical Phase
Comparable to an initial surgical debridement of traumatized soft tissue, a surgically motivated ‘second look’ strategy should occur.Given the post-traumatic inflammatory response [37–41], debridement and revision early after trauma has proven highly effective in improving overall patient outcome [42].
7.3.3 Early-Intent Defect Coverage
Background and Principles of Defect Coverage
A widely accepted guideline regarding a timely closure of wounds and defect coverage can be summed up by the ‘reconstructive ladder’ [43] concept (Fig. 7.2) in which the complexity of soft tissue injury directs the choice of surgical options. A more recent ‘reconstructive triangle’ [44] concept (Fig. 7.2) reflects a certain change in philosophy with more patient-centred view on certain defined goals of treatment and means to achieve them. Accordingly, decision making on how soft tissue injury and defect closure should be managed is based on an evaluation of overall safety, function and aesthetic form. This also includes two-timed approaches where early defect closure is achieved by technically simple measures such as split-skin grafting followed by more complex procedures such as functional myoplasty later on when the poly-traumatized patient has entered a rehabilitatory phase of treatment.
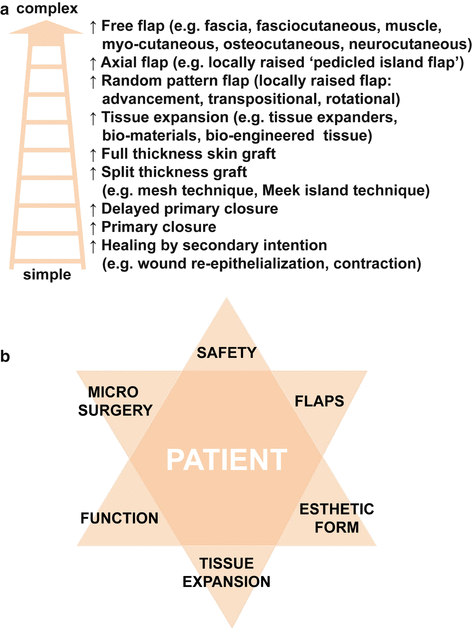
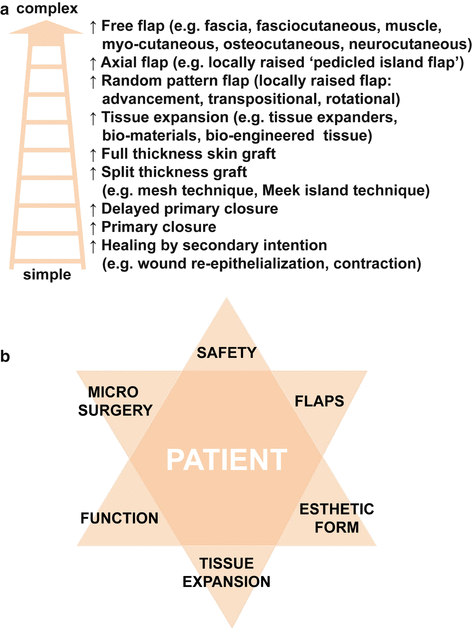
Fig. 7.2
Principles of soft tissue reconstruction. (a) The ‘reconstructive ladder’ concept has long been considered an important guideline for choosing different surgical approaches to wound closure. However, a perceived hierarchy ranging from ‘simple’ to ‘complex’ surgical procedures has been partly replaced by a rather undogmatic attitude aimed at combining all available techniques in order to achieve best possible results for a given patient represented by the (b) ‘reconstructive triangle’ concept which underlines different aspects and goals of surgical treatment. Also, a continued introduction of new aspects of bioengineering into the clinical context will continue to alter current treatment concepts
In general, if primary wound closure is not feasible, defect coverage can be achieved by two major principles.
First, by allowing the formation of granulation tissue which will lead to stable and well perfused wound(beds) which in turn can then be closed using various techniques of autologous skin grafting. This approach is usually limited to soft tissue defects that will not leave bone or tendons exposed. However, in recent years, development and application techniques regarding the use of biodegradable skin substitutes [45] such as MatriDerm® [46] or Integra® [47] have matured sufficiently to allow for coverage of exposed bone, muscle or tendons with stable and functionally satisfying results (Fig. 7.3).
