Heart Failure
Judy Cheng PharmD, BCPS
Heart failure is a public health problem of enormous and growing significance. According to the NHANES III data (MMWR, 1994), heart failure affects approximately 4.7 million people in this country. As the population ages and the incidence of patients surviving other symptomatic cardiac diseases such as myocardial infarction continues to rise, the incidence of heart failure and its mortality rate will continue to increase. Heart failure is the only cardiovascular disease that is increasing in prevalence.
The management of heart failure has become one of the most challenging problems confronting our health care system today. Each year, the United States spends nearly $9 billion in managing patients with heart failure.
Treatment of heart failure is no longer confined to symptom relief. Because the underlying etiologies that contribute to ventricular dysfunction may progress independently from the development of symptoms, treatment to prevent and delay the progression of the disease is equally important. This chapter will discuss the pathophysiology, treatment, and social impact of heart failure, with emphasis on long-term management and patient education to prevent exacerbation and delay the disease progress to improve the patient’s quality of life.
ANATOMY, PHYSIOLOGY, AND PATHOLOGY
Anatomy and Physiology of the Heart
FUNCTIONAL ANATOMY OF THE HEART
The heart is a four-chambered structure consisting of two atria and two ventricles. The atria are relatively thin-walled, low-pressure chambers that lie superior to the ventricles. Their primary function is to act as a reservoir, filling their respective ventricles. The ventricles are thick-walled chambers that function at higher pressures and pump blood from the heart into the respective great vessels. The atria and ventricles are more specifically identified as either right or left, according to their orientation in the chest. The right atrium and ventricle receive deoxygenated blood as it returns from the body. They then pump this blood to the lungs through the pulmonary artery. After the blood is oxygenated in the lungs, it returns via the pulmonary veins to the left atrium and is then pumped out to the rest of the body by the left ventricle via the aorta. The circulatory system thus consists of two circuits in series, the pulmonary and systemic, through which the blood sequentially flows.
The unidirectional blood flow is maintained by the four valves located in the heart. These valves allow the forward flow of blood and when closed prevent retrograde flow. The two atrioventricular valves, mitral and tricuspid, in the right and the left side of the heart respectively, separate the ventricles from their respective atria. The semilunar valves (aortic and pulmonic) divide the ventricles from their respective great vessel and artery. The left ventricle is separated from the aorta by the aortic valve; the right ventricle is separated from the pulmonary artery by the pulmonic valve.
CARDIAC PHYSIOLOGY AND HEMODYNAMICS
The primary physiologic function of the heart is to pump blood to supply oxygen and nutrients to the different body organ systems. Stroke volume is the volume of blood pumped per beat by each ventricle. Cardiac output is the volume of blood pumped per minute. Cardiac output and stroke volume are therefore related by the following equation:
Cardiac output (mL/min) = stroke volume (mL/beat) × heart rate (beats/min)
Stroke volume is regulated by three variables: the end-diastolic volume, the mean aortic or arterial blood pressure, and the contractility of the ventricles. End-diastolic volume (EDV) is the amount of blood in the ventricles just before contraction. Because this is a workload imposed on the ventricles before contracting, it is clinically referred to as the preload. Arterial pressure represents an impedance to the ejection of blood from the ventricles, or an afterload imposed on the ventricles after contraction has begun. The stroke volume is directly proportional to the preload and contractility but inversely proportional to the afterload.
The portion of the EDV that is ejected (the ejection fraction) against a given afterload depends on the strength of ventricular contraction. The left ventricular ejection fraction is one of the most important objective criteria used in describing the severity of heart failure. Normally, contraction strength is sufficient to eject two thirds of the EDV with each heart beat. In a healthy heart, this fraction remains relatively constant, even with an increase in EDV. This implies that the strength of ventricular contraction must increase as the EDV increases in a normal heart. Such intrinsic control of contractile strength was first described by two physiologists, Frank and Starling, and thus is named the Frank-Starling law of the heart.
The other factor that controls cardiac output is the heart rate. Heart rate is regulated by the autonomic nervous system. Stimulation of the sympathetic nervous endings in the musculature of the atria and ventricles increases the strength of contraction (positive inotropic effect) and decreases slightly the time spent in systole. On the other hand, enhancing the effect of the parasympathetic nervous system decreases cardiac rate and contractility.
