B. The synthesis of heroin in 1874 was based on the empirical finding that boiling morphine with specific acids caused the replacement of the two morphine —OH groups by —OCOH3 producing diamorphine or heroin (Fig. 19-1).
C. In 1937, meperidine (pethidine) became the first synthetic opioid synthesized based on the central structure of morphine. Since then, many synthetic and semisynthetic opioids have been produced, including the clinically important opioid antagonists naloxone and naltrexone, by replacing the N-methyl substituent in morphine with allyl and cyclopropylmethyl groups, respectively (see Fig. 19-1).
D. For clinical use during anesthesia, the most important opioids are the piperidines fentanyl, sufentanil, alfentanil, and remifentanil. These opioids produce potent analgesia and suppression of cardiovascular responses to noxious stimulation from surgery with predictable pharmacokinetics and pharmacodynamics.
1. The continued development of opioids with complex simultaneous actions at opioid and nonopioid target sites is facilitated by new information gained about mechanisms involved in endogenous pain and analgesia as well as advances in pain-related pharmacology.
2. Development of new opioids is also driven by concerns that the side effect profile of potent opioids, which presents a serious risk to patients, needs to be minimized.
II. THE ENDOGENOUS OPIOID SYSTEM
A. A major breakthrough in the understanding of opioid pharmacology came from a series of discoveries of opioid receptors, endogenous opioid peptides, their encoding genes, and endogenous opioid alkaloids.
B. The endogenous opioid system is composed of a family of structurally related endogenous peptides that act at a four-member opioid receptor family consisting of the μ-opioid receptor (MOR), κ-opioid receptor (KOR), δ-opioid receptor (DOR), and orphanin FQ/nociception (NOP) receptor.
1. At least three MOR subtypes have been described: μ1 is predominantly involved in opioid analgesia, μ2 is involved in opioid-induced respiratory depression, and μ3 is involved in opioid-induced immune suppression.
2. Functional validation of most opioid receptor subtypes awaits the development of antagonists with sufficient selectivity to allow a clear differentiation by effect.
3. The endogenous opioid peptides include endorphins, enkephalins, and dynorphins, each of which display different affinities for the μ-, κ-, and δ-opioid receptors. β-endorphins have a high affinity for the μ-opioid receptor, met- and leu-enkephalins for the κ-opioid receptor, and dynorphin A for the δ-opioid receptor.
C. Opioids act not only through central and peripheral neuronal pathways but also via nonneuronal mechanisms, such as actions on the immune system. Opioid receptors are linked to G proteins in the cell membrane and hence are members of the large G-protein–coupled receptor (GPCR) family. GPCRs mediate a cascade of downstream signaling pathways.
III. OPIOID RECEPTOR KNOCKOUT MICE
A. A variety of mice lacking various opioid receptors (“knockout mice”) have been bred to understand the molecular targets of exogenous opioids.
B. Mice lacking the MOR gene do not experience morphine-induced analgesia, respiratory depression, reward and withdrawal, inhibition of gastrointestinal transit, immunosuppression, or an increase in steroid hormones (Fig. 19-2).
C. These observations suggest the MOR is the target for both the desired and undesired effects of opioid analgesics. Consequently, designing a MOR-activating drug that selectively produces desired effects such as analgesia, but not undesired effects such as life-threatening respiratory depression, is not possible.
FIGURE 19-2. Effect of morphine in mice lacking the μ-opioid receptor (–/– = homozygous μ-opioid receptor knockout mice) and mice with intact receptors (+/+ = wild-type mice) on analgesic responses (A, tail flick test in two mice) and respiratory responses (B, the hypercapnic ventilatory response [HCVR] in two mice).
TABLE 19-1 CLASSIFICATION OF EXOGENOUS OPIOIDS
Synthesis
Natural (known as opiates): morphine
Semisynthetic (derived from the morphine molecule): codeine, heroin, hydromorphone, oxycodone, oxymorphone
Synthetic: meperidine, alfentanil, fentanyl, sufentanil, remifentanil
Potency (all may potentially produce serious side effects, including sedation, respiratory depression, hypotension, or bradycardia)
Weak: codeine, dextropropoxyphene, tramadol, hydrocodone
Medium: morphine, methadone, oxycodone, hydromorphone
Strong: fentanyl, sufentanil, alfentanil, remifentanil
Effect at Opioid Receptor
Full agonists: morphine, methadone
Partial agonists: buprenorphine at MOR
Antagonists: naloxone, naltrexone
Onset and Offset of Action
Rapid: remifentanil, alfentanil
Slow: morphine, buprenorphine
MOR = μ-opioid receptor.
