Fig. 1
Endocannabinoid signalling at a notional neuronal synapse. The major synthetic, signalling, and catabolic pathways for AEA and 2-AG are shown. Other GPCRs may be involved in endocannabinoid signalling alongside CB1 and CB2. FLAT-1, a truncated form of FAAH, and ECT are the putative EC transporters. The listed compounds are recognised enzyme inhibitors/receptor antagonists which can modulate EC signalling. It should be noted that the synthetic pathway responsible for AEA production in vivo has not been conclusively determined and that some evidence suggests AEA may act as an anterograde transmitter, being synthesised presynaptically and acting at postsynaptic TRPV1 receptors in some CNS regions (Aguiar et al. 2014). Figure adapted from Burston et al. (2013)
2 The Cannabinoid Receptors
The two known cannabinoid receptors have distinct expression patterns, which underlie their separate physiological roles. The CB1 receptor is found both in the periphery and central nervous system (CNS), although expression in the CNS is far greater. CB2, in contrast, is typically expressed predominantly by cells of the immune system, including glial cells of the CNS. However, more recent studies have demonstrated functional effects of CB2 receptor activity on neurones (Racz et al. 2008b; Burston et al. 2013).
The CB1 receptor is considered to be the most abundant GPCR in the CNS and is highly expressed in the neocortex, cerebellum, and limbic regions (Herkenham et al. 1990, 1991), but is also expressed in the peripheral nervous system (PNS). At peripheral and central terminals of nociceptive sensory nerves, CB1 gates the transduction of peripheral noxious stimuli into central neuronal pain signals (Guindon and Beaulieu 2006); at the level of the spinal cord, CB1 receptors can act to reduce or enhance propagation of pain signals to the brain (Sagar et al. 2010a, b; Pernia-Andrade et al. 2009; Woodhams et al. 2012), and those in nociception-associated higher brain regions, such as the periaqueductal grey matter (PAG) and the rostral ventromedial medulla (RVM), can initiate descending inhibition, or block descending facilitation, to the spinal cord nociceptive circuitry (Herkenham et al. 1991; Burns et al. 2007; Rea et al. 2007; Petrosino et al. 2007; Nadal et al. 2013; Martin et al. 1999). Interestingly, and of growing importance in pain research, CB1 is also highly expressed in the frontal–limbic brain circuits which are central to the affective/emotional aspects of pain in humans (Burns et al. 2007; Lee et al. 2013).
In line with its role in retrograde neurotransmission, CB1 is generally expressed presynaptically on axon terminals (Katona et al. 1999), where it is enriched in the perisynaptic zone (Nyíri et al. 2005), perfectly positioned to modulate the activity of the N- and P-/Q-type calcium channels which mediate neurotransmitter release. CB1 is negatively coupled to adenylate cyclase via Gi/o proteins, and thus its activation leads to inhibition of calcium channels, activation of potassium channels, and ultimately the reduction of neurotransmitter release (Pertwee 1997). At the circuit level, the net result of CB1 activity can be excitatory or inhibitory depending on the identity of the presynaptic cell and its location within the neural network. In addition to neuronal CB1, there is evidence of expression in B cells of the immune system (Graham et al. 2010; Pacher and Mechoulam 2011; Kaplan 2013) and also on glial cells of the CNS (Salio et al. 2002; Stella 2004, 2009; Navarrete and Araque 2008). Indeed, astroglial CB1 appears to have a pivotal role in both cannabinoid-mediated behaviour (Han et al. 2012) and EC-mediated plasticity (Min and Nevian 2012).
In contrast to the almost ubiquitous expression of CB1 in the nervous system, CB2 expression is thought to be confined to immune cells such as macrophages (Han et al. 2009), lymphocytes (Cencioni et al. 2010), and mast cells (Samson et al. 2003) in the periphery and astrocytes and microglia in the CNS (Alkaitis et al. 2010; Salio et al. 2002; Stella 2010). Activation of CB2 is inhibitory via Gi/o proteins, mediating the well-characterised anti-inflammatory effects of the ECs as well as having a role in anti-hyperalgesia in inflammatory pain states (Quartilho et al. 2003; Cabral and Griffin-Thomas 2009; Cabral et al. 2008; Khasabova et al. 2011; Ibrahim et al. 2006). There is a growing debate about the presence of functional CB2 receptors on some populations of neurons in the CNS (see commentary by Atwood and Mackie 2010), although at present this evidence remains controversial. Despite this restricted expression profile, CB2 plays an important role in pain signalling and may be of particular importance in the development of chronic pain states. This is discussed in more detail later in this chapter.
