INTRODUCTION
Radiation exposure may either be accidental or intentional. The year 2015 marked the 70th anniversary of the bombing of Hiroshima and Nagasaki, with nearly 200,000 acute deaths and untold numbers with chronic disability. Accidental exposures can occur during transport, storage, or working with radioactive materials or with errors in dosing radiotherapy. Most civilian incidents involve industrial exposures from sealed radiation sources.1 The Fukushima Daiichi nuclear disaster resulted in about 1000 disaster-related deaths; however as of this writing, no deaths were related to radiation exposure.2
The largest reported accidental exposure took place in Goiania, Brazil in 1987. An “orphaned” cesium-137 radiosource was left in place at an abandoned radiotherapy institute. Individuals looking for scrap metal removed the source and dismantled it. They proceeded to sell it to a junk dealer, who observed the material glowing in the dark. Due to this unique characteristic, he distributed it to family and friends, who quickly became ill with acute radiation syndrome. At the conclusion of this event, there were 112,000 individuals evaluated for exposures, 249 who were contaminated, 20 who required hospital admissions, and four who died.3,4
A famous case of malicious intentional exposure involved Alexander Litvinenko, a former KGB agent who had defected to England. In 2006, after a meeting with former co-workers, he suffered a protracted gastrointestinal illness with associated leukopenia. On the day of his death, elevated levels of polonium-210 were identified, confirming his death from radiation exposure. Investigations into his murder revealed that there are had been rehearsals in multiple areas of England leading to contamination.5 The public health response that followed found that there were 1693 local and international individuals who were potentially exposed during such rehearsals.6,7
Radiologic dispersal devices, or “dirty bombs,” combine radioactive materials with conventional explosives in attempts to disperse “hot material” over an unsuspecting population. The intended use of these devices is to generate some injuries, but the true goal is to generate massive panic and hysteria, overwhelm local resources, affect the local economy, and lead to prolonged clean-up efforts.4
FUNDAMENTALS OF RADIATION PHYSICS
Radiation energy includes the entire electromagnetic spectrum: from low-energy, long-wavelength, and low-frequency nonionizing radiation, such as radio waves and microwaves, to high-energy, short-wavelength, and high-frequency forms of ionizing radiation. Ionizing radiation has enough energy to remove an electron from an atom and generate charged particles. Sources of ionizing radiation are: alpha particles, beta particles, neutrons, and sole energy waves that include x-rays and gamma rays.8
Alpha and beta particles and positrons are charged particles that directly interact with electrons of the atom. Neutrons are not charged, and they lead to expulsion of other particles after interactions with the atomic nuclei, so neutrons indirectly generate charged atoms. Gamma and x-rays are electromagnetic waves that destabilize the atomic nucleus and lead to the expulsion of ionized particles (Table 10-1).
Type (Symbol) | Charge | Penetration | Shield | Hazard | Source |
---|---|---|---|---|---|
Alpha | +2 | Few centimeters in air | Paper, keratin layer of skin | Internal contamination only; requires special detection devices | Heavy radioisotopes (e.g., plutonium, uranium, radon) |
Beta | –1 | ~8 mm into skin | Clothing | External (skin) and internal contamination | Most radioisotopes decay by beta followed by gamma emission |
Positron | +1 | ~8 mm into skin | Lead, steel or concrete | Interacts with electrons and releases photons of energy | Medical tracers |
Neutron | 0 | Variable | Material with high hydrogen content | Whole-body irradiation | Nuclear power plants, particle accelerators, weapons assembly plants |
Gamma and x-rays | 0 | Several centimeters in tissue | Concrete, lead | Whole-body irradiation | Most radioisotopes decay by beta followed by gamma emission |
Alpha particles have relatively large size (two protons and two neutrons), have limited travel potential, and are unable to penetrate the outer layers of the skin. Thus, alpha particles are easily shielded with a piece of paper. Exposure to alpha particles only leads to pathology in the setting of ingestion, inhalation, or absorption. Detection can be problematic because common Geiger counters do not detect alpha particles without a special attachment.8
Beta particles are much smaller (a single electron) than alpha particles. Small size allows for greater penetration ability. Beta radiation can travel several meters in air, penetrates approximately 8 mm into exposed skin, and can cause serious burns. Beta radiation is a hazard if internally deposited. Most radioisotopes decay by beta radiation followed by gamma emission.8
Positrons are positively charged beta particles that are emitted from the atomic nuclei. They are the antiparticles to the electron, and interactions with electrons lead to the generation of highly energetic photons that requires shielding with lead, steel, or concrete. Positron sources are commonly used in medical procedures such as positron emission tomography scanning.8
Neutrons are uncharged particles that are capable of generating radiation via alterations of the atomic nuclear proton-to-electron ratio. These particles are capable of traveling large distances and require the use of helium, water, and paraffin as shielding. Neutron exposures are rare and tend to be limited to nuclear fallout, research, industry, and weapons manufacturing.8
Gamma rays and high-energy x-rays are able to travel meters in the air and can penetrate centimeters into human tissue. Shielding materials must be very dense, such as concrete or lead. Individuals exposed to high doses of these sources are at high risk of developing acute radiation syndrome.8
BIOLOGIC EFFECTS OF IONIZING RADIATION
Ionizing radiation leads to cellular effects at both high and low levels of exposure. At high doses, ionizing radiation causes cell death. At lower doses, it interrupts cellular reproduction through inhibition of mitosis, resulting in cellular injury with delayed onset of effects.9
Radiosensitivity refers to the response of cells to radiation injury. Rapidly dividing cells with short life spans are the cells most vulnerable to radiation injury, because they are quickly depleted and new cells are unable to replete the population.4
There are many ways in which radiation can be measured: dose given, exposure received, absorbed dose, or activity generated. Many conventional units may be used, and confusion can arise between interchanging units. See Table 10-2 for more information on units of measure.
