Chapter 19
Rational Use of Blood Products 
Basis for Transfusion of Blood Products: Benefits and Risks
Red Blood Cell Transfusion
The normal blood volume is 7% to 8% of predicted body weight (PBW). This corresponds to a total blood volume of ~70 mL/kg PBW (≈4.9 L for a 70 kg patient) with a hemoglobin volume of ~30 mL/kg and plasma volume of ~40 mL/kg. This corresponds to a normal hematocrit of 40% to 45% and a normal hemoglobin (Hgb) of 14 to 16 g/dL. Transfusion of red blood cells can help restore both circulating blood volume and oxygen carrying capacity as described by Equations 1 and 2 in Box 9.2, Chapter 9.
The body has many adaptive responses to increase oxygen delivery in the face of anemia (Box 19.1). Clinicians can increase O2 delivery by increasing the oxygen saturation or hemoglobin concentration or cardiac output. However, the latter results in increased myocardial oxygen consumption. Although this increases oxygen delivery acutely, a profound or prolonged increase in demand may precipitate ischemia in patients with underlying coronary artery disease.
General ICU Patients
Based on the findings of the TRICC trial, as a general rule, hemodynamically stable and asymptomatic patients in the ICU should not be transfused until their Hgb drops < 7 g/dL, at which point they should be transfused a single unit of PRBCs (Table 19.1). After this one unit is given, the patient’s Hgb should be rechecked to determine whether another transfusion is necessary to maintain the hemoglobin level at ≥ 7 g/dL.
TABLE 19.1
Suggested Packed Red Cell Transfusion Thresholds in Patients Who Are Not Actively Bleeding
Indication | Suggested transfusion threshold |
Hemodynamically stable patient | 7 g/dL∗ |
Patient with cardiovascular disease | 7–8 g/dL∗ |
Septic shock | 7–10 g/dL∗†‡ |
Acute coronary syndromes | |
Unstable angina, non-STEMI | 8–10 g/dL∗‡ |
STEMI | 10 g/dL∗ |
Traumatic brain injury | 7 g/dL∗ |
∗Transfuse only one-unit packed red cells at threshold, then recheck Hgb.
†Pending results for ProCESS study: http://clinicaltrials.gov/ct2/show/NCT00510835, accessed August 2, 2012.
Although the TRICC protocol’s Hgb threshold of < 7 g/dL for PRBC transfusion has been shown to benefit a population of patients, individuals may manifest varying degrees of tolerance to anemia. Should an anemic patient develop anginal pain, electrocardiographic (ECG) changes, or other signs/symptoms of inadequate oxygen delivery, the patient should be transfused, even if his or her Hgb is > 7 g/dL. Conversely, young and otherwise healthy patients may tolerate a Hgb < 7 g/dL. Though the safe and prudent lower limit for this patient population has not yet been defined, both the American Society of Anesthesiologists and the American Red Cross have published guidelines suggesting a lower limit of Hgb 6 g/dL in asymptomatic patients.
Stable Cardiovascular Disease
A 2012 guideline published by the American Association of Blood Banks (AABB) reflected these findings, recommending that asymptomatic patients be transfused at Hgb of 7 to 8 g/dL. Although general recommendations for transfusion triggers can be made (see Table 19.1), an appreciation of a particular patient’s physiology and symptoms, as well as an understanding of the inherent risks with transfusion, must be considered.
Acute Coronary Syndrome
For patients with acute coronary syndromes (ACS), there is very little clinical evidence to guide the transfusion threshold, as the TRICC trial excluded these patients and—despite the common presentation of ACS with anemia—a randomized, clinical trial has yet to be conducted. Myocardial oxygen delivery is dependent on coronary artery flow, and one of the physiologic compensations for anemia is coronary artery vasodilatation. Additionally, increased cardiac output (i.e., increased myocardial work) is another adaptation to anemia. For these reasons, the clinical rationale for transfusion in these patients is that an increase in oxygen carrying capacity should improve myocardial oxygenation in the background of acute coronary events.
Although a single study found that, in older adults, transfusion at a Hgb 10 g/dL was associated with improved survival, two other studies found that in patients with non-ST segment elevation myocardial infarctions (non-STEMI), a Hgb threshold of ~8 g/dL was associated with a trend toward improved outcomes. In all three of these trials, transfusion of patients who were not anemic was associated with increased mortality. Although the specific threshold remains to be determined, patients with ACS should be transfused to maintain a higher Hgb than patients with stable coronary artery disease (see Table 19.1). Until a randomized clinical trial provides better guidance, using a transfusion threshold of 8 to 10 g/dL seems reasonable.