3 Endogenous Ligands: The Endocannabinoids
The ECs are lipid signalling molecules formed of two major groups: the N-acylethanolamines (NAEs) and the monoacylglycerols (MAGs). The most widely investigated are anandamide (AEA) and 2-arachidonoyl glycerol (2-AG), respectively (structures shown in Fig. 1). Although AEA was discovered first (Devane et al. 1992), 2-AG is now thought to be the major synaptic EC ligand in nervous tissue, acting as a full agonist at both CB1 and CB2 (Sugiura et al. 2000), and present at far higher levels than AEA in brain tissue (Stella et al. 1997). In contrast, AEA is a partial agonist at both cannabinoid receptors, with a slight selectivity for CB1 over CB2, but also acts as a full agonist at the ion channel receptor transient receptor potential vanilloid 1 (TRPV1) at higher concentrations (Zygmunt et al. 1999; Ross 2003). As such, AEA can be considered an endocannabinoid/endovanilloid substance and may have a dual role in nociception, being antinociceptive at cannabinoid receptors and pronociceptive at TRPV1 (see Starowicz and Przewlocka 2012 for review).
4 Endocannabinoid Synthesis and Degradation
The major ECs have distinct synthetic and degradative pathways, with the localisation of the enzymatic machinery for each ligand determining its physiological effects. Importantly, both classes of ECs are enzymatically synthesised de novo from membrane phospholipid precursors and are rapidly metabolised by specific enzymes, providing clear targets for the pharmaceutical modulation of EC signalling. A schematic diagram of the actions of ECs at a generic neuronal synapse can be seen in Fig. 1. Synthesis of ECs is initiated either via sustained increases in intracellular calcium following intense stimulation of the cell or through the activation of several classes of GPCRs (Hu et al. 2014). Of particular note is the group 1 metabotropic glutamate receptor mGluR5, which is often located in the perisynaptic zone (Lujan et al. 1996), and has an established role in nociception (Radulovic and Tronson 2012; Hu et al. 2012).
2-AG synthesis proceeds via the production of diacylglycerol (DAG) species from membrane phospholipids by PLCβ; these are then metabolised by the enzymes diacylglycerol lipase-α and β (DAGLα and β) to form 2-AG (Bisogno et al. 2003). Notably, DAG acts as a signalling molecule in its own right, activating protein kinase C (PKC) (Stella et al. 1997)—an enzyme with well-established pronociceptive effects in pain pathways (Velazquez et al. 2007). Synthesis of 2-AG therefore also terminates DAG signalling. Monoacylglycerol lipase (MAGL) is the major metabolic enzyme for 2-AG (Dinh et al. 2002), responsible for 85 % of brain 2-AG hydrolysis, with minor contributions from αβ hydrolase domains 6 and 12 (ABHD6 and ABHD12) (Blankman et al. 2007). ABHD12 is highly expressed in microglia, whilst ABHD6 is postsynaptic and may therefore regulate the release of 2-AG (Savinainen et al. 2011).
Originally, a shared synthetic pathway for AEA and its congener lipids palmitoylethanolamine (PEA) and oleoylethanolamine (OEA) via the enzyme N-acyl-phosphatidylethanolamine-hydrolyzing phospholipase D (NAPE-PLD) was described (Di Marzo et al. 1994; Okamoto et al. 2004). However, NAPE-PLD null mice have normal brain levels of AEA (Leung et al. 2006), and additional synthetic pathways have since been identified (Simon and Cravatt 2006, 2008), calling into question the predominance of this pathway in vivo. At present, the mechanism responsible for the production of AEA in pain pathways has not been conclusively determined. The NAEs PEA and OEA do not directly interact with cannabinoid receptors and are thus not considered to be true ECs. PEA, however, does have antinociceptive and anti-inflammatory properties via interactions with members of the peroxisome proliferator-activated receptor family (D’Agostino et al. 2009; Lo Verme et al. 2005). The metabolism of AEA occurs predominantly via the enzyme fatty acid amide hydrolase (FAAH), although other metabolic pathways have been identified (reviewed in Ueda et al. 2013), and these alterative pathways may have physiological relevance under certain conditions. Of particular relevance is the hydrolysis of both AEA and 2-AG by cyclooxygenase 2 (COX2) (Glaser and Kaczocha 2010), a key enzyme in pain processing, which can produce pronociceptive prostamide EC metabolites (Sang et al. 2006, 2007).
5 The Endocannabinoid System and Pain
Systemic administration of cannabinoid receptor ligands is well known to produce analgesia in animal models of acute and chronic pain (Walker and Huang 2002). Despite the recent increase in use of medicinal marijuana, and the development of licensed cannabinoid drugs such as Sativex for multiple sclerosis (Garcia-Merino 2013), concerns remain over dependence, tolerance, and the cognitive side effects produced by these medications. The undesirable effects of cannabinoids are caused by the global activation of CNS CB1 receptors, and as a result research has focussed on presumed site-specific modulation of endogenous ligand activity or on effects at the non-psychotropic CB2 receptor. In the next sections, we will describe the differing contributions of ECs to acute nociception and the development of pathophysiological pain states in each physiological compartment: at the periphery, in the spinal cord, and in supraspinal regions. Particular emphasis will be placed on two promising areas of EC research, namely, the modulation of 2-AG signalling and the novel role of spinal CB2 receptors in chronic pain states.
