Post–Cardiac Arrest Syndrome and Management
Robert W. Neumar
Jerry Nolan
Robert W. Neumar and Jerry P. Nolan for the ILCOR Post-Cardiac Arrest Syndrome Writing Group
Robert W. Neumar, Co-chair, Jerry P. Nolan, Co-chair, Christophe Adrie, Mayuki Aibiki, Bemd W. Bottiger, Robert A. Berg, Clifton W. Callaway, Robert S. B. Clark, Romergryko G. Geocadin, Edward C. Jauch, Karl B. Kern, Ivan Laurent, W. T. Longstreth, Jr, Laurie J. Morrison, Peter Morley, Raina M. Merchant, Vinay Nadkarni, Mary Ann Peberdy, Antonio Rodriguez-Nunez, Emanual P. Rivers, Christian Spaulding, Frank W. Sellke, Kjetil Sunde, and Terry Vanden Hoek.
Mortality after ROSC is high. In published studies, average in-hospital mortality ranges from 50% to 90% after both out-of-hospital and in-hospital cardiac arrest.
PCAS represents a unique combination of pathophysiolgic processes that are potentially reversible.
Optimizing post–cardiac arrest care will improve survival with good neurologic outcome.
Optimized post–cardiac arrest care is a complex, time-sensitive endeavor that is best performed by a well-coordinated multidisciplinary team.

Background
Originally the D in the ABCDs of cardiopulmonary resuscitation stood for “definitive therapy.”1 Definitive therapy includes management of the pathologies that result from cardiac arrest as well as those that cause it. PCAS is a unique and complex combination of pathophysiologic processes, including (1) post–cardiac arrest brain injury, (2) post–cardiac arrest myocardial dysfunction, and (3) a systemic ischemia–reperfusion response.2 This state is often complicated by a fourth component: the unresolved pathology that caused the cardiac arrest. Extensive animal data have demonstrated that all components of PCAS are responsive to therapy. In humans, therapeutic hypothermia has provided the essential proof of concept that interventions initiated after ROSC can improve outcome.3,4 It is clear that optimized post–cardiac arrest management requires a comprehensive multidisciplinary plan that can be executed reliably 24 hours a day, 7 days a week. This approach has already been shown to improve outcome at individual institutions when compared with historical controls.5,6,7 In addition to an optimized therapeutic strategy, it is essential to develop a consistent, reliable, rational approach to limiting care in cases of futility and to consider the potential for organ donation.
Epidemiology of Post–Cardiac Arrest Syndrome
Early mortality of patients achieving ROSC after cardiac arrest varies dramatically between studies, countries, regions, and hospitals. The cause of these differences is multifactorial but includes variability in patient populations, reporting methods, and post–cardiac arrest care. In published studies, average in-hospital mortality ranges from 50% to 90% after both out-of-hospital and in-hospital cardiac arrest.8,9,10,11,12,13,14,15,16 Of the patients who survive to hospital discharge, approximately 60% to 80% have a good neurologic outcome.8,14,16 The reported incidence of clinical brain death in patients with sustained ROSC after cardiac arrest ranges from 8% to 16%.17,18 A number of studies have reported that transplant outcomes using organs obtained from appropriately selected post–cardiac arrest patients do not differ from those using organs obtained from other brain-death donors.18,19,20
Pathophysiology of Post– Cardiac Arrest Syndrome
The high mortality of patients who initially achieve ROSC after cardiac arrest can be attributed to a unique pathophysiologic process, involving multiple organs, which is often superimposed on the persistent, acute pathology that caused the cardiac arrest as well as underlying comorbidities. The four key components of PCAS are (1) post–cardiac arrest brain injury, (2) post–cardiac arrest myocardial dysfunction, (3) a systemic ischemia–reperfusion response, and (4) persistent precipitating pathology (Table 28-1).2 The severity of these disorders after ROSC will vary with the duration and cause of cardiac arrest, and they may be undetectable if cardiac arrest is brief.
