In 2012, there were 1.5 million persons with newly diagnosed invasive cancer in the United States.41 Among patients receiving chemotherapy for their disease, some will seek further care at an emergency department and require inpatient hospitalization for effects related to their treatment.10,35,38 In a prospective analysis of cancer registry data, 8.7% (179/2068) of patients receiving chemotherapy were admitted to the hospital for chemotherapy-related effects.14 Fever, neutropenia, and severe gastroenteritis are common reasons for inpatient care among patients receiving chemotherapy.14,21,35
The chemotherapeutics employed in curative and palliative regimens in many cases preclude cell division via a variety of mechanisms. Chemotherapeutics have also found expanded use in a variety of rheumatologic, dermatologic, and autoimmune disorders. Clinicians caring for patients affected by chemotherapeutics should understand the expected cellular responses from an initial and long-term exposure perspective. Chemotherapeutics cause damage primarily to deoxyribonucleic acid (DNA). This chapter will provide the reader with a focused review of cellular growth and proliferation, DNA damage from chemotherapeutics, the response of the cell to DNA damage, and the response of the tissues commonly affected by chemotherapeutics. This information will enhance the clinician’s management of patients affected by chemotherapeutics. New cancer therapies, such as targeted therapeutics and immunotherapies, are available for clinical use, and these therapies are discussed elsewhere (Chap. 50).23
Cell activities are growth, metabolism, proliferation, apoptosis, and senescence. The cell cycle is the classic paradigm that depicts cell proliferation in sequential steps that are represented by phases, and it is coordinated by the cell-cycle control system (Fig. 23–1).47 The phases are G0 (resting phase), G1, S (synthesis), G2, and M (mitosis). Stem cells tend to reside at G0. When cells are stimulated by a mitotic stimulant, such as a growth factor, they leave the resting phase and enter the cell cycle. G1-, S-, and G2-phases are known as the interphase of the cell cycle, and they encompass cell growth and DNA replication. G1 and G2 are growth or gap phases, and they allow for growth and regulatory processes that aid in the transition from the S-phase to the M-phase. The G1-phase is the most variable in duration among cell types, and it controls or determines the length of the cell cycle. A typical cell cycle takes about 24 hours, and mitosis takes about 20 to 60 minutes.15 The replication of DNA occurs in the S-phase, and mitosis and cytokinesis occur in the M-phase. The G2-phase likely serves to prevent the unintended consequence of a cell undergoing separation with an incomplete copy of the DNA. The fidelity of cellular duplication is ensured by checkpoints located throughout the cell cycle.
FIGURE 23–1.
Cell cycle specificity of chemotherapeutics: selected class of cell cycle phase–specific chemotherapeutics and their sites of action in the cell cycle. Arrows represent the progression of the cell cycle by phases, G0 (resting), G1 (gap1), G2 (gap2), M (mitosis), and S (DNA synthesis). The colors of each chemotherapeutic is matched with its cell cycle of activity. (Reproduced with permission from Brunton LL, Hilal-Dandan R, Knollmann BC. Goodman & Gilman’s: The Pharmacological Basis of Therapeutics, 13th ed. New York: NY: McGraw Hill Education; 2018.)

The process for DNA replication begins in the G1-phase, occurs in the S-phase, and ends in the G2-phase. There are several steps in the process, including identification of the origins for replication, incorporation of protein complexes at these origins, DNA unwinding, DNA elongation, and DNA termination. The cell enters mitosis at the completion of DNA replication.
In the G1-phase, the replication origins on the DNA are identified and the pre-replication complex (pre-RC) is formed. This complex consists of the origin recognition complex, MCM (mini-microsomal maintenance) (inactive helicase), CDT1, and CDC6, which facilitates the loading of MCM into the pre-RC. In the transition from the G1-phase to the S-phase, the pre-IC is formed and it activates helicase (via the CDC45-MCM-GINS {CMG} helicase complex), which begins DNA replication in the S-phase.
In the S-phase, DNA unwinds using the CMG helicase complex, elongates, and undergoes synthesis. The replisome accomplishes these steps during DNA replication, which includes assembly of supporting proteins, unwinding, polymerization, synthesis of RNA primers, and coordination of the polymerization between the leading and lagging strands of DNA.
The replisome is comprised of DNA polymerase, helicase, single-strand DNA binding protein, trimetric clamp processivity factor (enables DNA polymerase to synthesize a continuous strand of DNA on a template DNA without frequent dissociation), pentameric clamp loader complex, and primase. In addition, the replisome contains a translesion DNA polymerase, which allows the cell to bypass extensively damaged DNA and continue with DNA replication.