5.1 The EC System and Peripheral Pain Processing
In the periphery, CB1 receptors localised on sensory afferent terminals gate the transduction of pain signals from noxious stimuli (Stander et al. 2005). These receptors have a significant contribution to cannabinoid-mediated analgesia, since their selective deletion in mice greatly reduces the efficacy of both locally and systemically administered cannabinoids in models of acute and chronic pain (Agarwal et al. 2007). Numerous studies have demonstrated antinociceptive efficacy of local administrations of both AEA (Guindon et al. 2006; Guindon and Beaulieu 2006) and 2-AG (Desroches et al. 2008; Guindon et al. 2007), and elevated peripheral tissue levels of both ECs have been detected in numerous preclinical models of inflammatory pain (Beaulieu et al. 2000; Maione et al. 2007), suggesting endogenous activity of the EC system. These effects are not solely mediated via neuronal CB1, since blocking CB1 and/or CB2 receptors prior to formalin administration increases nociceptive responses (Guindon et al. 2007). In fact, the antinociceptive effect of peripherally administered 2-AG on inflammatory pain has been described as mainly CB2 mediated (Guindon et al. 2007). The mechanism is likely multifaceted, involving inhibition of the production and release of proinflammatory and pronociceptive mediators such as reactive oxygen species (Hao et al. 2010) and cytokines (Cencioni et al. 2010) by peripheral immune cells and also the peripheral release of endogenous opioids (Ibrahim et al. 2005; Desroches et al. 2014a).
Since ECs are rapidly degraded in vivo, their effects are short-lived, and they are thus unsuitable for use as analgesics. However, the recent development of specific inhibitors of the major catabolic enzymes (MAGL and FAAH) has enabled researchers to prolong the effect of endogenously generated ECs. This is a very promising analgesic strategy, since ECs are specifically generated at sites of nociceptive activity, and such an approach may avoid the unwanted effects of global CB1 receptor agonism. Systemic administration of either MAGL inhibitors (Long et al. 2009a; Ignatowska-Jankowska et al. 2014; Kinsey et al. 2009, 2013; Bisogno et al. 2009) or FAAH inhibitors (Jayamanne et al. 2006; Chang et al. 2006; Lichtman et al. 2004; Kathuria et al. 2003; Fegley et al. 2005; Russo et al. 2007) has been shown to be antinociceptive in models of acute and chronic pain. Local effects of MAGL and FAAH inhibition are best studied in rodent models of inflammatory pain, in which peripheral administration of an irritant produces oedema and pain behaviour. The MAGL inhibitor JZL184 (Long et al. 2009a) effectively blocks the pain behaviour and thermal hypersensitivity induced by intra-plantar capsaicin administration (Spradley et al. 2010). Intra-plantar JZL184 administration also inhibits local MAGL activity, increases 2-AG tissue levels, and blocks pain behaviour in the formalin model through mechanisms involving both CB1 and CB2 (Guindon et al. 2011). Peripheral FAAH inhibition via intra-plantar URB597 reduced carrageenan-induced hyperalgesia (Jhaveri et al. 2008) and also ameliorates an electrophysiological marker of central sensitisation in rat spinal cord (Sagar et al. 2008). Unlike the actions of 2-AG, peripheral effects of AEA may not involve CB2. URB937, a peripherally restricted FAAH inhibitor which cannot cross the blood–brain barrier, blocks the hypersensitivity induced by both inflammation and peripheral nerve injury via a CB1 receptor-mediated mechanism (Clapper et al. 2010). Enhancing peripheral EC signalling also has potential in the relief of chronic pain states, since inhibition of MAGL or FAAH is antinociceptive via CB1 and CB2 in a rodent model of neuropathic pain (Desroches et al. 2014b).
5.2 The Spinal Endocannabinoid System and Acute Pain Processing
The dorsal horn of the spinal cord is a key region in the pain processing pathway, receiving and encoding sensory input from the periphery and integrating the descending modulatory signals from higher brain regions. Intrathecal administration of cannabinoids is antinociceptive (Welch and Stevens 1992), whilst blocking spinal CB1 receptors produces hyperalgesia in mice (Richardson et al. 1997) and enhances nociception-evoked firing of wide dynamic range (WDR) neurones (Chapman 1999). These data suggest a powerful regulatory role of EC signalling at this level of the pain pathway.