IV. CLASSIFICATION OF EXOGNEOUS OPIOIDS. Opioids may be classified based on their synthesis, chemical structure, potency, receptor binding, and effect at the opioid receptors (Table 19-1). The term opioids is used for all opioids (endogenous opioid peptides, natural opioid alkaloids, semisynthetic opioids, and synthetic opioids).
V. OPIOIDS ACTING AT OPIOID AND NONOPIOID RECEPTORS
A. Most opioid analgesics act at multiple receptor systems with different affinities. (Morphine acts with high affinity at MOR and with lower affinities at KOR and DOR.)
B. Methadone is the most potent NMDA receptor antagonist. Antagonism of the NMDA receptor is clinically useful in reducing opioid tolerance and opioid-induced hyperalgesia (OIH) and in chronic pain states leading to pain hypersensitivity.
C. Nonopioids may also act at opioid receptors.
1. Ketamine is an NMDA receptor antagonist with affinity for multiple receptor systems, including the opioid receptors.
2. Its anesthetic properties are related to its effect at the NMDA receptors, and its analgesic effects are predominantly caused by MOR activation.
VI. OPIOID MECHANISMS
A. Mechanism of Opioid Analgesia
1. Opioids modify both nociception (reception of signals in the CNS) and the perception of a noxious stimulus (emotional coloring of pain).
2. Different types of peripheral sensory nociceptors, often free nerve endings, are stimulated by tissue damage, and the resulting pain information is transmitted to the spinal cord by two types of small-diameter peripheral afferent fibers: slow conducting, unmyelinated C fibers (which cause a dull burning pain) and faster, thinly myelinated Aδ fibers (which cause sharp, pricking pain).
3. μ-Opioid–induced analgesia and descending inhibitory pathways may be activated not only by exogenous opioids but also by activation of endogenous opioid systems.
a. Stress-induced analgesia. The endogenous opioid system is activated under stressful conditions, as demonstrated by the delayed onset of pain by soldiers wounded in battle.
b. Placebo-induced analgesia. The endogenous opioid system also mediates placebo-induced analgesia, a reduction of pain resulting from an expectation of pain relief.
c. Conditioning pain modulation (CPM) is a condition in which pain arising from a noxious stimulus applied to one part of the body is decreased by application of a second remote noxious stimulus. CPM is caused by the activation of descending inhibitory pathways.
B. Peripheral Opioid Analgesia
1. Opioids are involved in peripheral analgesia by acting directly on sensory neurons (Aδ and C fibers) to inhibit pain signal transmission (important in inflammatory pain).
FIGURE 19-3. Schematic diagram illustrating the role of opioids in analgesia of peripheral inflammation. Opioid-containing leukocytes are attracted to inflamed tissue by various chemokines and cytokines. Specific upregulated protein facilitates leukocyte migration through the vascular endothelium. In the inflamed tissue, leukocytes interact with releasing agents such as corticotropin-releasing factor (CRF), interleukin-1 (IL-1), and norepinephrine (NE) derived from postganglionic sympathetic neurons to secrete opioid peptides. These bind to peripheral opioid receptors, synthesized in the dorsal root ganglia and transported to peripheral endings of sensory neurons, to mediate analgesia. AR = adrenergic receptor; CRFR = corticotropin-releasing factor receptor; ICAM = intercellular adhesion molecule; PECAM = platelet endothelial cell adhesion molecule.
2. The immune system is also widely involved in peripheral analgesia (opioid receptors are located not only on neurons but also on immune cells, such as human leukocytes (Fig. 19-3).
C. Opioid-Induced Hyperalgesia and Tolerance
1. Opioids can induce the paradoxical effect of OIH (increase in pain sensitivity), which may limit the analgesic effects of opioids.
2. During long-term or high-dose opioid treatment, rapid opioid dose escalation, or administration of an opioid with rapid onset and offset (e.g., remifentanil), a paradoxical increase in pain accompanies the treatment escalation. The high incidence of OIH after remifentanil infusions may be related to its rapid offset of analgesia. To prevent severe pain responses after remifentanil-based anesthesia, administration of morphine (0.1–0.25 mg/kg) 45 to 60 minutes before the end of surgery is advisable.