In general, the replication process proceeds in the following manner: DNA unwinds, forms replication forks, strands are stabilized by single-strand-binding proteins to prevent coiling, tension on the DNA helix in front of the replication fork is relaxed by topoisomerases I and II, and the ATR-ATRIP complex (ataxia telangiectasia mutated and Rad3-related-ATR-interacting protein) limits excessive firing or activation of origin complexes during the S-phase. Deoxyribonucleic acid elongation uses various DNA polymerases (ie, alpha, delta, and epsilon) in the synthesis of DNA. RNA primase forms a RNA primer that is used by DNA polymerase to synthesize a complementary chain to the template DNA chain in a 5′ to 3′ direction. In the leading strand, DNA is synthesized in a continuous manner as it follows behind the CMG helicase complex. In contrast to the leading strand, the lagging strand DNA is synthesized in fragments that are later joined to form a continuous strand. Deoxyribonucleic acid polymerase removes RNA primers and replaces the segments with deoxyribonucleotides. Deoxyribonucleic acid ligase joins remaining gaps between fragments. When DNA replication is complete, the cell will enter G2/M-phase.
Cellular injuries caused by chemotherapeutics are typically from a stress that impairs a DNA function, such as replication. Cells undergoing proliferation that are injured by stress to DNA will halt the cell cycle by activating a checkpoint, and it will attempt to repair the injury. If the repair is unsuccessful or there is a significant injury, the cell will undergo apoptosis or senescence. Stress may also be indirect, such as impairment of the spindle fibers during mitosis. When stress causes a structural change to DNA, such as a break in the strand of DNA or the presence of a bulky adduct, the result is considered a damaged DNA.43 Structural damage to DNA can be repaired; however, errors in the repair can lead to mutations. Mutation is a heritable change in the encoded nucleotide sequence of the DNA. Cells with mutations and a slow replication tend to accumulate over time, and they will develop into tumors that occur at an increased age of the host organism. Cells with mutations and a fast replication tend to develop into tumors at an early age.
Some chemotherapeutics cause DNA damage by forming bulky adducts, such as by alkylation or intrastrand crosslinks. For example, cisplatin causes DNA crosslinks (approximately 90% intrastrand and <5% interstrand); and nitrogen mustard, nitrosoureas, and triazenes cause alkyl-DNA adducts (Fig. 23–2).6 At very high doses, chemotherapeutics can cause additional toxicities (Chaps. 50 and 51). Some chemotherapeutics act at specific phases in the cell cycle and they are known as cell cycle phase–specific chemotherapeutics. For example, vincristine acts specifically during the M-phase, and cytarabine acts during the S-phase based on the mechanism of action (Fig. 23–1). These chemotherapeutics will not affect cells in the Go-phase. However, cell cycle phase–nonspecific chemotherapeutics, like alkylating agents, affect cells at all phases of the cell cycle.
FIGURE 23–2.
Mechanism of action of alkylating agents of mechlorethamine, a prototypic nitrogen mustard. (A) Activation reaction. (B) Alkylation of N7 of guanine. With bifunctional drugs such as nitrogen mustards, the second 2-chloroethyl side chain can undergo a similar activation crosslink of 2 nucleic acid chains or a nucleic acid to a protein. (Modified with permission from Rollins DE, Blumenthal DK. Workbook and Casebook for Goodman and Gilman’s The Pharmacological Basis of Therapeutics. New York: NY: McGraw-Hill Education; 2018.)

Cell senescence is considered a protective outcome because it arrests DNA-damaged cells, and it is a process of aging. The factors determining cell senescence are the cell type, type of damage, and severity of damage. Although the specific initiators of senescence are unknown, these items likely involve dysfunctional telomeres, damaged DNA, dysfunctional chromatin, oncogenes, and other types of stresses to the cell. The pathways involved with senescence are p53 (primary) and p16-pRB (retinoblastoma protein) (secondary).
Checkpoints work with the phases of the cell cycle to regulate cellular duplication so the process is accomplished in a correct manner. For example, cells with damaged DNA will not proceed in the cell cycle because this type of damage will lead to a cell with unstable genetic material during mitosis. Notable checkpoints are located at G1/S, the S-phase, the G2/M boundary, and the M-phase.51 The checkpoint at G1/S is the major one in humans. In the G-phase, DNA replication checkpoints arrest cell cycle progression and inhibit firing of late replication origins in response to stressors. The checkpoint in the S-phase slows down DNA replication and cell cycle progression when the DNA replication fork stalls. A stalled fork can result from an obstacle such as an adduct, a lack of substrates (eg, nucleotide), or an inactive enzyme.28 The checkpoint at G2/M is considered important for determining cell survival or death because many cells are observed to be halted at the G2-phase. In the M-phase, a cell with damaged DNA can undergo apoptosis (initiated by spindle assembly checkpoint {SAC}), undergo endoreplication (ie, DNA replication but no cell division), or return to mitosis.9 Cells with endoreplication will survive, but they cannot proliferate.