Although the nociceptive circuitry of the dorsal horn has yet to be fully described (Todd 2010), the expression pattern of the EC system within some of its key components has been mapped. CB1 receptors are highly expressed presynaptically on the central terminals of nociceptive primary afferents and on populations of excitatory interneurons within the dorsal horn (Hegyi et al. 2009). At these synapses, the 2-AG signalling machinery has a complementary expression pattern, with DAGLα present postsynaptically (Nyilas et al. 2009) and MAGL localised within presynaptic terminals (Horvath et al. 2014). In contrast, the localisation of AEA-related machinery in the nociceptive circuitry remains unclear (Hegyi et al. 2012).
The simplest picture arising from these data is that spinal 2-AG signalling initiated by excessive nociceptive activity negatively modulates nociceptive signalling via inhibiting the release of pronociceptive neurotransmitters from primary afferent terminals. Indeed, under naïve conditions, spinal administration of the MAGL inhibitor JZL184 selectively inhibits acute mechanically evoked nociceptive neurotransmission in a CB1-receptor-mediated manner in rats (Woodhams et al. 2012), whilst FAAH inhibition is ineffective (unpublished data from our group). However, CB1 is also expressed on inhibitory interneurons and glial cells (Hegyi et al. 2009), and spinal levels of both AEA and 2-AG are elevated in animal models of acute and chronic pain (Sagar et al. 2010a, b, 2012). Spinal administration of AEA (Welch et al. 1995) or elevation of spinal AEA via the FAAH inhibitor URB597 (Jhaveri et al. 2006) is also antinociceptive, suggesting AEA signalling is also important. Furthermore, pronociceptive actions of CB1 agonism on inhibitory interneurons have been described following severe noxious stimulation (Pernia-Andrade et al. 2009), suggesting that the effects of EC signalling vary depending upon the physiological context.
During sustained noxious stimulation, spinal 2-AG and AEA signalling is temporally segregated. Assessing spinal levels of ECs in the surgical incision model of a resolving pain state in rats has revealed a time course of changes (Alkaitis et al. 2010). In the hours following a peripheral surgical incision, no changes in spinal 2-AG are observed, but there was a marked decrease in AEA levels corresponding to maximal mechanical hypersensitivity, which returns to baseline as nociceptive behaviour subsides. In contrast, 2-AG levels increase at later time points, corresponding with glial cell activation, upregulation of CB2 receptors, and resolution of the pain state. These data suggest that reduced AEA signalling may be involved in the onset of a pain state, whilst enhanced 2-AG signalling may be involved in pain resolution. Targeting AEA signals during the onset of pain behaviour may therefore not be an effective analgesic strategy, but it may instead be possible to block the onset of hypersensitivity by prolonging the immediate effects of 2-AG. A preliminary report indicates that spinal administration of JZL184 after carrageenan administration is able to block WDR receptive field expansion, a correlate of central sensitisation, in rats (Woodhams et al. 2012).
5.3 A Novel Role of Spinal CB2 Receptors in Chronic Pain States
In persistent pain states, such as neuropathic pain resulting from peripheral nerve damage, central sensitisation leads to a reorganisation of spinal nociceptive circuitry and the development of hypersensitivity. This manifests as both hyperalgesia (excessive pain following a nociceptive stimulus) and allodynia (perception of a normally innocuous stimulus as painful), for which current analgesics are largely ineffective. It is in such chronic pain states that EC research has the greatest potential. Spinal EC signalling is significantly enhanced in models of neuropathic pain, with elevations of both cannabinoid receptors (Lim et al. 2003; Zhang et al. 2003) and ECs reported (Sagar et al. 2012). Given the role of spinal 2-AG signalling at CB2 receptors in resolution of pain states highlighted above (Alkaitis et al. 2010), targeting CB2 in chronic pain states is a novel area of research with great promise.
A number of studies have reported upregulation of CB2 receptor mRNA and protein in the spinal cord in models of neuropathic pain (see references in Sagar et al. 2012), and spinal administration of CB2 agonists attenuates both neuronal (Sagar et al. 2005) and behavioural (Romero-Sandoval et al. 2008a; Yamamoto et al. 2008) nociceptive responses. Interestingly, and in contrast to both mixed CB1 and CB2 agonists and selective CB1 agonists, activation of spinal CB2 receptors was not effective in control rats. Similarly, CB2 agonism alters spinal nociceptive neuronal activity in a model of osteoarthritic pain, but has no effect in control animals (Burston et al. 2013). These data suggest a pain state-specific role for this receptor. Specificity of CB2 agonists has been demonstrated by their lack of effect in CB2 knockout mice in these models (Yamamoto et al. 2008). However, there is some conflicting evidence in the literature, with a recent study reporting no effect of the CB2 agonists GW405833 and JWH-133 on mechanical allodynia in the chronic constriction injury (CCI) model of neuropathy (Brownjohn and Ashton 2012). This study also reported no elevation of CB2 at either the protein or mRNA level, in contrast to numerous other studies.