3. OIH is not the same phenomenon as opioid tolerance.
a. Acute opioid tolerance caused by tachyphylaxis requires increasing doses of the opioid to reach a specific analgesic end point during the initial hours of opioid treatment.
b. Chronic tolerance, often seen in opioid abusers, occurs over days and manifests as a decreasing analgesic effect, resulting in dose escalation and increasing the likelihood of OIH.
VII. ROUTES OF ADMINISTRATION
A. The most important and predictable route of administration of opioids perioperatively is the intravenous route, since the amount of drug entering the systemic circulation is precisely known.
B. Opioids given orally have bioavailabilities of 20% to 40% caused by a rapid first-pass effect as a result of opioid metabolism in the liver.
C. Opioids given via the epidural (patient-controlled epidural analgesia) and intrathecal routes need to diffuse into the surrounding nerve tissue and spinal cord to activate MORs.
1. More lipophilic opioids (fentanyl, sufentanil) will penetrate faster and achieve higher concentrations into the spinal cord than hydrophilic opioids such as morphine and meperidine, yet they are also cleared from the spinal fluid more rapidly.
2. Thus, the lipophilic opioids may cause an early-onset respiratory depression, whereas morphine tends to cause late ventilatory depression due to its slow clearance from the spinal fluid.
D. Intramuscular injections of opioids for treatment of postoperative pain should be avoided because there are superior alternatives such as the intravenous (IV) administration of opioids using patient-controlled analgesia (PCA) devices.
VIII. PHARMCOKINETICS (PK) AND PHARMACODYNAMICS (PD)
A. When injected intravenously, opioids are rapidly transported to the heart and pulmonary blood vessels from where they are dispersed to various organs and tissues.
B. After a standard dose of opioid, the interpatient variability of effect is large and is related to a variety of factors, including weight-related parameters (lean and fat body mass), organ function (hepatic and renal function), and cardiac output.
C. When an opioid is injected into the venous system, there is an initial rapid peak in plasma concentration. Next, the drug rapidly enters multiple organ systems with high blood flow (e.g., the brain, liver, kidney) from which the plasma drug concentration rapidly drops followed by a slower drop caused by redistribution to organs (e.g., the muscles and later, tissues with high fat content) that are less well perfused.
D. The time needed for the drug’s plasma concentration to decrease by 50%, from a steady-state plasma concentration after the drug infusion has stopped, is called the context sensitive half-time (CSt½) (Fig. 19-4).
1. The CSt½ depends on the duration of the infusion.
2. For fentanyl, the CSt½ increases with the duration of the infusion. For remifentanil, the half-time is independent of the duration.
E. Metabolism: Which Pathways and Metabolites Are Clinically Relevant?
1. Most opioids are metabolized in the liver through either phase I (oxidative and reductive reactions catalyzed by the cytochrome P450 enzyme system) or phase II reactions (conjugation to a specific substrate).
2. Three aspects of opioid metabolism have clinical importance:
a. Medications that inhibit or induce the CYP450 system may increase or decrease the clinical effect of opioids by interfering with their metabolism.
b. Opioid metabolites may either be active or inactive (applies not only to their analgesic effect but also to their unwanted side effects).
c. Genetic variability in the CYP system has clinical implications.
F. Morphine undergoes rapid metabolism in the liver, and within minutes after its administration, the two most important hydrophilic metabolites (morphine-3-glucuronide [M3G] and morphine-6-glucuronide [M6G]) appear in the plasma. In humans, M3G is without any analgesic or antianalgesic action. M6G is a full μ-opioid receptor agonist.
FIGURE 19-4. Context-sensitive half-times for remifentanil, fentanyl, and morphine. A. Time to a 50% drop in concentration versus infusion duration. B. Time to a 75% drop in concentration versus infusion duration.
