Proteinase-activated receptor (PAR)
Activating protease
Activating peptide
PAR antagonist
PAR1
Thrombin
TFLLR
FR-171113
FXa
RWJ-56110
Trypsin
RWJ-58259
Granzyme A
BMS-200261
APC
SCH-79797
Gingipains-R
PAR2
Trypsin
SLIGKV
FSLLRY-NH2
Tryptase
SLIGRL
GB-83
Factor VIIa
FLIGRL
GB-88
Factor Xa
ENMD-1068
Neutrophil elastase
Granzyme A
Matriptase
Acrosien
Gingipains-R
Proteinase-3
PAR3
Thrombin
None
None
PAR4
Thrombin
GYPGQV
Pepducin P4pal-10
Trypsin
AYPGKF
tcY-NH2
Plasmin
YD-3
Bacterial gingipains
Cathepsin G
1.1 Proteinase-Activated Receptor: Activation, Signal Transduction and Desensitisation
1.1.1 Activation
Unlike classical ligand-binding receptors, PARs have a unique mechanism of receptor activation. All PARs contain a discrete serine proteinase cleavage site within their extracellular N-terminus which when cleaved unmasks a new amino acid terminal known as a tethered ligand. The exposed tethered ligand then binds to the second extracellular loop of the receptor to initiate the recruitment of G proteins and other signalling molecules to the intracellular domains of the receptors. PAR3 is a distinct receptor in that it acts as a cofactor for thrombin-mediated activation of the PAR4 receptor (Nakanishi-Matsui et al. 2000). For practical purposes, short synthetic peptides derived from the sequence of the unmasked tethered ligand have been developed which can also activate PARs in the absence of receptor proteolysis (Fig. 1). These experimental tools allow for the pharmacological study of PAR processes in vitro and in vivo without the confounding multifactorial effects of proteolytic activity. Hence, these short activating peptides have been used in studying the role of PARs in different physiological and pathophysiological situations (Russell and McDougall 2009). In addition to protease activation, some proteases can also disarm PAR signalling by cleaving the N-terminus downstream of the receptor-activating site, thereby detaching the tethered ligand making it unavailable for receptor activation (Fig. 1). A recent report confirmed that serine proteinase enzymes such as neutrophil elastase can disarm trypsin-mediated PAR2 signalling and at the same time activate PAR2 signalling selectively via a mitogen-activated protein kinase (MAPK) pathway, without triggering an elevation in intracellular calcium levels (Ramachandran et al. 2011).
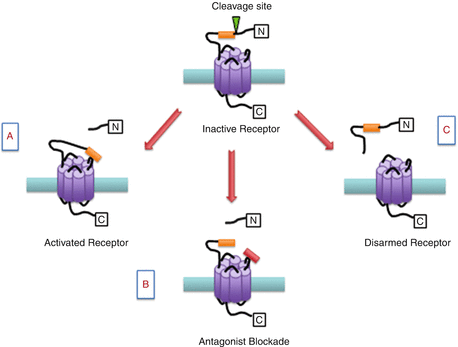
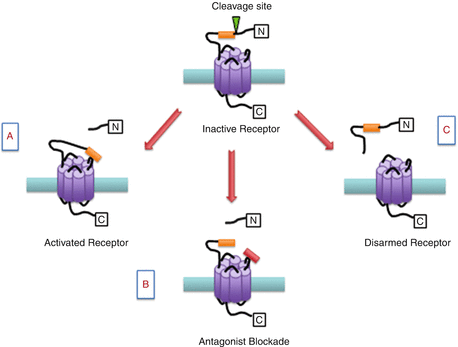
Fig. 1
Schematic representation of proteinase-activated receptor (PAR) modulation. (a) Inactive PARs can be cleaved by a protease revealing a tethered ligand (orange box) which binds to the active domain of the same receptor leading to cell signalling. (b) The PAR binding site can be blocked with a small molecule antagonist (red box). (c) Alternative proteases can remove a segment of the N-terminal including the binding domain leading to disarmament of the receptor
1.1.2 Signalling
PAR1
PARs couple with G proteins and activate multiple pathways and hence they can regulate various cellular functions. PAR1 interacts with several α-subunits particularly G1α, G12/13α and Gq11α. G1α leading to inhibition of adenylyl cyclase (AC) to reduce cyclic adenosine monophosphate (cAMP) (Benka et al. 1995). Gq11α activates phospholipase Cβ (PLCβ) to generate inositol trisphosphate (InsP3), which mobilises Ca2+, and diacylglycerol (DAG), which activates protein kinase C (PKC). G12/13α couples to guanine nucleotide exchange factors (GEF), resulting in activation of Rho, Rho-kinase (ROK) and serum response elements (SRE) (Ossovskaya and Bunnett 2004). Recent studies suggest that the cleaved N-terminal domain of PAR1 is released and exhibits biological activity in certain settings and has been termed ‘parstatin’ (Duncan and Kalluri 2009). PAR1 can activate the MAPK cascade by transactivation of the EGF receptor, through activation of PKC, Phosphoinositide 3-kinase (PI3K), Pyk2 and other mechanisms (Coughlin 2000).