The high mortality of patients who initially achieve ROSC after cardiac arrest can be attributed to a unique pathophysiologic process, involving multiple organs, which is often superimposed on the persistent, acute pathology that caused the cardiac arrest as well as underlying comorbidities.
Post–Cardiac Arrest Brain Injury
Post–cardiac arrest brain injury is a common cause of morbidity and mortality. In one study of patients surviving to ICU admission but subsequently dying in-hospital, brain damage was the cause of death in 68% after out-of hospital cardiac arrest and in 23% after in-hospital cardiac arrest.21 Clinical manifestations of post–cardiac arrest brain injury include coma, seizures, myoclonus, varying degrees of neurocognitive dysfunction (ranging from memory deficits to persistent vegetative state), and brain death (Table 28-1).22,23,24,25,26,27,28,29,30 The unique vulnerability of the brain is attributed to its limited tolerance of ischemia as well as its unique response to reperfusion. The mechanisms of brain damage triggered by cardiac arrest and resuscitation are complex, and many pathways are executed over hours to days following ROSC.31,32,33 The relatively protracted time course of injury cascades and histologic change suggests a broad therapeutic window for neuroprotective strategies following cardiac arrest.
Several postarrest factors can potentially exacerbate post–cardiac arrest brain injury. These include microcirculatory failure,34,35,36,37,38 impaired autoregulation,39,40,41,42,43,44,45,46 hyperoxia,47,48,49 hypercarbia, pyrexia,8,50,51 hyperglycemia,8,52,53,54,55,56,57 and seizures.30 Therapeutic strategies to limit these potential causes of secondary brain injury are discussed later in this chapter. There is limited evidence that brain edema or elevated intracranial pressure (ICP) directly exacerbates post–cardiac arrest brain injury. Although transient brain edema is observed early after ROSC, most commonly after asphyxial cardiac arrest, it is rarely associated with clinically relevant increases in ICP.58,59,60,61 In contrast, delayed brain edema, occurring days to weeks after cardiac arrest has been attributed to delayed hyperemia; this is more likely the consequence rather than the cause of severe ischemic neurodegeneration.59,60,61 There are no published prospective trials
that examine the value of ICP monitoring and management in post–cardiac arrest patients.
that examine the value of ICP monitoring and management in post–cardiac arrest patients.
Brain damage was the cause of death in 68% after out-of-hospital cardiac arrest and in 23% after in-hospital cardiac arrest.
Table 28-1 • Post–Cardiac Arrest Syndrome: Pathophysiology, Clinical Manifestations, and Potential Treatments | ||||||||||||||||||||||||
---|---|---|---|---|---|---|---|---|---|---|---|---|---|---|---|---|---|---|---|---|---|---|---|---|
|
Post–Cardiac Arrest Myocardial Dysfunction
Post–cardiac arrest myocardial dysfunction is a significant cause of morbidity and mortality after both in- and out-of-hospital cardiac arrest.21,62,63 In swine studies, ejection fraction decreases from 55% to 20% and left ventricular end-diastolic pressure increases from 8 to 10 to 20 to 22 mm Hg as early as 30 minutes after ROSC.64,65 In one series of 148 patients who underwent coronary angiography after cardiac arrest, 49% of subjects had myocardial dysfunction manifested by tachycardia and an elevated left ventricular end-diastolic pressure, followed approximately 6 hours later by hypotension (mean arterial pressure <75 mm Hg) and a low cardiac output (cardiac index <2.2 L/min/m2).63
Existing preclinical and clinical evidence indicates that this phenomenon is both responsive to therapy and reversible.63,64,65,66,67,68 In a swine model with no antecedent coronary or other features of left ventricular dysfunction, the time to recovery appeared to be somewhere between 24 and 48 hours.65 Several case series have described transient myocardial dysfunction after human cardiac arrest. In one study, cardiac index values reached their nadir at 8 hours postresuscitation, improved substantially by 24 hours, and were almost uniformly back to normal by 72 hours in patients surviving out-of-hospital cardiac arrest.63 More sustained depression of ejection fraction among in- and out-of-hospital post–cardiac arrest patients has been reported with continued recovery over weeks to months.67 The responsiveness of post–cardiac arrest global myocardial