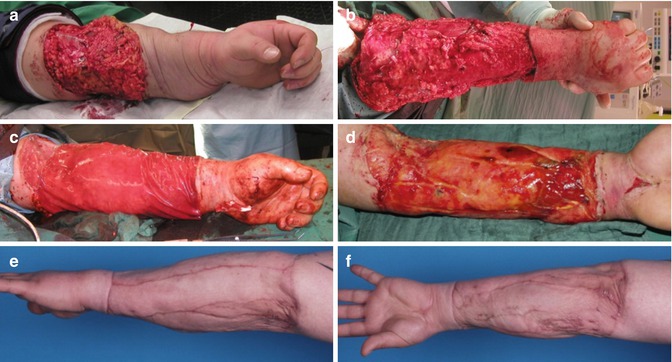
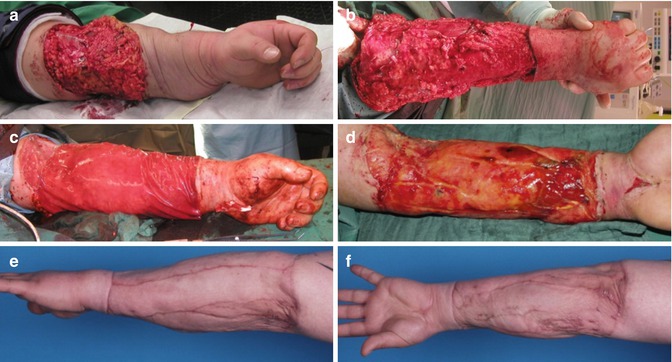
Fig. 7.3
Clinical Case: This 24-year-old patient was overrun by a motor vehicle and sustained (a) a degloving injury of the left lower arm (b) requiring debridement of the resulting soft tissue necrosis. (c) Defect coverage was performed using a collagen-glycosaminoglycan-based biodegradable matrix wound dressing (Integra™) which (d) when integrated acted as dermal substitute suitable for autologous split-skin grafting. This allowed the formation of (e, f) a levelled, stable new soft tissue sheath with unhindered sliding of underlying muscle and tendon
Secondly, any type of surgical measure that will ‘move’ a defined block of tissue from one area to another defines defect coverage using flaps. A block of tissue that does not incorporate a defined vascular pattern is called a ‘random pattern’ flap, as opposed to the ‘axial pattern’ or ‘island’ flaps with an identifiable vascular pedicle. Flaps with a vascular pedicle are most versatile since they allow for a safe mobilization of large tissue blocks both locally and distally as ‘free flaps’. A list of flaps commonly used for defect coverage is given in Table 7.1. The authors’ recommendation for defect coverage using flaps in defined anatomical areas is given in Fig. 7.4.
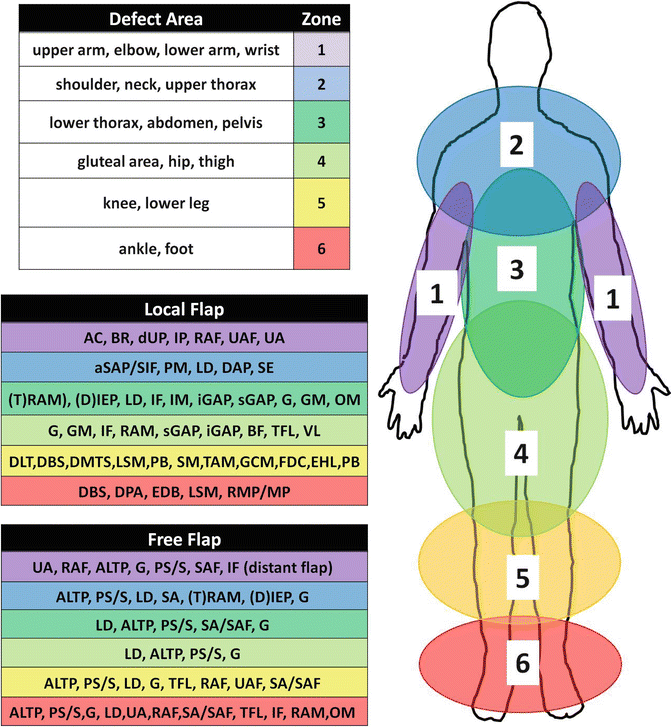
Table 7.1
Shown: List of commonly used flaps for soft tissue coverage associated with trauma
Flap-name (abbreviation) | Flap-type+ | Flap-size++ | Comments+++ | Literature |
---|---|---|---|---|
Upper extremity | ||||
Anti-cubital perforator (AC) | FC | s | Local | [63] |
Brachio-radialis muscle (BR) | M, MC | s | Local | [64] |
Deltoideus perforator (DAP) | FC | s | Local | |
Distal ulnar perforator (dUP) | FC | m | Local, “Becker flap” | [65] |
Interossea posterior artery (IP) | FC | s | Local | [66] |
Radial artery forearm (RAF) | FC | m | “Chinese flap” | |
Ulnar artery forearm (UAF) | FC | m | Local and free | |
Upper arm perforator (Lateral, medial, anterior, posterior UA) | FC | m | Local and free | |
Lower extremity | ||||
Antero-lateral thigh perforator (ALTP) | FC | l | Local and free | |
Biceps femoris muscle (BF) | M, MC | m | Local | [82] |
Distal lateral thigh (DLT) | FC | m | Local and free | |
Distally based sural (DBS) | FC | s | Local | |
Dorsalis pedis artery (DPA) | FC | s | Local | |