PAR2
PAR2 couples to Gqα and stimulates the generation of InsP3 and mobilisation of Ca2+ in PAR2-transfected cell lines (Bohm et al. 1996). In the case of enterocytes and transfected epithelial cells, activation of PAR2 leads to arachidonic acid release and the generation of prostaglandins E2 and F1α (Kong et al. 1997). This suggests that PAR2 cleavage involves the activation of phospholipase A2 and cyclooxygenase-1 (Kong et al. 1997). Also, it has been reported that PAR2 activates MAP kinases ERK1/2 and weakly stimulates MAP kinase p38, although c-Jun amino-terminal kinase is not activated (Belham et al. 1996; DeFea et al. 2000).
PAR3 and PAR4
PAR3 does not signal autonomously and is only considered as a cofactor for PAR4 activation by thrombin (Nakanishi-Matsui et al. 2000). However, in a recent report, it was observed that PAR3 can elicit Rho- and Ca2+-dependent release of ATP from lung epithelial A549 cells and PAR4 couples to Gq and G12/13 signalling effectors in order to signal through G proteins (Seminario-Vidal et al. 2009).
1.1.3 Desensitisation
The desensitisation mechanisms for the PARs are distinct but poorly understood. Phosphorylation of activated PAR1 takes place to uncouple it from G proteins and G protein-coupled receptor kinase 3 or 5 (GRK 3 or 5) which enhances PAR1 phosphorylation. Moreover, the β-arrestin 1 mechanism also contributes to desensitisation of PAR1. In the case of PAR2, the main mechanism of uncoupling is phosphorylation by PKC and other kinases, by binding both β-arrestin 1 and β-arrestin 2 leading to a rapid uncoupling from G protein signalling at the cell surface. The mechanisms contributing to the desensitisation of PAR3 and PAR4 are unknown; however, it has been suggested that receptor internalisation may contribute to the termination of PAR4 signalling (Soh et al. 2010).