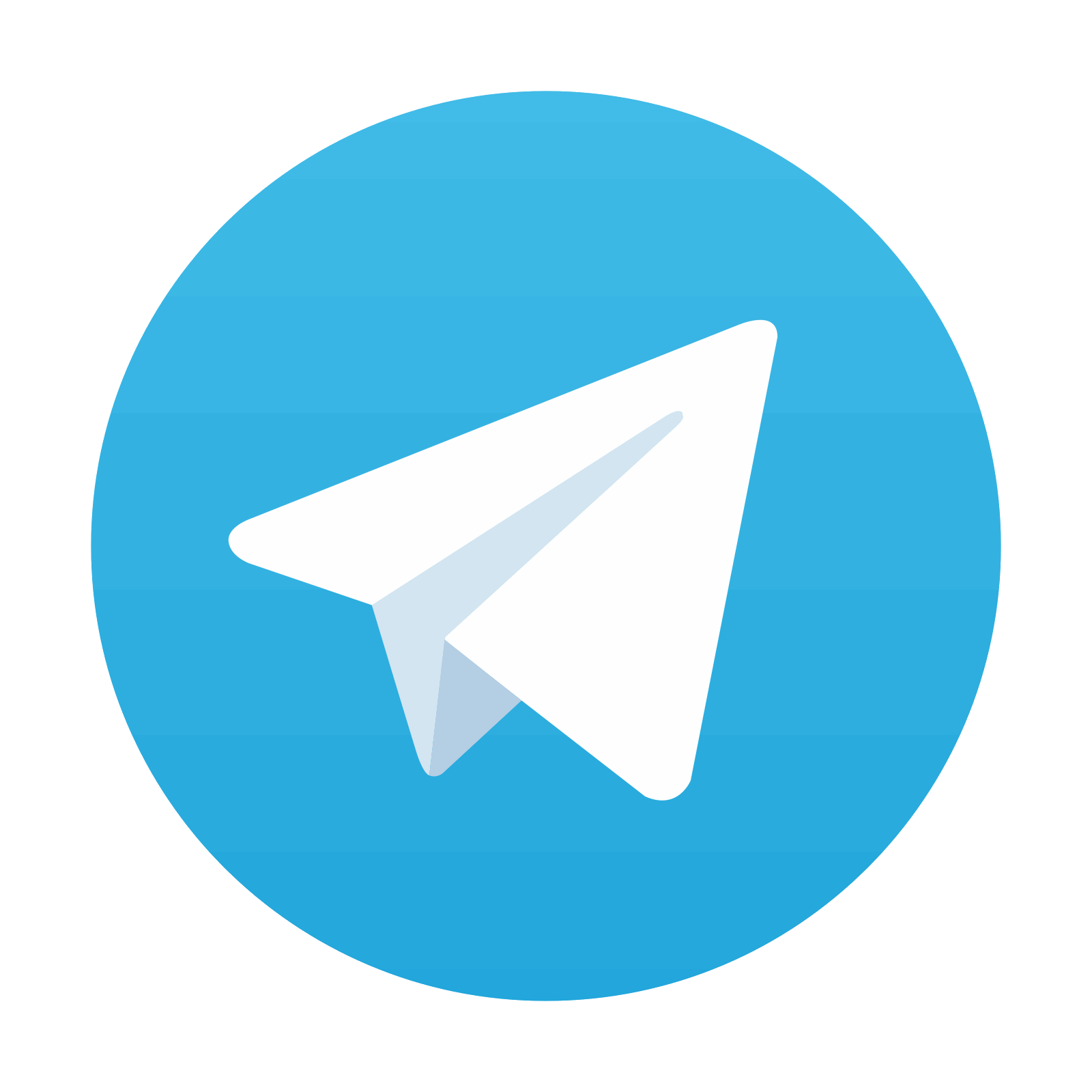
Stay updated, free articles. Join our Telegram channel

Full access? Get Clinical Tree
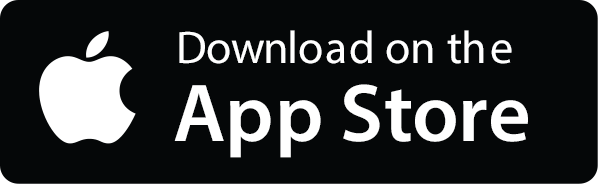
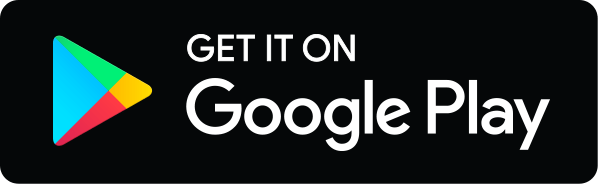