Description | Conventional Units | SI Unit | Conversion |
---|---|---|---|
Activity | Curie | Becquerel | 1 Bq ~2.7 × 1011 Ci |
Units of activity describe the amount of radioactivity present. | 1 Ci ~3.7 × 1010 Bq | ||
Exposure | Roentgen | Coulomb per kilogram | 1 R = 2.58 × 104 cP/kg |
Units of exposure measure the amount of x-ray or gamma radiation that produces a given number of ionizations in air. | |||
Absorbed dose | rad | Gray | 1 rad = 0.01 Gy |
Units of absorbed dose can be applied to any type of radiation and reflect the energy imparted to matter. | 1 Gy = 100 rad | ||
Dose equivalent | Roentgen equivalents man | Sievert | 1 rem = 0.01 Sv |
Units that provide a common scale of measure for the different types of radiation. | 1 Sv = 100 rem |
Just as there are many radiation units, there are many ways to monitor radiation exposures. Commonly used equipment includes dosimeters and survey meters (Table 10-3). In the setting of radiation emergencies, both of these devices should be available to ED staff. Staff should wear dosimeters because of their small size and ability to measure and record an individual’s cumulative exposure doses. In contrast, rate meters are survey instruments that record the amount of radiation in an area over a particular time course and are best suited to monitor environmental contamination.
Equipment Type | Device | Common Type of Measurement | Units Commonly Recorded |
---|---|---|---|
Dosimeter | Thermoluminescent dosimeter or film badge | Cumulative dose of beta, x-ray, and gamma | Roentgen equivalents man or sieverts |
Dosimeter | Pocket dosimeter | Cumulative exposure to x-ray and gamma | Milliroentgen |
Survey meter | Geiger-Müeller tube | Low exposure rates of x-ray, gamma, and beta* | Counts per minute† |
Survey meter | Ion chamber | Higher exposure rates of x-ray and gamma | Milliroentgen per hour |
Radiation exposures are an unavoidable hazard of living on our planet. Common sources of unavoidable radiation include cosmic and solar rays, naturally occurring elements such as radon and uranium, and even some of the carbon in our bodies. The background radiation dose of individuals living in the United States is approximately 6.2 mSv (620 mrem).10 The International Commission on Radiological Protection, the National Commission on Radiological Protection and Measurements, and the Health Physics Society have set the annual radiation dose limit for the general public at 1 mSv per year (100 mrem) over natural background radiation. See Table 10-4 for selected approximate levels of radiation exposure.