1.2 Proteinase-Activated Receptor: Role in Physiology and Disease
1.2.1 Cardiovascular System
Protease signalling through PARs contributes to both normal homeostasis and various cardiovascular disease states such as thrombosis and atherosclerosis. Evidence that PARs are involved in cardiovascular homeostasis comes from the observation that the receptors are expressed on platelets, endothelial cells and smooth muscle cells (Nelken et al. 1992; Takada et al. 1998). PAR1, but not PAR2, is expressed on rat cardiac fibroblast (Steinberg 2005). A study, however, showed the presence of PAR2 in rat cardiac fibroblast (Murray et al. 2012). PAR1 and PAR4 mainly coordinate thrombin-mediated platelet aggregation. After activation, PAR1 rapidly transmits a signal across the plasma membrane to G proteins, which results in the formation of platelet–platelet aggregates. It also causes stimulation of Gq proteins which culminates in a rapid rise in intracellular calcium and activation of the GP IIb/IIIa (αIIbβ3) fibrinogen receptor. PAR4 is cleaved and signals more slowly but, despite its slower response, generates the majority of the intracellular calcium flux and does not require additional input from ADP receptor to form stable platelet clumping. Blockade of thrombin binding to mouse PAR3 or knockout of the PAR3 gene inhibited mouse platelet aggregation indicating the importance of PAR3 for thrombin signalling in mouse platelets. However, when mouse PAR3 was overexpressed in mouse platelets, it did not trigger thrombin signalling. Mouse platelets express both PAR3 and PAR4, so Matsui et al. carried out a series of experiments and showed that PAR3 and PAR4 interact with each other and PAR3 functions as a cofactor in cleavage and activation of PAR4 by thrombin (Nakanishi-Matsui et al. 2000). PAR2 is expressed by numerous cell types within the cardiovascular system. Functional PAR2 expression has been demonstrated on vascular endothelium, smooth muscle cells and cardiomyocytes (Steinberg 2005; Sabri et al. 2000). Armed with this information, a group of researchers carried out an investigation which involved evaluation of the role of PAR2 in a cardiac ischaemia/reperfusion injury model (Antoniak et al. 2010). It was demonstrated that PAR2 deficiency reduced myocardial infarction and heart remodelling after ischaemia/reperfusion injury. In another study, it has been reported that PAR2 contributes to the pathogenesis of heart hypertrophy and failure. In a similar study, it was demonstrated that cardiomyocyte-specific overexpression of PAR2 led to pathological heart hypertrophy associated with cardiac fibrosis (Antoniak et al. 2013). Pathological remodelling of the heart in αMHC-PAR2 mice was accompanied by increased ANP: Atrial natriuretic peptide, BNP: B-type natriuretic peptide and βMHC expression and decreased MHC expression (Antoniak et al. 2013).
1.2.2 Nervous System
Various reports available in the literature show that thrombin changes the morphology of neurones and astrocytes, induces glial cell proliferation and even exerts, depending on the concentration applied, either cytoprotective or cytotoxic effects on neurones (Wang and Reiser 2003). Thrombin induces various neuronal changes such as neurite retraction, cell rounding, NMDA receptor potentiation and protection from cell death which are all mediated by PAR1 (Jalink and Moolenaar 1992; Turnell et al. 1995; Gingrich et al. 2000). PAR1 agonists also stimulate proliferation and shape changes in astrocytes, which results in the release of endothelin-1 and nerve growth factor and to inhibit the expression of glutamate receptors (Beecher et al. 1994). When thrombin was infused into the brain, it has been shown to reproduce inflammatory signs observed after injury in the CNS (Suidan et al. 1996). Although expression of the other two thrombin receptors (PAR3 and PAR4) in the brain has also been detected by several studies, the physiological role of PAR3 and PAR4 in neuronal differentiation is presently unknown (Wang and Reiser 2003). In a recent study, animals subjected to transient middle cerebral artery occlusion followed by reperfusion showed an increase in PAR2 expression. Also, there was significant decrease in the neuronal expression of phosphorylated extracellular signal-regulated kinase (p-ERK) in PAR2 KO mice (Jin et al. 2005). The p-ERK is mainly responsible for regulating cell survival under normal and pathological conditions (Bonni et al. 1999). In the case of PAR2 KO mice, the infarct volume was increased significantly. Also, astrocyte activation was reduced in PAR2 KO mice. Astrocytes are important cells as they provide structural, trophic and metabolic support to neurones and thereby regulate synaptic activity (Stoll et al. 1998). So, it is clear that PAR2 gene deficiency increases brain injury. The role of PAR2 in neurodegenerative disorders is uncertain; however, PAR2 exerted protective effects in neurones, but its activation in glia was pathogenic with secretion of neurotoxic factors and suppression of astrocytic anti-inflammatory mechanisms (Afkhami-Goli et al. 2007).