Extensor digitorum brevis muscle (EDB) | M | s | Local | |
Fibular osteocutaneous (FOC) | OC | m | Free | |
Flexor digitorum communis muscle (FDC) | M | s | Local | |
Gastrocnemius muscle (GCM) | M | s | Local, medial or lateral head | |
Gluteus maximus muscle (GM) | M, MC | l | Local and free | |
Gracilis muscle (G) | M, MC | m | Local and free | |
Inferior gluteal perforator (iGAP) | FC | m | Local | |
Lateral supramalleolar (LSM) | FC | m | Local, “fibular/peroneal artery perforator” | [106] |
(Reverse) medial plantar artery (RMP/MP) | FC | s | Local | |
Peroneus brevis muscle (PB) | M | s | Local | |
(Distal-medial thigh) saphenus (DMTS) | FC | s | Local, ‘saphena neuro-cutaneous’ | |
Soleus muscle (SM) | M | s | Local | |
Superior gluteal perforator (sGAP) | FC | m | Local | [100] |
Tensor fasciae latae muscle (TFL) | MC, FC | m | Local and free | [120] |
Tibialis anterior muscle (TAM) | M | s | Local | |
Vastus lateralis muscle (VL) | M, MC | m | Local | |
Thorax, abdomen, pelvis | ||||
(Para)scapular (PS/S) | FC, OC | l | Local and free | |
(Deep) inferior epigastric artery (perforator) (DIEP/IE) | FC | m | Local and free | |
Iliacus muscle (IM) | M | l | Local | [133] |
Inguinal flap (IF) | FC, OC | l | “Groin flap” | |
Latissimus dorsi muscle (LD) | M, MC | l | Local and free | |
Omentum major (OM) | l | Local and free | ||
Pectoralis major muscle (PM) | M, MC | Local and free | ||
(Trans-) rectus abdominis muscle (TRAM/RAM) | M, MC | m | Local and free | |
Serratus anterior muscle (fascia) (SA/SAF) | M, F, OC | l | Free | |
Superior epigastric artery (SE) | M, MC | s | Local | |
Supraclavicular island flap/anterior supraclavicular artery perforator (SIF/aSAP) | FC | l | Local | |
Free-style perforator flaps | FC | Local |
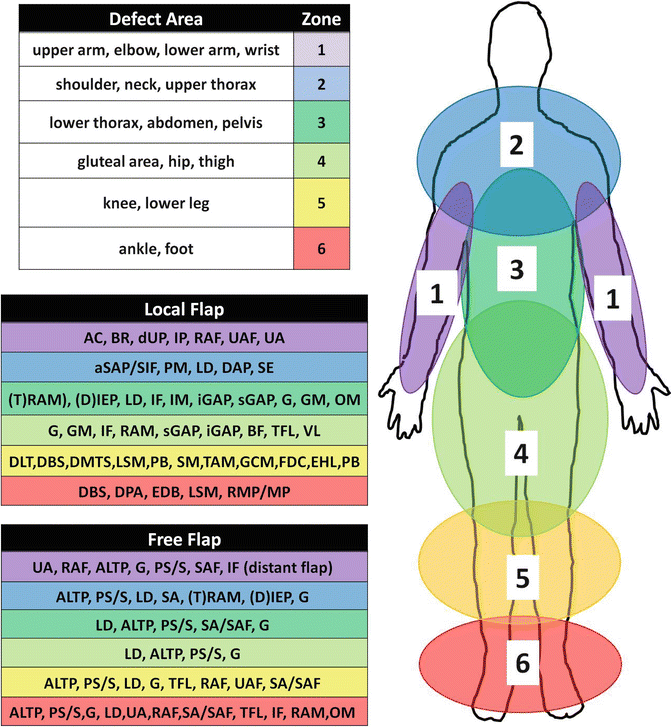
Fig. 7.4
Shown: A comprehensive listing of local and free tissue transfer for defined anatomical regions recommended by the authors (for abbreviations see Table 7.1)
Random pattern flaps are essentially synonymous with locally mobilized skin flaps. Standard skin flaps used for defect closure are further sub-categorized based on the underlying principle of mobilization as either advancement, transpositional or rotational. A key consideration of local tissue mobilization for defect closure is that unidirectional loss of tissue mobility (through defect) is best compensated