The majority of preclinical studies of neuropathic pain utilise ligation or severance of peripheral nerves to induce a chronic pain state, but painful neuropathy is also associated with disease states such as diabetes, or as a result of chemotherapy treatments (Windebank and Grisold 2008). The few studies modelling these more clinically relevant conditions have, however, revealed evidence of a role for spinal CB2 receptors in attenuating pain behaviour. In a model of chemotherapy-induced neuropathy, spinal administration of the pan cannabinoid receptor agonist WIN55, 212-2 reduced mechanical allodynia via CB1 and CB2 receptors (Rahn et al. 2007). Likewise, in the rat STZ model of diabetes and diabetic neuropathy, CB1 receptor is downregulated in nociceptive primary afferent fibres (Zhang et al. 2007), but systemic administration of WIN55, 212-2 is still able to produce significant antinociceptive effects (Doğrul et al. 2004; Ulugol et al. 2004).
Targeting CB2 may also be effective in other chronic pain states, such as osteoarthritis. Mice over-expressing the CB2 receptor develop less severe pain behaviour following intra-articular injection of monosodium iodoacetate, whilst mice lacking CB2 receptors show enhanced pain responses on the contralateral side, indicating a role of CB2 receptors in this pain state (La Porta et al. 2013). Additionally, we have recently demonstrated that spinal CB2 expression is elevated in a rat model of osteoarthritic pain and that chronic systemic administration of the CB2 agonist JWH-133 can block pain behaviour, whilst acute spinal administration attenuates mechanically evoked nociceptive neurotransmission (Burston et al. 2013). Moreover, we found that spinal CB2 mRNA expression was negatively correlated with (macroscopic) knee chondropathy in end-stage OA patients, suggesting that disease progression may lead to a decrease in CB2 receptor expression and a facilitation of spinal hyperexcitability in human OA.
5.4 CB2 Receptor Modulation of Spinal Immune Cell Function
CB2 is primarily expressed on glial cells of the CNS, and these cells are therefore most likely to mediate the effects of CB2 agonists in models of persistent pain. Studies of cultured microglial cells have revealed that 2-AG signalling via CB2 receptors inhibits microglial migration (Walter et al. 2003) and that CB2 agonists reduce both the activation and migration of cultured primary microglia challenged with bacterial lipopolysaccharide (Romero-Sandoval et al. 2009). In a unilateral spinal nerve transsection model of neuropathic pain, CB2 upregulation on spinal microglia and perivascular cells has been reported (Romero-Sandoval et al. 2008b), and spinal administration of the CB2 receptor agonist JWH015 reduced both mechanical hypersensitivity and markers of microglia activation in this model. CB2 receptors are upregulated on both microglia and astrocytes following spared nerve injury in mice, and chronic systemic administration of the CB2 agonist NESS400 reduces pain behaviour, astrogliosis, microglial activation, and levels of proinflammatory cytokines, whilst promoting levels of anti-inflammatory cytokines (Luongo et al. 2010).
Genetic manipulations in mice have revealed the likely function of upregulated CB2 to be in attenuating the development of central sensitisation. Mice lacking CB2 receptors have enhanced pain responses to sciatic nerve ligation compared to their wild-type littermates, including the development of hypersensitivity in the contralateral side (Racz et al. 2008b). These behavioural effects are accompanied by large increases in spinal glial cell activation, which can be significantly attenuated by over-expression of CB2 receptors. Activation of CB2 receptors can reduce the release of proinflammatory cytokines from glial cells, and this appears to be important in the analgesic mechanism. Neuropathy induces a large IFN-γ response in CB2 knockout mice, whilst deletion of both IFN-γ and CB2 blocks the development of neuropathy-induced pain behaviour (Racz et al. 2008a).
It should be noted that the role of CB2 in persistent pain states may not be restricted to immune cells, as there is some evidence for neuronal CB2 expression in the spinal cords of neuropathic (Racz et al. 2008b) and osteoarthritic animals (Burston et al. 2013). However, at the present time, a lack of specific tools to map the expression of this receptor has hampered efforts to conclusively demonstrate neuronal expression.