dysfunction to inotropic drugs is well documented in animal studies.64,66
dysfunction to inotropic drugs is well documented in animal studies.64,66
Systemic Ischemia–Reperfusion Response
Cardiac arrest represents the most severe shock state during which delivery of oxygen and metabolic substrates is halted abruptly and metabolites are no longer removed. Cardiopulmonary resuscitation (CPR) reverses this process only partially, achieving cardiac output and systemic oxygen delivery (DO2) that is much less than normal. Inadequate tissue oxygen delivery can persist even following ROSC because of myocardial dysfunction, pressor-dependent hemodynamic instability, and microcirculatory failure. The whole-body ischemia–reperfusion of cardiac arrest with associated oxygen debt causes generalized activation of immunologic and coagulation pathways, thus increasing the risk of multiple organ failure and infection.69,70,71 This condition has many features in common with sepsis.72,73,74,75,76 In addition, activation of blood coagulation without adequate activation of endogenous fibrinolysis may also contribute to microcirculatory reperfusion disorders after cardiac arrest.77,78 Finally, the stress of total-body ischemia–reperfusion appears to adversely affect adrenal function.79,80 However, the relationship of adrenal dysfunction to outcome remains controversial.
Clinical manifestations of the systemic ischemia–reperfusion response include intravascular volume depletion, impaired vasoregulation, impaired oxygen delivery and utilization, and increased susceptibility to infection. In most cases these pathologies are both responsive to therapy and reversible. Data from clinical sepsis research suggest that outcomes are optimized when interventions are both goal-directed and initiated as early as possible.
Persistent Precipitating Pathology
PCAS is commonly associated with persisting acute pathology that caused or contributed to the cardiac arrest itself. The diagnosis and treatment of acute coronary syndrome, pulmonary diseases, hemorrhage, sepsis, and various toxidromes is often complicated in the setting of PCAS. However, early identification and effective therapeutic intervention is essential if optimal outcomes are to be achieved.
Therapeutic Strategies
Care of the post–cardiac arrest patient is time-sensitive, occurs in various locations both in and out of the hospital, and involves teams of health care providers from several disciplines. Ideally, a comprehensive clinical pathway tailored to available resources should be developed by relevant specialists.
Care of the post–cardiac arrest patient is time-sensitive, occurs in various locations both in and out of the hospital, and involves teams of health care providers from several disciplines. Ideally, a comprehensive clinical pathway tailored to available resources should be developed by relevant specialists. Treatment plans for post–cardiac arrest care must accommodate a spectrum of patients, ranging from the awake, hemodynamically stable survivor to the unstable comatose patient with persistent precipitating pathology. Such a plan enables physicians, nurses, and other health care professionals to optimize post–cardiac arrest care and prevent premature withdrawal of care before long-term prognosis can be established. This approach has been demonstrated to improve outcomes at individual institutions when compared with historical controls.5,6,81
Post–cardiac arrest patients generally require intensive care monitoring; this can be divided into three categories (Table 28-2): general intensive care monitoring, more advanced hemodynamic monitoring, and cerebral monitoring. General intensive care monitoring (Table 28-2) is the
minimal requirement; additional monitoring should be added depending on the status of the patient and local experience. The impact of specific monitoring techniques on outcome after cardiac arrest has not been studied prospectively.
minimal requirement; additional monitoring should be added depending on the status of the patient and local experience. The impact of specific monitoring techniques on outcome after cardiac arrest has not been studied prospectively.