for by tissue mobilization along a main vector that is perpendicular to a corresponding vector of reduced tissue mobility. Also, as a rule of thumb, the ratio between length and width of a randomly patterned flap should not exceed 3:1 for sufficient flap perfusion. This ratio can be modulated using, e.g. a ‘bridge’ flap technique, effectively creating two pedicles.
A detailed study of human vascular anatomy underlying skin perfusion such as performed by C. Manchot (1889) and M. Salmon (1936) proved to be essential for the design of larger skin or fascio-cutaneous flaps. Human vascular anatomy (opposed to, e.g. rodent models) rarely allow larger cutaneous flaps to be raised based on a vascular pedicle that runs along the subcutaneous plane. Instead, skin perfusion is predominantly ensured by ‘perforators’ which originate from a deeper muscle plane. Thus, the vascular anatomy of skin is closely linked to that of muscle. Regarding free transfer of muscle tissue, a highly valuable classification system of muscular artery perfusion was introduced by Mathes and Nahai [48]. Nowadays, so-called perforator flaps play a dominant role whenever coverage of soft tissue defects of significant size and depth is required. As already mentioned, perforator flaps are essentially fascio-cutaneous flaps where a substantial area of skin and underlying fat tissue are dependent on a vascular pedicle that usually perforates perpendicular to the surface plane of the flap. Design and surgical preparation of a perforator flap requires a concise knowledge of a perforator’s relation to the vascular system of an underlying muscle. As elaborated by Cormack and Lamberty [49], a perforator-based flap pedicle might require transmuscular, septal, subfascial preparation techniques. Knowledge of perforator anatomy is also invaluable in raising osteo-myo-cutaneous flaps. In order to minimize donor site co-morbidity due to flap raising, muscle flaps are usually reserved for large and deep defects requiring a certain volume and an increased ‘mechanical resistance’ of the flap. Also, commonly used muscle flaps do cause only minor functional deficits. A ‘monitor skin island’ as part of a muscular flap is still considered the most practical way of monitoring adequate flap perfusion despite various alternative approaches or monitor devices available on the market (e.g. O2C™ [50]).