5.5 Supraspinal Sites of Action of the Endocannabinoids
At the supraspinal level, the EC system can influence ascending pain signals in the thalamus, descending modulatory signals in the brainstem, and the affective/emotional aspects of pain sensation through actions in frontal–limbic circuits. In the rat, direct microinjection of cannabinoid agonists into the thalamus, PAG, dorsal raphe nucleus, and RVM all produce antinociceptive effects in acute pain tests (Martin et al. 1995, 1996, 1999), which can be blocked via antagonism of CB1 (Lichtman et al. 1996). The involvement of ECs at these sites has been revealed by the release of AEA following electrical stimulation of the PAG or after peripheral inflammatory insult (Walker et al. 1999). PAG levels of AEA and 2-AG are also elevated in animal models of neuropathic pain (Petrosino et al. 2007), highlighting this area as a key region in the pain matrix. Prolonging AEA action in the PAG via inhibition of FAAH is antinociceptive in acute pain tests, although a biphasic effect suggests higher AEA concentrations can result in pronociceptive via actions at TRPV1 (Maione et al. 2006). The mechanism of action of ECs in the RVM has been revealed through electrophysiological studies. In vivo recordings from the RVM in rats demonstrated that cannabinoids inhibit the firing of ON cells, whilst promoting the firing of OFF cells (Meng and Johansen 2004). As their names suggest, these two cell types have opposing effects, with ON cells facilitating and OFF cells inhibiting nociceptive activity.
The PAG has excitatory projections to the RVM, and the action of AEA in this region is thought to be via disinhibition of PAG neurons, leading to activation of OFF cells in the RVM, and induction of descending inhibitory GABAergic signalling to the spinal cord (Vaughan et al. 1999, 2000). EC signalling in the PAG also mediates stress-induced analgesia (SIA), the well-characterised reduction in nociceptive responses observed following the presentation of an external environmental stressor. In animal studies, mice or rats exposed to mild electrical foot shock show reduced pain behaviour in a subsequent test of acute nociception (Hohmann et al. 2005). This effect is mediated by CB1 and accompanied by the release of both AEA and 2-AG in the PAG. The effect size of SIA can be augmented through the use of either FAAH or MAGL inhibitors (Suplita et al. 2005, 2006), though it should be noted the MAGL inhibitor utilised in this study has low efficacy and selectivity (Vandevoorde et al. 2007). More recent evidence suggests that the involvement of 2-AG in the dorsolateral PAG is critical and suggests a mechanism involving the activation of descending inhibitory pathways (Gregg et al. 2012). The link between stress and pain in humans is far more complex than in animal models, but recent clinical studies suggest it is no less important (Vachon-Presseau et al. 2013). Since the EC system is also strongly linked to anxiety/anxiolysis (reviewed in Mechoulam and Parker 2013), it seems highly likely that EC signalling will be involved in this interaction. Indeed, a recent report has demonstrated that impaired endocannabinoid signalling in the RVM underpins hyperresponsivity to noxious stimuli in the Wistar Kyoto rat, a strain with a heightened stress/anxiety profile (Rea et al. 2014).
One of the major unresolved issues in pain research is how to match the objectively measurable neuronal and behavioural nociceptive output of nociceptive responses, with the subjective, integrative experience of pain. Since non-human animals cannot report their internal states, and invasive electrophysiological recordings cannot be performed in humans, it has long seemed that this was a gap which could not be bridged. However, the advent of imaging techniques such as functional magnetic resonance imaging (fMRI) has allowed researchers to begin to address this. Cannabinoids alter affective or emotional states in humans via effects on the frontal–limbic circuitry, and this likely contributes to their analgesic effects. Dissociating the affective component from the somatosensory is complex, but a recent neuroimaging study has attempted to do so. Subjects were asked to rate the intensity of mechanically evoked allodynic responses following cutaneous capsaicin treatment, in the presence and absence of systemically administered Δ9-THC, whilst brain activity was monitored via fMRI (Lee et al. 2013). The cannabinoid treatment had no significant effect on scores of pain intensity, but reportedly reduced the unpleasantness of the pain sensation. This effect was accompanied by reduced activity in the anterior cingulate cortex (ACC) and enhanced activity in the amygdala. The ability of EC-directed perturbations to mimic this affective component of cannabinoid analgesia has not yet been tested, but this study indicates that this will be an important question to answer. Some recent preclinical data mirror these fMRI findings and further highlight the ACC as an important area in the aversive component of pain (Lu et al. 2011; Chen et al. 2012). Astrocyte activity in the ACC correlates with the maintenance of pain aversion behaviour, and ablation of these astrocytes reverses the behavioural phenotype. Since astrocyte activity is regulated by cannabinoid receptors, it seems logical to assume that altering EC tone within the ACC could prove a useful strategy for treating the affective component of chronic pain states.