1.2.3 Gastrointestinal System
PARs have an important role in controlling gastrointestinal function since the digestive system produces, secretes and therefore is exposed to many different proteinases Vergnolle 2000. In addition to their digestive effects, gastrointestinal proteinases are involved in local tissue remodelling, blood coagulation, nutrient absorption and gut motility (Kawabata et al. 2001). During inflammation, infiltrating immune cells release serine proteinases such as thrombin, trypsin and mast cell tryptase which can subsequently cleave PARs. PAR2 agonists and trypsin present in the intestinal lumen stimulate the generation of inositol 1,4,5-triphosphate, arachidonic acid release and secretion of prostaglandin E2 (PGE2) and F1α from enterocytes (Kong et al. 1997). Since prostaglandins are known to regulate gastric secretion, intestinal transport and motility, this PAR2 pathway is important for the management of intestinal function (Eberhart and Dubois 1995). PGE2 is involved in the protection of cells in the upper intestine against digestion by pancreatic trypsin, and this is mediated by PARs in the epithelium (Kong et al. 1997). In a study by Cocks et al., it was reported that activation of PAR2, which co-localises with trypsin in airway epithelium, induces the relaxation of airway preparations by the release of PGE2, and it offers bronchoprotection. The bronchial epithelium resembles intestinal epithelium in terms of its morphology. So, it is possible that PAR2 may exert protective effect on the intestinal epithelium (Cocks et al. 1999a, b).
PAR2 present on enterocytes can be activated by trypsin, tryptase, while PAR2 effector cells like inflammatory cells, fibroblasts or neurones result in the secretion of eicosanoids (Kong et al. 1997). PAR1 and PAR2 activation can alter gastrointestinal motility since they are highly expressed by gastrointestinal smooth muscle cells (Corvera et al. 1997). When PAR1 and PAR2 were activated, they induced contractions in gastric smooth muscle (Saifeddine et al. 1996). Also, indomethacin blocked gastric contractions which indicates that the PAR1- and PAR2-induced contractions are prostaglandin mediated (Cocks et al. 1999b). In a study, the role for PARs in the modulation of motility of the rat oesophageal muscularis mucosae was observed (Kawabata et al. 2000). PAR1-activating peptides, but not the PAR1-inactive peptide, evoked a marked contraction in smooth muscle. However, PAR2 and PAR4 agonists caused negligible muscular contraction (Kawabata et al. 2000).
1.2.4 Musculoskeletal System
Individual PARs are expressed in a wide variety of musculoskeletal tissues such as bone, articular cartilage, menisci, synovium and muscle (Russell and McDougall 2009; McDougall and Linton 2012; Chinni et al. 2000). PAR1 and PAR2 are expressed in bone marrow stromal cells (Smith et al. 2004). Human osteoblasts express PAR1, 2 and 3, and mouse osteoblasts express PAR1, 2 and 4, whereas rat osteoblasts have been shown to express PAR1 and 2 only (Jenkins et al. 1993; Pagel et al. 2003; Bluteau et al. 2006). Activation of either PAR1 or PAR4 on osteoblasts using specific receptor-activating peptides causes a rapid mobilisation of intracellular calcium (Jenkins et al. 1993; Pagel et al. 2003). Thrombin is also involved in stimulating proliferation of bone marrow stromal cells in a PAR1-dependent manner (Song et al. 2005). Thrombin also decreases alkaline phosphatase activity in osteoblasts which is a marker of osteoblast differentiation (Abraham and Mackie 1999). Elsewhere, thrombin has been reported to be involved in stimulating bone resorption in an organ culture of neonatal mouse skull bones and foetal rat long bones (Gustafson and Lerner 1983). The resorption induced by thrombin can be inhibited by indomethacin (Gustafson and Lerner 1983; Hoffmann et al. 1986). This action could be related to thrombin stimulating the release of PGE2 and IL-6 by osteoblastic cells, and this effect is mediated by stimulation of osteoclast differentiation (Mackie et al. 2008). In chondrocytes which are obtained from osteoarthritis cartilage, the expression of PAR2 has been found to be higher when compared with normal cartilage (Xiang et al. 2006). PAR2 and PAR3 are expressed by cartilage in the embryonic mouse skeleton (Abraham et al. 1998). Elsewhere, it has been reported that thrombin triggers proliferation of chondrocytes isolated from