Cyclin-dependent kinases (Cdks) are a family of serine/threonine protein kinases that are responsible for the phosphorylation of specific proteins and the binding of inhibitory proteins in the cell cycle.7,40 Cyclin-dependent kinases are activated by rising concentrations of specific cyclins and Cdk-activating kinases, and inhibited by other proteins and protein kinases. For example, in the G1-phase, mitotic stimulants, such as growth factors, activate the complex cyclin D-Cdk4 by increasing cyclin D by gene expression, which binds to Cdk4. This complex activates the transcription factor E2F, which is important for entering the cell cycle. E2F promotes the expression of genes responsible for DNA synthesis and the production of cyclins E and A, which are needed for the G1/S transition and the S-phase, respectively.
Cyclin E complexes with Cdk2, which activates the inhibitory pRb that regulates EF2 to ensure the expression of genes specific to G1/S at the proper time in the cell cycle. Cyclin A complexes with Cdk2, which is involved with chromosome condensation and the firing of replication origins. In the M-phase, the increased concentration of cyclin A–Cdk complex, decreased concentrations of the protein kinases Wee1 and Myt1, and the increased concentration of the phosphatase Cdc25 activates the cyclin B–Cdk1 complex, which is necessary for the early stages of mitosis leading to metaphase and the activation of the anaphase-promoting complex (APC). The APC is a protease complex that degrades the remaining cyclins in the S- and M-phases, and it signifies the transition from metaphase to anaphase and the beginning of the exit from mitosis. When the cell leaves the M-phase and enters the G1-phase, the Cdk concentration is kept low by decreased gene expression of cyclin and increased cyclin degradation. The cell cycle is activated by increasing the concentration of cyclin D at this phase.
A cascading event of inhibitory proteins and protein complexes halt the cell cycle by inhibiting Cdk activity in the G1-phase when there is DNA damage. For example, when ATM kinase detects a DNA double-strand break (DSB) it rapidly halts the cell cycle by inhibiting the activation of Cdk2–cyclin through Chk2 and Cdc2. A later process that causes a prolonged cell arrest follows the rapid response and it involves p53. Ataxia telangiectasia mutated kinase activates p53, which causes p21 to bind and inactivate Cdk2–cyclin. When there is a stalled replication fork in the S-phase, ATR can activate p53 and p21 to halt the cell cycle. The cell cycle will restart after the DNA damage is repaired. If the damage cannot be repaired, p53 can remove the cell through apoptosis. The INK4 proteins (p15, p16, 18, and p19) inhibit the binding of Cdk4 and Cdk6 to cyclin D and the activation of cyclin D–Cdk, which halts the cell cycle at the beginning of the G1-phase.