Table 28-2 • Post–Cardiac Arrest Syndrome: Monitoring Options | ||
---|---|---|
|
Diagnosis and Treatment of Acute Coronary Syndromes
The majority of out-of-hospital cardiac arrest patients have coronary artery disease (CAD),82,83,84 and an acute coronary syndrome (ACS) is the most common cause of sudden cardiac death in the adult.84 Acute changes in coronary plaque morphology occur in 40% to 86% of cardiac arrest survivors and are found in 15% to 64% of autopsy studies.85 Thus, early post–cardiac arrest coronary angiography with subsequent percutaneous coronary intervention (PCI) is appropriate not just for those patients with ST-segment elevation myocardial infarction (STEMI) but also for those who are suspected of having an ACS.6,86,87,88,89,90,91 Several studies have evaluated the use of primary PCI for patients with ST elevation on their electrocardiograms (ECGs) following resuscitation from cardiac arrest, and the reported in-hospital mortality rates range from 20% to 45%.89,90,92 These studies have included many patients who remain comatose after initial resuscitation and, when appropriate (see below), the combination of mild hypothermia and primary PCI resulted in better outcomes than PCI alone.6,7,93 Chest pain and/or ST-segment elevation may be poor predictors of acute coronary occlusion in post–cardiac arrest patients;86 prospective studies are needed to determine whether immediate coronary angiography should be performed on all patients who experience ROSC after out-of-hospital cardiac arrest.
If there are no facilities for immediate PCI, in-hospital thrombolysis is recommended for patients with ST-segment elevation who have not received prehospital thrombolysis.94,95 Coronary artery bypass grafting is indicated in the postresuscitation phase for patients with left main stenosis or triple-vessel coronary artery disease if the cardiac arrest was thought to be caused by ischemic heart disease. In addition to acute reperfusion, management of ACS and CAD should follow standard guidelines (see Chapters 5 and 7).
Early Hemodynamic Optimization
Early hemodynamic optimization or early goal-directed therapy (EGDT) is an algorithmic approach to restoring and maintaining the balance between systemic oxygen delivery and demand. Monitoring and therapy are initiated as early as possible with the aim of achieving goals within hours of presentation. This involves optimizing preload, arterial oxygen content, afterload, contractility, and systemic oxygen utilization. EGDT has been studied in randomized prospective clinical trials of severe sepsis and in postoperative patients.96,97,98 Goals in these studies have included a central venous pressure (CVP) of 8 to 12 mm Hg, mean arterial pressure (MAP) of 65 to 90 mm Hg, central venous oxygen saturation (ScvO2) >70%, hematocrit >30% or Hb >8 g dL-1, lactate ≤2 mmol/L, urine output ≥0.5 mL/kg/hr, and oxygen delivery index >600 mL/min/m2. The goals are achieved with the use of intravenous fluids, inotropes, vasopressors, and blood transfusion as required. The benefits of EGDT include modulation of inflammation, reduction of organ dysfunction, and reduction of health care resource consumption.96,97,98 In severe sepsis, EGDT has also been shown to reduce mortality.96
Early hemodynamic optimization or early goal-directed therapy (EGDT) is an algorithmic approach to restoring and maintaining the balance between systemic oxygen delivery and demand. Monitoring and therapy are initiated as early as possible with the aim of achieving goals within hours of presentation.
The systemic ischemia–reperfusion response and myocardial dysfunction of PCAS have many characteristics in common with sepsis.72 Therefore, it has been hypothesized that early hemodynamic optimization might improve the outcome of post–cardiac arrest patients. However, the benefit of this approach has not been studied in randomized prospective clinical trials. Moreover, the optimal goals and strategies to achieve those goals could be different in PCAS, given the concomitant presence of post–cardiac arrest brain injury, myocardial dysfunction, and persistent precipitating pathologies.