human articular cartilage (Kirilak et al. 2006). Myoblasts express PAR1 and PAR2, whereas muscle fibres express PAR1, 2 and 4 (Chinni et al. 2000; Jenkins et al. 1993). When myoblasts were stimulated with thrombin or a PAR1-activating peptide, it resulted in mobilisation of calcium (Mackie et al. 2008). A study was carried out to explore the role of PAR2 activation in four different models of arthritis and in human arthritic synovium (Busso et al. 2007). In the adjuvant-induced arthritis model, arthritic symptoms were significantly decreased in PAR2-deficient mice and also in the presence of anti-mBSAIgG antibodies (Busso et al. 2007). No difference in arthritis severity was seen in mice with ZIA, K/BxN serum-induced arthritis or CFA-induced arthritis. Expression of PAR2 in rheumatoid arthritis synovium was significantly higher than in osteoarthritis synovium (Busso et al. 2007). In another study, when an antagonist of PAR2 (GB88) was tested for its efficacy, it attenuated PAR2 signalling, macrophage activation, mast cell degranulation and collagen-induced arthritis in rats (Lohman et al. 2012). McDougall et al. evaluated the role of PAR4 in synovial blood flow which is increased during inflammation. When kaolin/carrageenan was injected into the knees of the animals, they showed an increase in synovial blood flow. Treatment of these inflamed knees with the PAR4 antagonist pepducin P4pal-10 reduced the hyperaemia associated with acute synovitis (McDougall et al. 2009).
Persistent neck pain is a major cause of disability and the cervical facet joint is a common source of neck pain (Barnsley et al. 1995). Rats subjected to a painful joint distraction and receiving an injection of ketorolac either immediately or 1 day later showed an increase in spinal PAR1 and astrocytic PAR1 expression. The astrocytic PAR1 was returned to sham levels when ketorolac was administered on day 1 but not after the immediate administration. However, spinal PAR1 was significantly reduced by ketorolac independent of timing. This indicates that spinal astrocyte expression of PAR1 is involved in the maintenance of facet joint-mediated pain (Dong et al. 2013).
2 Proteinase-Activated Receptor: Role in Pain
2.1 PARs and Inflammatory Pain
Pain is a natural response to noxious environmental stimuli and warns the body of actual or impending damage. In addition to this physiologically appropriate acute pain response, long-lasting pain is maladaptive and serves no functional benefit to the organism. Chronic pain is typically a consequence of an underlying malady and is very difficult to treat across the lifespan. Inflammatory diseases, such as rheumatoid arthritis and Crohn’s disease, can be extremely painful and difficult to manage. During nociceptive pain, afferent nerve fibres are activated directly by a noxious environmental stimulus, and the resulting pain response lasts for only a relatively short period. During inflammation, however, the afferent fibres are continuously bombarded by inflammatory mediators culminating in protracted pain. This continuous peripheral drive leads to plasticity changes in the central nervous system resulting in chronic pain. While the list of inflammatory mediators and their distinct receptors involved in inflammatory pain is escalating, recent reports suggest an important role for PARs in pain signalling pathways.
2.1.1 PAR1
It has been shown that PAR1 is expressed on sensory neurones, but their role in nociceptive signalling remains under investigation. Asfaha et al. (2002) studied the effect of PAR1 activation on nociceptive response by thermal and mechanical stimuli. When thrombin was injected into the paws of rodents, it increased the nociceptive threshold and withdrawal latency indicative of an anti-nociceptive effect. While intraplantar injection of carrageenan produces a classical inflammatory pain response, co-administration of the compound with thrombin resulted in a reduction in mechanical and thermal hyperalgesia. Similarly in another study, when thrombin and the PAR1 agonist TFLLR-NH2 were injected intraplantarly, their effects significantly attenuated the hyperalgesia in rats treated with carrageenan (Kawabata et al. 2002). The mechanism by which PAR1 ameliorates inflammatory hyperalgesia was elucidated by Martin et al. (2009) who demonstrated that PAR1 agonism triggers the production of proenkephalin and the activation of opioid receptors.