5.6 Enhancing EC Signalling: Problems of Plasticity
The antinociceptive efficacy of 2-AG elevation via MAGL inhibition in preclinical models is well established, presenting a very promising area for therapeutic research. Systemic administration of the MAGL inhibitor JZL184 (Long et al. 2009a) elevates levels of 2-AG in brain and peripheral tissues (Long et al. 2009b) and increases acute pain thresholds in mice. Robust antinociceptive effects have also been demonstrated in models of peripheral inflammatory pain (Ghosh et al. 2013), gastrointestinal pain (Busquets-Garcia et al. 2011; Kinsey et al. 2011), neuropathic pain (Kinsey et al. 2009, 2010), chemotherapy-induced neuropathy (Guindon et al. 2013), and bone cancer pain (Khasabova et al. 2011). Many of these conditions are refractory to standard analgesics, and thus targeting MAGL/2-AG has great potential. However, some recent evidence suggests that there may be a problem with using MAGL inhibitors. High doses of JZL184 induce some cannabinoid-like behaviours (Long et al. 2009a), suggesting that this approach may not entirely avoid the unwanted side effects of cannabinoids. Worse still, sustained global elevation of 2-AG via genetic deletion of MAGL or persistent blockade of MAGL activity with JZL184 produces functional antagonism of the brain EC system, resulting in profound downregulation and desensitisation of CB1 receptors in nociception-associated regions and a loss of analgesic phenotype (Chanda et al. 2010; Schlosburg et al. 2010). Chronic JZL184 administration may also result in physical dependence since repeated high dose JZL184 treatment produces behavioural symptoms of withdrawal when precipitated with a CB1 antagonist (Schlosburg et al. 2009).
However, these may not be insurmountable problems. Chronic partial inhibition of MAGL produces sustained analgesia in the absence of cannabinoid side effects in mice (Busquets-Garcia et al. 2011; Kinsey et al. 2011). Furthermore, a novel inhibitor, KML129, with a more attractive therapeutic profile has been developed (Ignatowska-Jankowska et al. 2014). This compound has greater selectivity for MAGL over FAAH than JZL184 and produces antinociceptive, but not cannabimimetic, effects in mouse models of acute and chronic pain. It remains to be seen whether this compound will also avoid functional antagonism of the EC system upon chronic administration.
FAAH inhibitors are also robustly antinociceptive in models of acute and chronic pain (Jayamanne et al. 2006; Chang et al. 2006; Lichtman et al. 2004; Kathuria et al. 2003; Fegley et al. 2005; Russo et al. 2007) and appear to lack the cannabimimetic effects associated with MAGL inhibition (Schlosburg et al. 2010; Busquets-Garcia et al. 2011). Although there is a wealth of preclinical data to support the use of EC-targeted compounds in clinical pain trials, the only major attempt to date was a failure. Pfizer investigated the ability of a highly selective FAAH inhibitor, PF-04457845, to produce analgesia in an osteoarthritic patient population (Huggins et al. 2012). The drug treatment was well tolerated and produced significant elevations in circulating AEA, but no analgesic effect was observed. Some preclinical evidence suggests a loss of antinociceptive activity with chronic administration of a FAAH inhibitor (Okine et al. 2012), but it is also possible that the major analgesic effect of cannabinoids in humans is dissociative, rather than sensory (Lee et al. 2013), an effect which has not been demonstrated with FAAH or MAGL inhibitors. This perhaps highlights a limitation of current clinical trials of analgesics, which focus on the sensory analgesic profiles of novel compounds without assessing effects on the affective/emotional aspects of pain. Based on this rather disappointing failure of PF-04457845, it remains to be seen whether the highly promising findings from animal studies can be translated into the clinic.
6 Summary
The EC system is a critical regulator of nociceptive function, active at all levels of the pain processing pathways. It is also a highly plastic system, with altered expression and function occurring with switches in physiological state. A wealth of preclinical data suggests that pharmacological modulation of EC function via the use of specific enzyme inhibitors is an efficacious analgesic approach and may be of particular importance in chronic, refractory pain states. However, clinical research in this area is still at an early stage, and some initial setbacks introduce a note of caution.
Acknowledgements
D.S. and J.J.B. are funded by Arthritis Research UK Pain Centre funding (grant no. 18769).