Hemodynamic instability is common after cardiac arrest and manifests as dysrhythmias, hypotension, and a low cardiac index.63 Underlying mechanisms include intravascular volume depletion, impaired vasoregulation, and myocardial dysfunction. Dysrhythmias are treated by maintaining normal electrolyte concentrations and using standard drug and electrical therapies. There is no evidence to support the prophylactic use of antiarrhythmic drugs after cardiac arrest. Dysrhythmias are commonly caused by focal cardiac ischemia, and early reperfusion treatment is probably the best antiarrhythmic therapy. Ultimately, survivors whose cardiac arrest is attributed to a primary dysrhythmia should be evaluated for pacemaker or an implantable or internal cardioverter defibrillator (ICD) placement.
The first-line intervention for hypotension is to optimize right heart filling pressures using intravenous fluid. In one study, 3.5 to 6.5 L intravenous crystalloid was required in the first 24 hours after out-of-hospital cardiac arrest to maintain right atrial pressures in the range of 8 to 13 mm Hg.63 In one study, out-of-hospital post–cardiac arrest patients had a positive fluid balance of 3.5 ± 1.6 L in the first 24 hours, with a CVP goal of 8 to 12 mm Hg.6 The appropriate CVP for individual patients is highly variable; for example, those with right heart dysfunction or pulmonary embolism will require a much higher CVP than a patient whose primary problem is severe left ventricular dysfunction.
The optimal MAP for post–cardiac arrest patients has not been defined by prospective clinical trials. These
patients require an adequate MAP to perfuse the postischemic brain but without subjecting the postischemic heart to excessive afterload. The loss of cerebrovascular pressure autoregulation makes cerebral perfusion dependent on cerebral perfusion pressure (CPP = MAP – ICP). Since sustained elevation of the ICP during the early post–cardiac arrest phase is uncommon, cerebral perfusion is predominantly dependent on MAP. If fixed or dynamic cerebral microvascular dysfunction is present, an elevated MAP could theoretically increase cerebral oxygen delivery. In one human study, the MAP during the first 2 hours after ROSC correlated positively with neurologic outcome.99 Good outcomes have been achieved in published studies where the MAP target was as low as 65 to 75 mm Hg27 to as high as 90 to 100 mm Hg4,5 for patients admitted after out-of-hospital cardiac arrest. The optimal MAP in the post–cardiac arrest period might be dependent on the duration of cardiac arrest, with higher pressures needed to overcome the potential no-reflow phenomenon observed with >15 minutes of untreated cardiac arrest.34,35,100 At the opposite end of the spectrum, a patient with an evolving acute myocardial infarction (AMI) or severe myocardial dysfunction might benefit from the lowest target MAP that will ensure adequate cerebral oxygen delivery.
patients require an adequate MAP to perfuse the postischemic brain but without subjecting the postischemic heart to excessive afterload. The loss of cerebrovascular pressure autoregulation makes cerebral perfusion dependent on cerebral perfusion pressure (CPP = MAP – ICP). Since sustained elevation of the ICP during the early post–cardiac arrest phase is uncommon, cerebral perfusion is predominantly dependent on MAP. If fixed or dynamic cerebral microvascular dysfunction is present, an elevated MAP could theoretically increase cerebral oxygen delivery. In one human study, the MAP during the first 2 hours after ROSC correlated positively with neurologic outcome.99 Good outcomes have been achieved in published studies where the MAP target was as low as 65 to 75 mm Hg27 to as high as 90 to 100 mm Hg4,5 for patients admitted after out-of-hospital cardiac arrest. The optimal MAP in the post–cardiac arrest period might be dependent on the duration of cardiac arrest, with higher pressures needed to overcome the potential no-reflow phenomenon observed with >15 minutes of untreated cardiac arrest.34,35,100 At the opposite end of the spectrum, a patient with an evolving acute myocardial infarction (AMI) or severe myocardial dysfunction might benefit from the lowest target MAP that will ensure adequate cerebral oxygen delivery.