2.1.2 PAR2
PAR2 is cleaved by trypsin, mast cell tryptase, chymase, neutrophil elastase but not by thrombin. Peptide agonists that trigger PAR2 can lead to acute inflammation, in part via a neurogenic mechanism (Steinhoff et al. 2000). It has been confirmed that PAR2 is expressed by primary afferent neurones, and PAR2 agonists trigger the peripheral release of inflammatory neuropeptides such as substance P and calcitonin gene-related peptide (Steinhoff et al. 2000). Intraplantar injection of sub-inflammatory doses of PAR2 agonists in rats and mice induced a prolonged thermal and mechanical hyperalgesia and elevated fos protein expression in the dorsal horn, indicating that peripheral PAR2 stimulation leads to increased electrochemical activity of spinal neurones (Vergnolle et al. 2001). Interestingly, this hyperalgesia was not present in mice lacking substance P-preferring NK-1 receptors or preprotachykinin-A or in rats treated with an intrathecal injection of a NK-1 antagonist. These observations further support the suggestion that substance P is involved in PAR2-mediated pain responses (Vergnolle et al. 2001).
In joints, PAR2 has emerged as a new therapeutic target for arthritis (Russell and McDougall 2009). PAR2 is expressed in several cell types where its cleavage by serine proteinases is involved in the pathogenesis of inflammatory arthritis by mechanisms that are as yet unclear (Russell et al. 2012). However, there have been a few studies attempting to explore neuronal and inflammatory changes in joints after PAR2 activation. Using the retrograde neuronal tracer Fluoro-Gold, Russell et al. (2012) identified the expression of PAR2 in rat knee joint L3–L5 DRG cells. Additionally, it was found that activation of PAR2 by the selective activating peptide 2-furoyl-LIGRLO-NH2 increased joint nociceptor fibre firing rate during normal and noxious rotation (Russell et al. 2012). Furthermore, intravital microscopy experiments showed significantly increased leukocyte rolling and adhesion in response to PAR2 stimulation (Russell et al. 2012). All these effects were blocked by pretreatment with a TRPV1 or NK-1 receptor-selective antagonist. In another study, it has been shown that intra-articular injections of the PAR2-activating peptide, SLIGRL-NH2, caused swelling, cytokine release and increased sensitivity to pain in the mouse knee joint (Helyes et al. 2010). The secondary mechanical allodynia and change in weight distribution induced by intra-articular SLIGRL-NH2 were also found to be TRPV1 dependent (Helyes et al. 2010). In other joints, PAR2 is expressed in the lining layer of the rat temporomandibular joint (TMJ) synovium, as well as in a high proportion of the trigeminal ganglion neurones that innervate this joint (Denadai-Souza et al. 2010). When PAR2 agonists were injected by the intra-articular route into the TMJ, they triggered a dose-dependent increase in plasma extravasation, neutrophil influx and induction of mechanical allodynia, and these effects were inhibited by a NK-1 receptor antagonist (Denadai-Souza et al. 2010).
There are a number of ion channels involved in modulating inflammatory pain, viz. TRPV1, TRPA1 and P2X3. Inflammatory mediators such as substance P and bradykinin potentiate currents through ATP receptor channels containing the P2X3 subunit (Paukert et al. 2001). PAR2-induced neurogenic inflammation causes an increase in P2X3 currents, evoked by α- and β-methylene ATP in DRG neurones (Wang et al. 2012). Thus, it has been proven that the functional interaction of the PAR2 and P2X3 in primary sensory neurones could contribute to the generation of inflammatory pain.