The SAC consists of several proteins that monitor the alignment of the spindle at the metaphase plate, the attachment of the chromosomes to the spindles through the kinetochore, and the binding of the kinetochore to spindle fibers at opposite poles.7 If there is an irregularity in the centromere–kinetochore–microtubule interface or the tension among these structures, the SAC will inhibit the onset of anaphase by blocking the activation of APC, which is needed to activate the protease separase. Separase cleaves the protein complex that holds the sister chromatids together and this step initiates the separation of the sister chromatids during early anaphase. An irregularity in the spindle assembly results in chromosomal instability and abnormal chromosomes, such as aneuploidy. Microtubule inhibitors (eg, vinca alkaloids) prevent the proper assembly of the kinetochore microtubules and will activate the SAC.48
The DNA damage response (DDR) detects and repairs lesions in the DNA, and coordinates these activities with the cell cycle. The mechanism of the repair for lesions in the DNA depends on the type of the lesion and the location of the lesion in the cell cycle. When there is a lesion to a nucleotide or a single-strand break, the nuclear excision repair (NER) or the base excision repair (BER) mechanism is used to correct the lesion. When there is a double-strand break, the nonhomologous end joining (NHEJ) or the homologous recombination (HR) is used to correct the damage. Interstrand crosslinks in the DNA (caused by nitrogen mustards and cisplatin) are significant to the survival of the cell because these lesions cannot be easily repaired.6,29 However, intrastrand crosslinks in the DNA can continue on in the cell cycle after the lesion is addressed by the NER.18
The DNA repair systems for interstrand crosslinks involve the NER and translesional synthesis (TLS) when the cell is in the G0– or G1-phase, and HR when the cell is in the S-phase. The TLS allows DNA replication to proceed when there is extensive DNA damage by using a low-fidelity translesion polymerase. The cell’s capacity to repair the DNA also determines the repair mechanism. For example, a cell with a damaged DNA and with a mutation at p53 will bypass apoptosis and end up in the M-phase.13,34
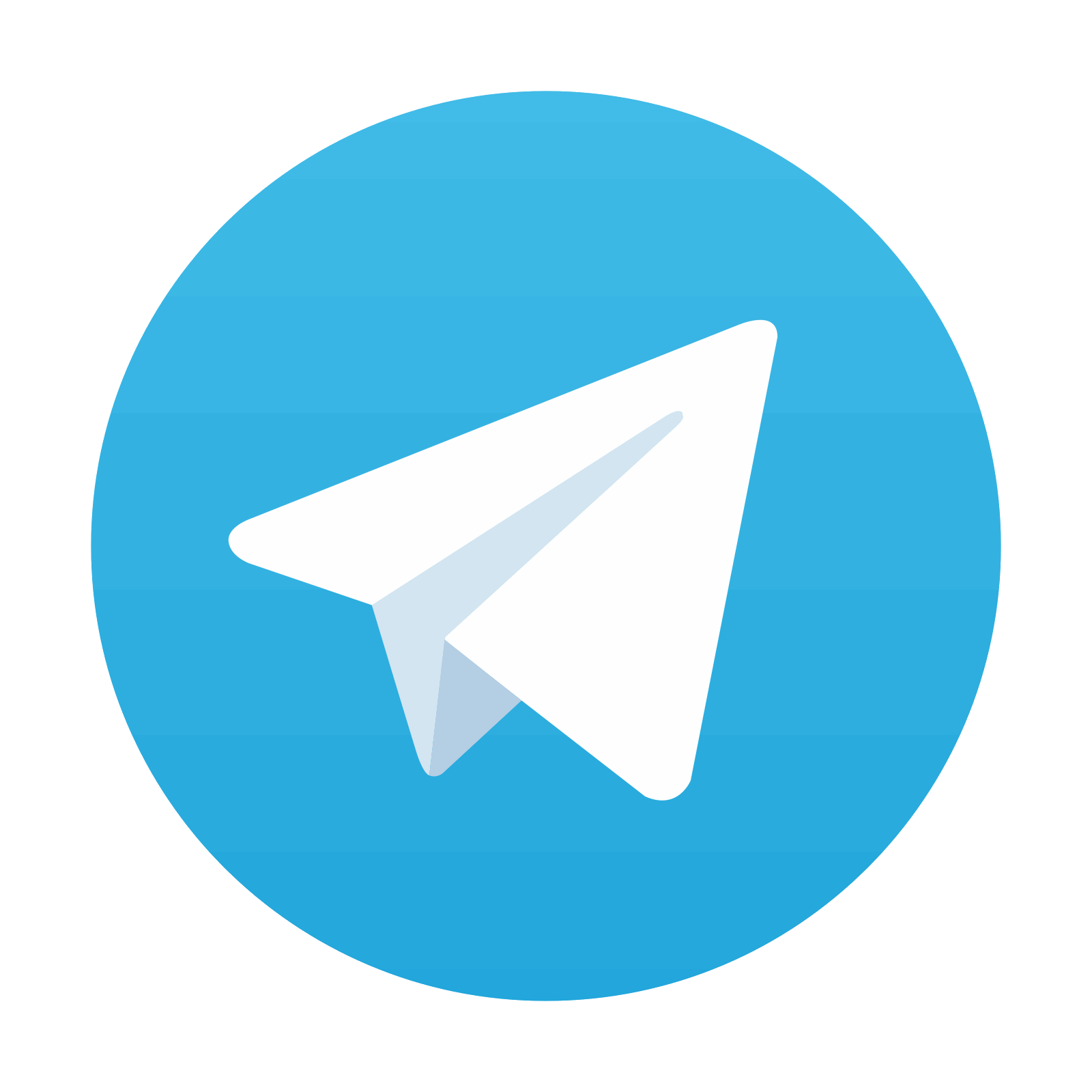
Stay updated, free articles. Join our Telegram channel

Full access? Get Clinical Tree
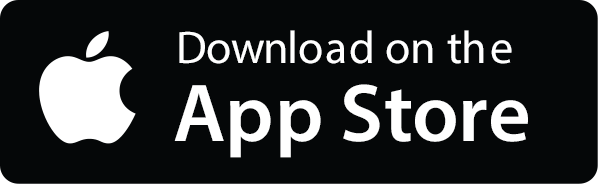
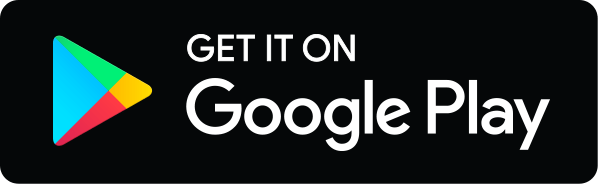