Inotropes and vasopressors should be considered if hemodynamic goals are not achieved despite optimized preload. Post–cardiac arrest global myocardial dysfunction is common;63,67,72 although it is generally reversible and responsive to inotropes, the severity and duration of the myocardial dysfunction will affect survival.63 Early echocardiography will enable the extent of myocardial dysfunction to be quantified and may guide therapy. Impaired vasoregulation is also common in post–cardiac arrest patients; this may require treatment with vasopressors and is also reversible. Persistence of reversible vasopressor dependency has been reported for up to 72 hours after out-of-hospital cardiac arrest despite preload optimization and reversal of global myocardial dysfunction.63 No individual drug or combination of drugs has been demonstrated to be superior in the treatment of post–cardiac cardiovascular dysfunction. The choice of inotrope and/or vasopressor can be guided by blood pressure, heart rate, echocardiographic estimates of myocardial dysfunction, and surrogate measures of tissue oxygen delivery such as ScvO2, lactate clearance, and urine output. If a pulmonary artery catheter (PAC) or some form of noninvasive cardiac output monitor is being used, therapy can be further guided by cardiac index and systemic vascular resistance.
If volume expansion and treatment with vasoactive and inotropic drugs do not restore adequate organ perfusion, consider mechanical circulatory assistance.101,102 This treatment can support the circulation for the period of transient severe myocardial dysfunction that often occurs after ROSC.63 The intra-aortic balloon pump (IABP) is the most readily available device to augment myocardial perfusion; it is generally easy to insert with or without radiologic imaging, and its use after cardiac arrest is well documented.6,93
Monitoring of the balance between systemic oxygen delivery and consumption can be accomplished indirectly with SvO2 (mixed venous oxygen saturation) or ScvO2 (central venous oxygen saturation). However, use of PAC—required for the measurement of SvO2—is diminishing, the optimal ScvO2 goal for post–cardiac arrest patients has not been defined, and the value of continuous ScvO2 monitoring is uncertain.
Urine output and lactate clearance are surrogates for oxygen delivery. Although two EGDT trials used a urine output target of ≥0.5 mL/kg/hr,96,98 a higher urine output goal of >1 mL/kg/hr is reasonable in post–arrest patients treated with therapeutic hypothermia, because this intervention induces diuresis.6 A limitation is that urine output can be misleading in the presence of acute or chronic renal insufficiency. Lactate concentrations are elevated early after ROSC because of the total-body ischemia caused by cardiac arrest. This limits the utility of a single measurement during early hemodynamic optimization. Lactate clearance is associated with outcome after out-of-hospital cardiac arrest;103,104 however, lactate clearance can be impaired by hepatic insufficiency and hypothermia. The optimal goal for hemoglobin concentration in the post–cardiac arrest phase has not been defined.6
In summary, based on the limited available evidence, reasonable goals for post–cardiac arrest syndrome include a MAP of 65 to 100 mm Hg (taking into consideration the patient’s normal blood pressure, the cause of the arrest, and the severity of any myocardial dysfunction), ScvO2 >70%, urine output >1 mL/kg/hr, and a normal or decreasing serum lactate concentration. Goals for hemoglobin concentration during postresuscitation care remain to be defined.