It has also been demonstrated that PAR2 activation induces visceral pain (Kawao et al. 2004). A study was undertaken to evaluate the effect of activation of PAR2 on colonic motility. PAR2 agonists administered intraluminally induced contractions of the colon and produced hypersensitivity to colorectal distension (Suckow et al. 2012). Lesioning of TRPV1 neurones by capsaicin treatment eliminated this enhancement in contraction which indicates that TRPV1/PAR2 expressing primary afferent neurones mediate an extrinsic motor reflex pathway in the colon (Suckow et al. 2012). In another study, PAR2 agonist administration induced sustained, concentration-dependent contraction of oesophageal longitudinal smooth muscle strips. Capsaicin desensitisation, substance P desensitisation or application of the selective neurokinin-2 (NK-2) receptor antagonist MEN 10376 blocked these contractions (Paterson et al. 2007). This pathway is similar to the pathway involved in acid-induced longitudinal smooth muscle contraction and oesophageal shortening (Paterson et al. 2007). It could be possible that acid-induced longitudinal smooth muscle contraction may involve mast cell-derived mediators that activate capsaicin-sensitive neurones via PAR-2 and hence modulation of PAR2 might be useful in treatment of oesophageal pain and hiatus hernia (Liu et al. 2010). PAR2 is also involved in mediating inflammatory pain in acute pancreatitis (Ceppa et al. 2011). Exogenous trypsin injected at a sub-inflammatory dose caused increased c-fos immunoreactivity, which is an indicator of spinal nociceptor activation. There were no signs of inflammation at this dose as indicated by serum amylase and myeloperoxidase levels. Trypsin IV and P23 injected at similar doses resulted in an increase of some inflammatory end points and caused a more robust effect on nociception; these effects were blocked by the trypsin inhibitor melagatran (Ceppa et al. 2011). Trypsin IV and rat P23 activate PAR2 and are resistant to pancreatic trypsin inhibitors, and hence they contribute to pancreatic inflammation and pain (Ceppa et al. 2011). Elsewhere, caerulein (an oligopeptide that stimulates smooth muscle and increases digestive secretions) administered at a single dose increased abdominal sensitivity to stimulation by von Frey hairs, without causing pancreatitis in PAR2 KO mice. Multiple administrations increased the severity of abdominal allodynia/hyperalgesia in PAR2 KO as compared to WT mice. When a PAR2-AP was co-administered with caerulein, it abolished hyperalgesia/allodynia in WT mice but not in PAR2 KO mice. These results clearly indicate that PAR2 attenuates pancreatitis-related hyperalgesia/allodynia without affecting the disease (Kawabata et al. 2006).
Phosphoinositide 3-kinases (PI3Ks) have also been implicated in dermal mechanosensitivity where touch was evaluated. PI3Kγ gene deletion increased scratching behaviours in histamine-dependent and PAR2-dependent itch, whereas PI3Kg-deficient mice were not able to enhance scratching in chloroquine-induced itch (Lee et al. 2011). Furthermore, deletion of the PI3Kγ gene does not affect behavioural licking responses to intraplantar injections of formalin or mechanical allodynia in a chronic inflammatory pain model (Lee et al. 2011). These findings suggest that PI3Kγ contributes to behavioural itching induced by histamine and PAR2 agonist but not a chloroquine agonist (Lee et al. 2011).
2.1.3 PAR3
There have been no specific studies conducted evaluating the role of PAR3 in inflammatory pain. In an investigation carried out by Zhu et al. (2005), however, it was observed that PAR3 mRNA was expressed in 41 % of rat DRGs. It was also observed that 84 % of PAR3 positive cells co-localised with CGRP suggesting that PAR3 could be involved in peripheral nociceptive mechanisms.