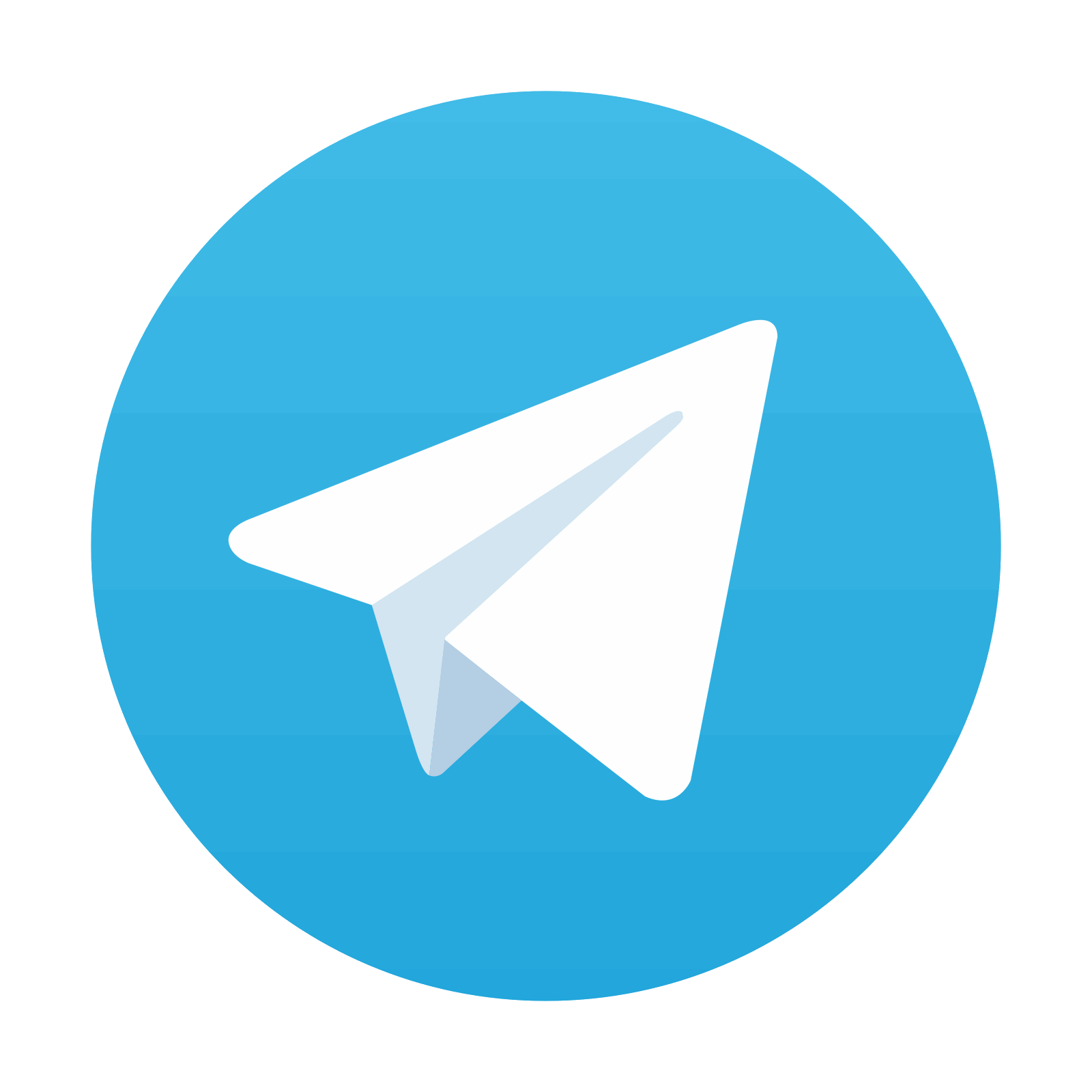
Stay updated, free articles. Join our Telegram channel

Full access? Get Clinical Tree
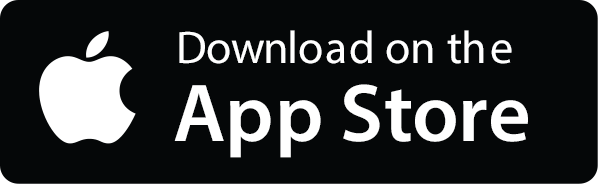
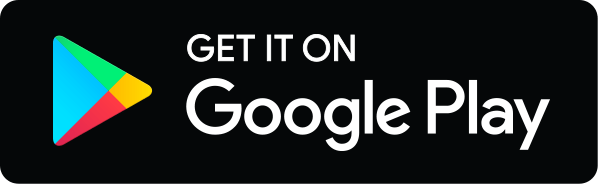