References
Adams IB, Martin BR (1996) Cannabis: pharmacology and toxicology in animals and humans. Addiction 91:1585–1614PubMed
Agarwal N, Pacher P, Tegeder I, Amaya F, Constantin CE, Brenner GJ, Rubino T, Michalski CW, Marsicano G, Monory K, Mackie K, Marian C, Batkai S, Parolaro D, Fischer MJ, Reeh P, Kunos G, Kress M, Lutz B, Woolf CJ, Kuner R (2007) Cannabinoids mediate analgesia largely via peripheral type 1 cannabinoid receptors in nociceptors. Nat Neurosci 10:870–879PubMedCentralPubMed
Aguiar DC, Moreira FA, Terzian AL, Fogaca MV, Lisboa SF, Wotjak CT, Guimaraes FS (2014) Modulation of defensive behavior by Transient Receptor Potential Vanilloid Type-1 (TRPV1) channels. Neurosci Biobehav Rev 46(Pt 3):418–428PubMed
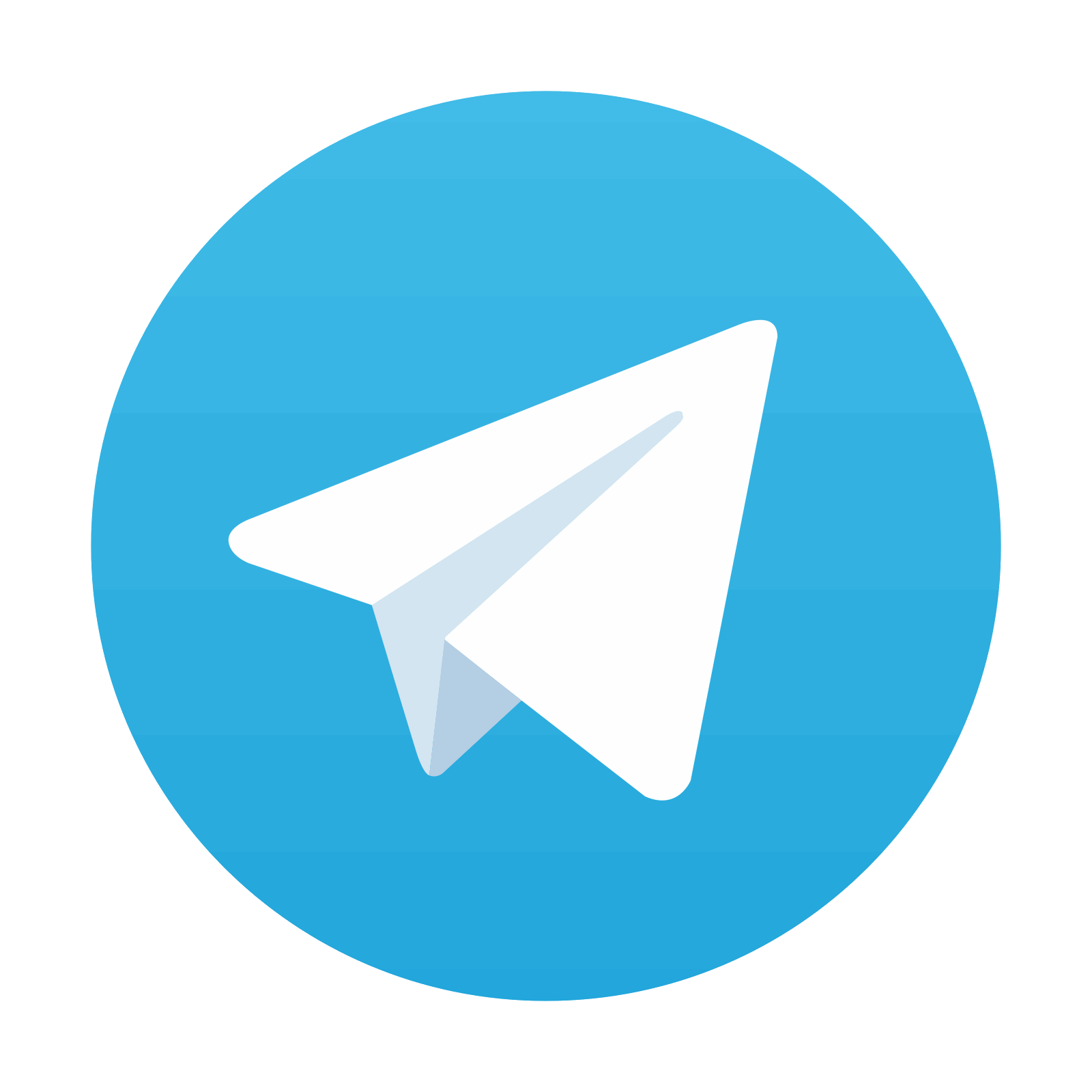
Stay updated, free articles. Join our Telegram channel

Full access? Get Clinical Tree
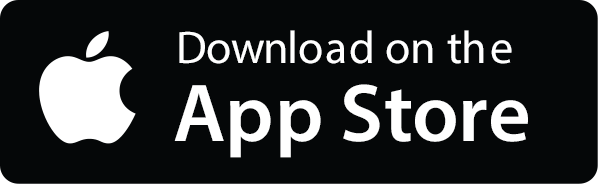
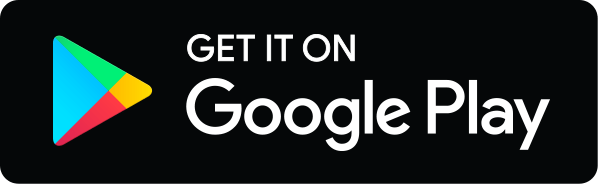