Early Septic Shock
The care of patients with early septic shock was dramatically altered by the paradigm-shifting 2001 publication by Rivers and co-workers. This study found that a multifactorial intervention of hemodynamic goals, including volume resuscitation, vasopressor support, inotropic support, and blood transfusion, dramatically improved survival when administered to patients with severe septic shock within the first 6 hours of presentation. Because blood transfusion was one of several components administered (as a “sepsis bundle”), one cannot ascertain any independent effect that it may have had on outcome. Although many practitioners may transfuse hemodynamically unstable or acidemic patients to a Hgb of 10 mg/dL, the appropriate Hgb level for this patient population has not been determined prospectively. However, Angus and co-workers are conducting a multicenter, randomized controlled clinical trial, the Protocolized Care for Early Septic Shock (ProCESS) study (http://clinicaltrials.gov/ct2/show/NCT00510835, accessed August 2, 2012), which is addressing this important question and whose results should help to provide further guidance.
Beyond the early phase of septic shock, multiple, well-designed studies have failed to show that the transfusion of packed red cells can independently improve oxygen consumption or end-organ oxygen utilization in patients with early sepsis. Additionally, in late septic shock (> 24 hours after presentation), transfusion has not been shown to improve organ perfusion or oxygen consumption by multiple techniques, including gastric tonometry, sublingual microvascular studies, or indirect calorimetry. Because of the immunosuppression associated with transfusion and its strong association with the development of acute lung injury (ALI) and ARDS (with sepsis being the most common etiology of ALI/ARDS), the administration of packed red cells may be harmful. Some clinicians argue, pending the results of the ProCESS study noted previously, that a Hgb threshold of < 10 gm/dL for transfusing the septic patient should not be used (see Table 19.1).
Neurologic Injuries
In an observational study, anemia was associated with an increased risk of cerebral infarction and death in patients with subarachnoid hemorrhage (SAH). However, transfusing SAH patients does not appear to reduce mortality while, at the same time, increases the risk of acute lung injury. In TBI, transfusion has not been found to reduce in-hospital morbidity or mortality. Three studies have found that transfusing packed red cells increases cerebral oxygenation (PbtO2) in TBI; however, the significance of these findings is unclear. None of these studies used other volume expanders as a control, and no dose-dependent relationship was seen with the administration of multiple units of transfused blood. Moreover, approximately one quarter of the patients transfused actually had decreases in PbtO2 and, when measured, no effect on neurologic outcomes was observed. These findings can likely be explained by changes in the injured brain’s metabolism and circulation (e.g., patients with TBI have low cerebral oxygen extraction and demonstrate a loss of autoregulation). Likewise, vasospasm is one of the prominent pathophysiologic features of SAH. Moreover, brain edema may make oxygen delivery flow dependent rather than diffusion dependent. Thus, the importance and benefit of increasing cerebral oxygen delivery are unclear. For this reason, the American College of Critical Care Medicine Taskforce’s Clinical Practice Guidelines concluded that there is no convincing evidence for benefit in a liberal transfusion strategy (Hgb < 10 g/dL) in these patients. Thus, at this time, a restrictive transfusion strategy (Hgb < 7 g/dL) is recommended (see Table 19.1).
Although the majority of evidence suggests that best outcomes are obtained in the nonbleeding patient by minimizing transfusion, the opposite seems true in the setting of active hemorrhage. However, randomized clinical trials to support this conclusion are lacking. The pathophysiology of hemorrhage is more complex than simple hypovolemic shock, as it involves not only prior and ongoing blood loss but also an acquired coagulopathy and the loss of endothelial barrier integrity (Chapter 9). Current best evidence suggests that patients who are bleeding, particularly those with significant blood loss (≥ five units of PRBCs), benefit from more aggressive administration of blood products.