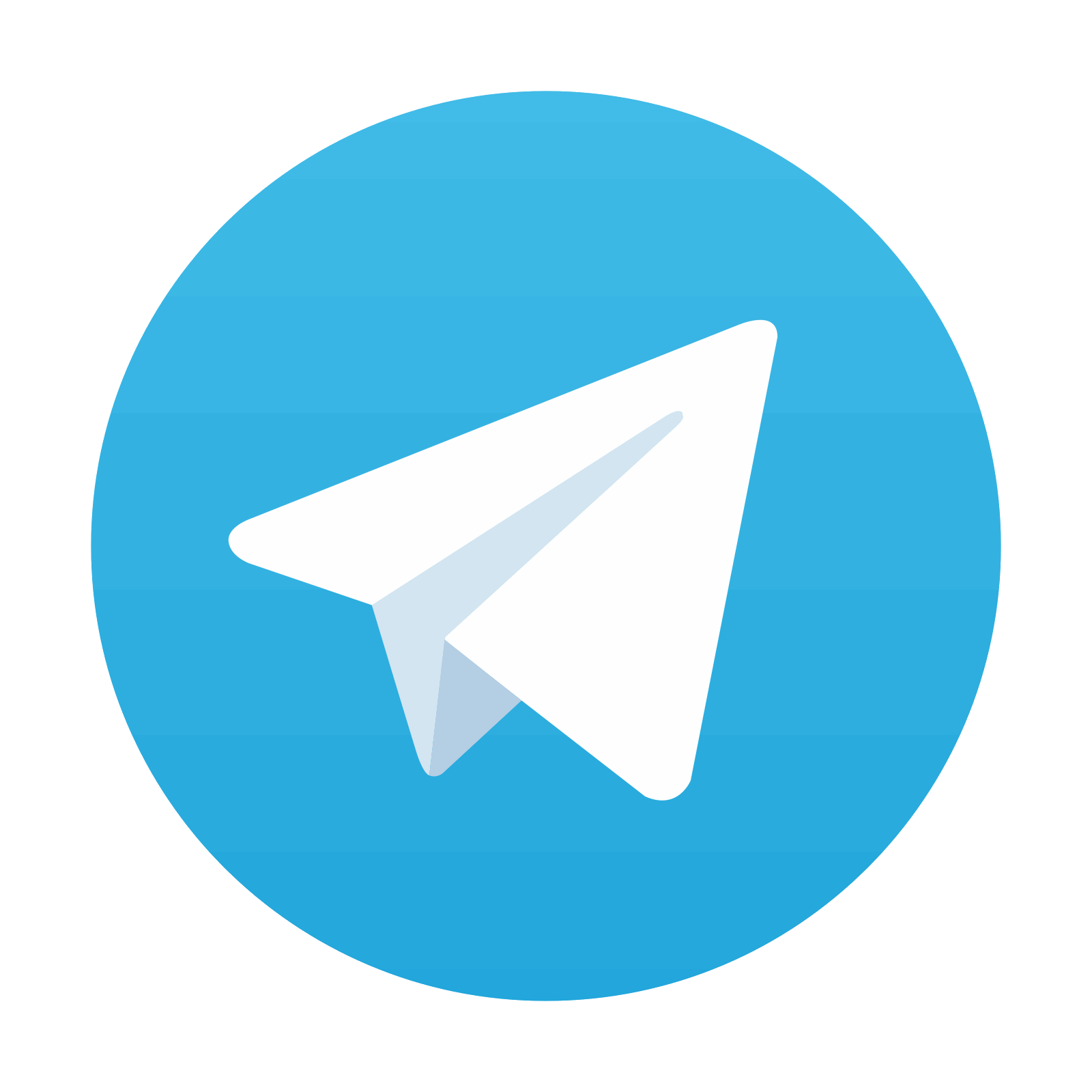
Stay updated, free articles. Join our Telegram channel

Full access? Get Clinical Tree
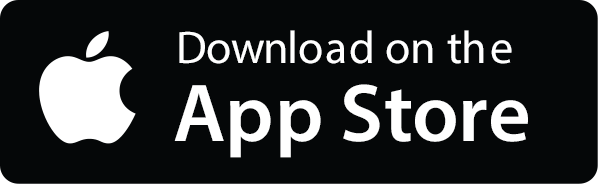
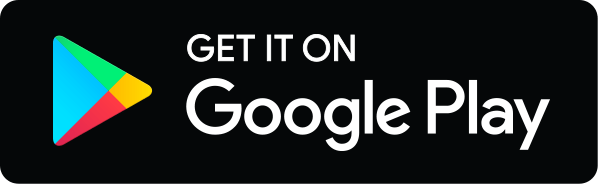