Controlled Reoxygenation
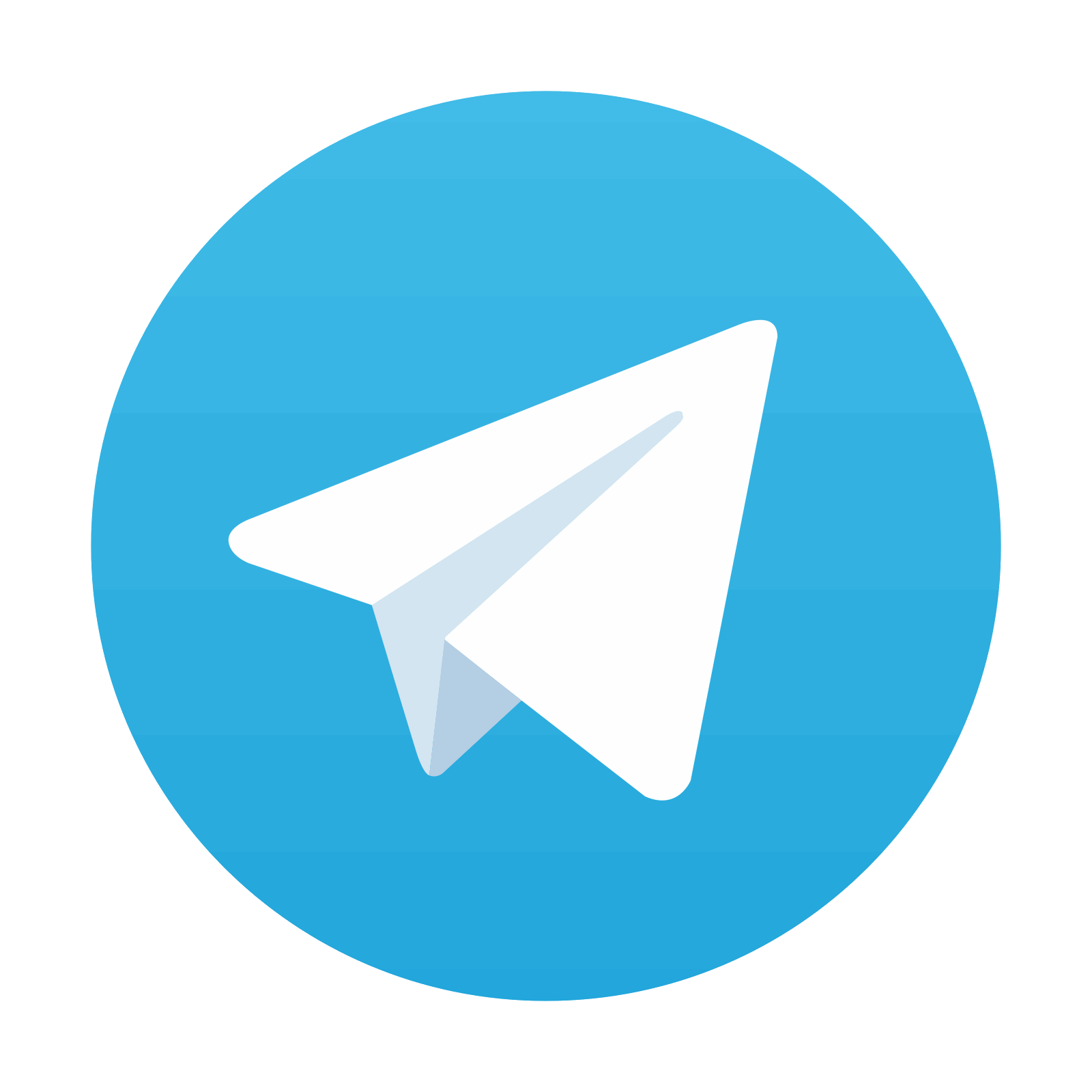
Stay updated, free articles. Join our Telegram channel

Full access? Get Clinical Tree
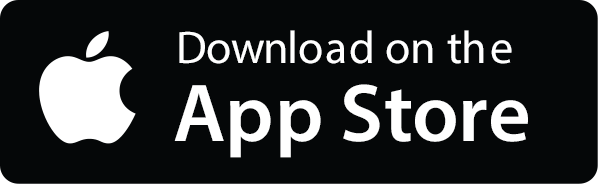
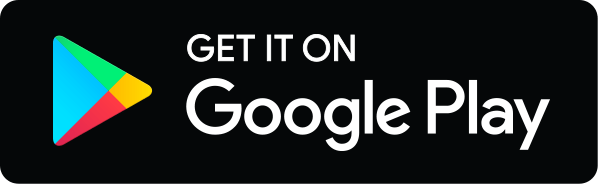