Natural background radiation | 620 mrem/y (U.S. average) |
Chest x-ray (effective dose) | 10 mrem |
Abdominal x-ray | 120 mrem |
Lumbar spine x-ray | 70 mrem |
CT head | 200 mrem |
CT chest | 700 mrem |
CT abdomen or pelvis | 1000 mrem |
Jet travel | 1 mrem per 1000 miles traveled |
Annual radiation dose limit (public) | 100 mrem/y* |
Occupational exposure limit | 5000 mrem/y |
Lethal dose in 50% of exposed subjects within 60 d (3.5–4.5 Gy) | 350,000–450,000 mrem (350–450 rad†) |
The LD50/60 from exposure to ionizing radiation is defined as the dose of penetrating ionizing radiation that will result in the deaths (lethal dose) of 50% of the exposed population within 60 days without medical treatment. Regarding human survival, the most commonly cited value is an LD50/60 of approximately 3.5 to 4.5 Gy (350 to 450 rad).8 In the setting of supportive medical therapy, including antibiotics, blood products, and reverse isolation, the value is 4.8 to 5.4 Gy (480 to 540 rad). During mass exposures where resources may be limited to basic first aid, the LD50/60 falls to approximately 3.4 Gy (340 rad). The use of stem cell transplantation and hematopoietic growth factor administration has theoretically increased the LD50/60 to 11 Gy (1100 rad).11
CLINICAL EFFECTS OF RADIATION
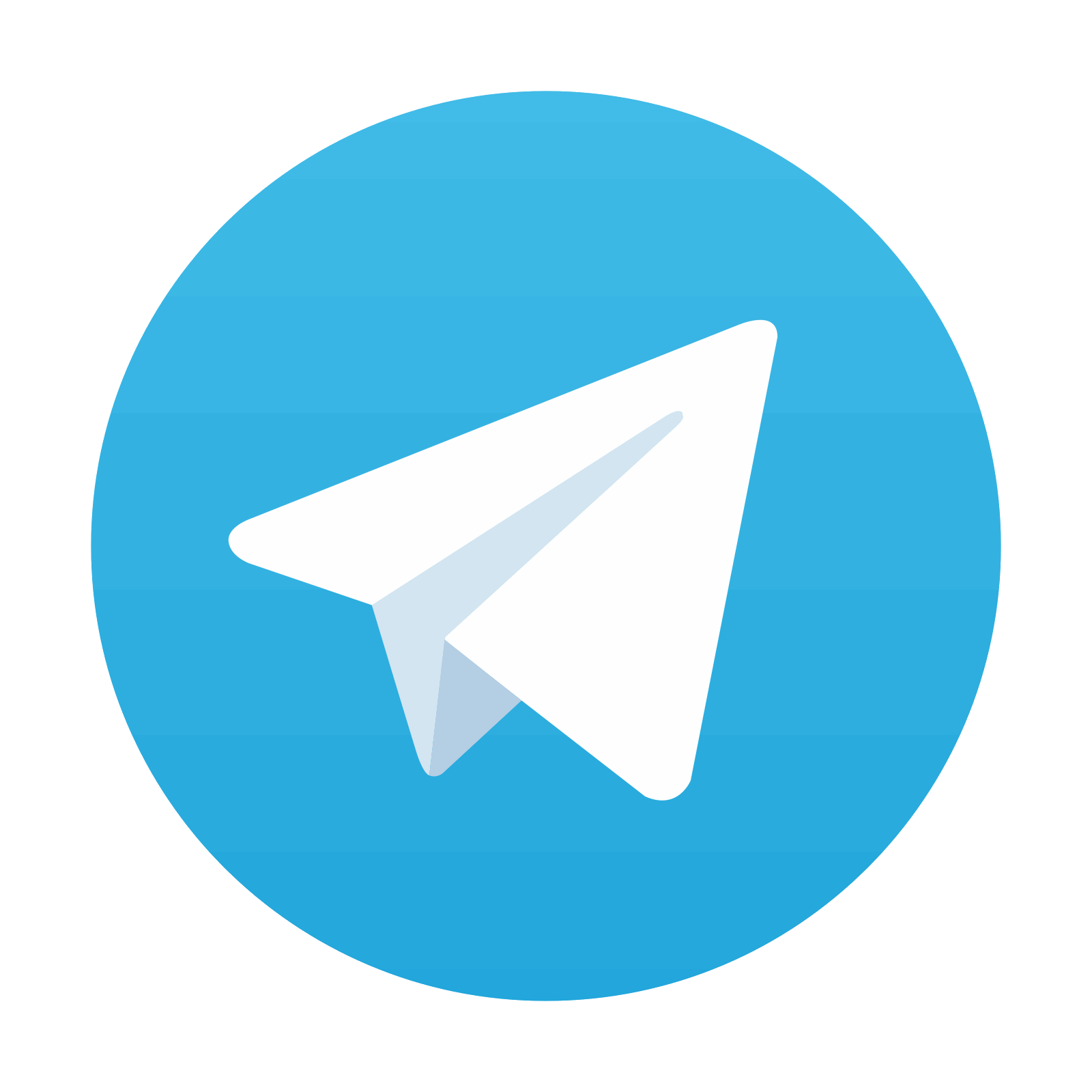
Stay updated, free articles. Join our Telegram channel

Full access? Get Clinical Tree
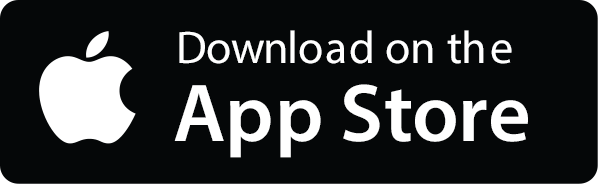
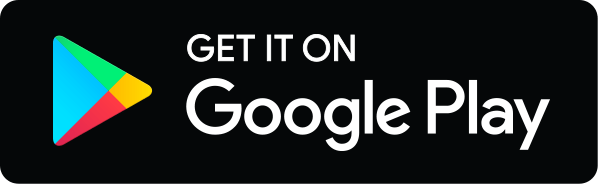