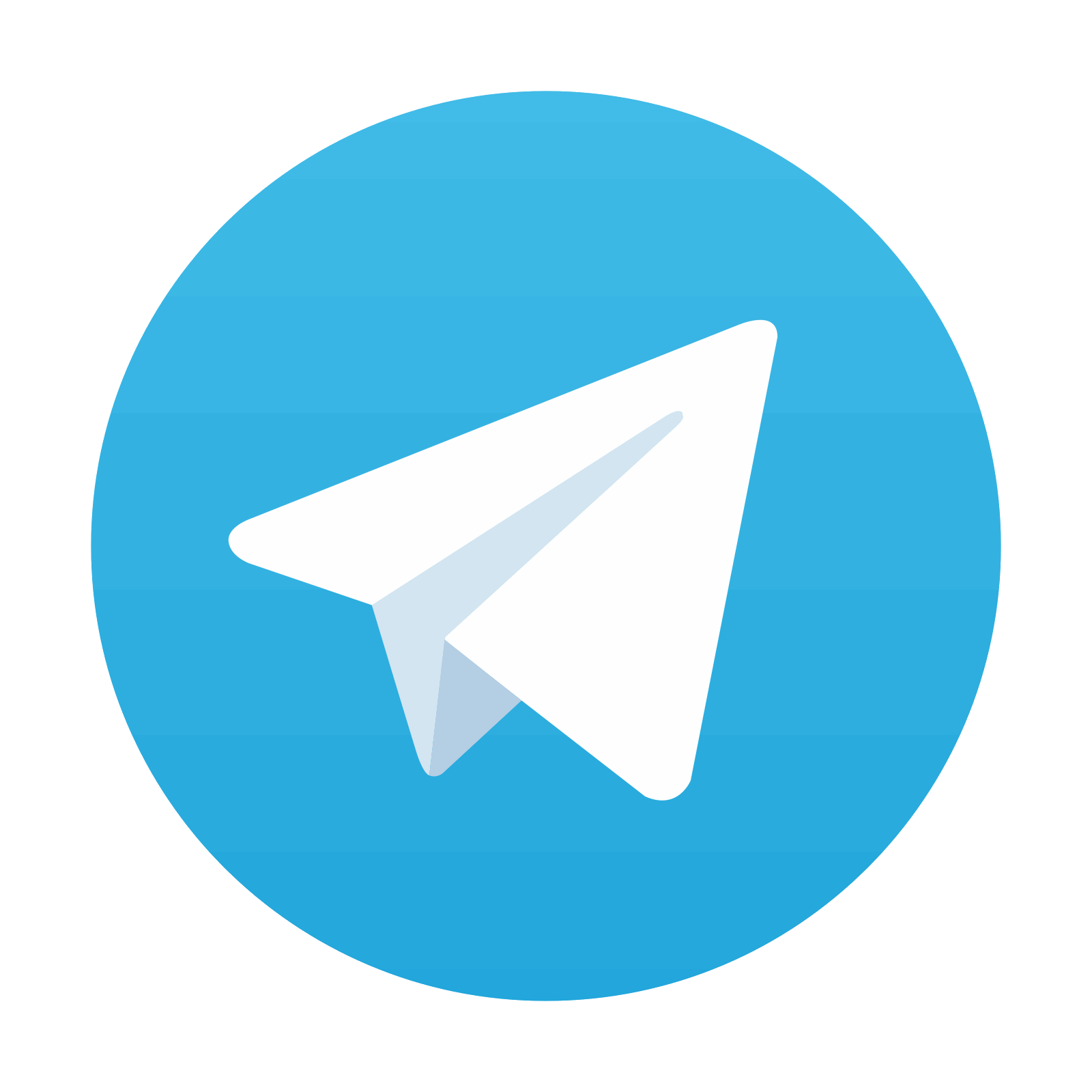
Stay updated, free articles. Join our Telegram channel

Full access? Get Clinical Tree
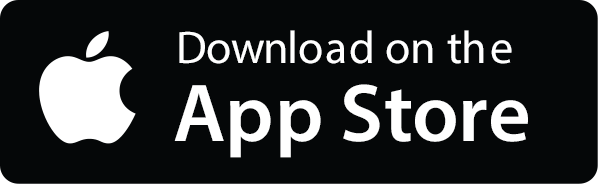
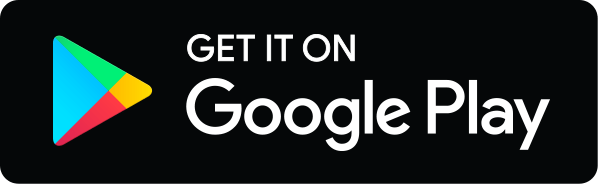