Pathophysiology
PATHOPHYSIOLOGY OF HEART FAILURE
The pathophysiology of heart failure begins with myocardial cell damage caused by etiologies such as ischemic heart disease and hypertension. Table 11-1 details the causes of heart failure (Willerson & Cohn, 1995). As myocardial damage becomes significant, cardiac output decreases and intraventricular pressure increases to the point where body organ perfusion and functions are compromised. In the left ventricle, an increase in diastolic pressure leads to a rise in pressure in the pulmonary circulation. This causes pulmonary edema and prevents proper oxygenation of the blood, leading to dyspnea. In the right ventricle, an increase in diastolic pressure leads to an elevation of venous pressure, resulting in peripheral edema.
In the presence of a primary abnormality in myocardial contractility or excessive hemodynamic stresses, the heart relies on three major adaptive mechanisms in attempts to maintain cardiac output:
The Frank-Starling mechanism, in which an increase in preload brought about in part by salt and water retention helps sustain cardiac performance (stroke volume is directly proportional to preload)
Increased release of catecholamines by adrenergic cardiac nerves and the adrenal medulla activation of the renin–angiotensin–aldosterone system, and other neurohormonal adjustments that act to maintain arterial pressure and vital organ perfusion
Myocyte hypertrophy with or without chamber dilatation, in which left ventricular mass is increased in an attempt to enhance contractility.
Figure 11-1 illustrates the interactions of the different compensatory mechanisms in heart failure.
Initially, these mechanisms can maintain cardiac output, arterial blood pressure, and organ perfusion, but they may also exert more stress on the already injured myocardium. Therefore, in the later phase of the disease, such compensatory mechanisms actually contribute to the worsening of symptoms. Table 11-2 illustrates the short-term and the long-term responses of the impaired myocardium to these compensatory mechanisms.
HEMODYNAMIC COMPENSATORY MECHANISMS IN HEART FAILURE
In the early phase of heart failure, activation of the sympathetic nervous system increases both the heart rate and the contractile force, thus increasing cardiac output. However, an important cardiac compensatory mechanism called myocardial remodeling, which occurs during myocardial injury and in a state of enhanced sympathetic nervous activities, may be both adaptive and maladaptive (Braunwald, 1992; Grossman et al, 1975; Patterson & Adams, 1996). Unlike skeletal muscle cells, myocardial cells cannot divide to increase their numbers, but they undergo remodeling by which they increase in length or volume (dilatation and hypertrophy). Early in heart failure, dilatation and hypertrophy may enhance contraction, but chronically they often worsen cardiac damage. In addition, dilatation of heart chambers leads to an increase in myocardial wall stress, which is one of the determinants of myocardial oxygen demand and supply imbalance. Hypertrophy, if severe and longstanding, leads to loss of contractile force.
NEUROHORMONAL COMPENSATORY MECHANISMS IN HEART FAILURE
One of the most significant compensatory mechanisms of heart failure is the activation of certain endogenous neurohormones.
Reduction of cardiac output stimulates other body systems to try to maintain normal blood pressure and organ perfusion. The major neurohormonal systems that regulate these compensatory mechanisms are the sympathetic, renin–angiotensin, and atrial natriuretic systems.
Reduction of cardiac output stimulates other body systems to try to maintain normal blood pressure and organ perfusion. The major neurohormonal systems that regulate these compensatory mechanisms are the sympathetic, renin–angiotensin, and atrial natriuretic systems.
SYMPATHETIC NERVOUS SYSTEM
Plasma norepinephrine levels are increased in heart failure (Packer, 1988). With such activation of the sympathetic nervous system, there is an initial increase in cardiac contractility. Vasoconstriction also occurs and afterload increases. As mentioned, this is intended to enhance cardiac output and maintain vital organ perfusion. Chronically, however, this leads to an increase in systemic vascular resistance and adds to the strain of a failing heart. Increasing afterload leads to a decrease in blood perfusion to different organs, increasing preload (venous return to the heart). Vasoconstriction also leads to a reduction of blood flow to the kidneys, which stimulates the retention of sodium and water to compensate for the perceived lack of blood volume. Such retention of fluid and water does not improve stoke volume; rather, it contributes to the congestive symptoms of heart failure.
RENIN–ANGIOTENSIN SYSTEM
In states of low cardiac output, the renin–angiotensin–aldosterone axis is activated. This acts in concert with the activated adrenergic nervous–adrenal medullary system to maintain arterial pressure (Dzau, 1987). Renin is released by the kidneys in response to reduced blood flow. Renin converts angiotensinogen to angiotensin I in the circulation. Angiotensin I circulates to the lungs and other tissues, where angiotensin-converting enzymes (ACE) converts it to angiotensin II. Angiotensin II is a potent vasoconstrictor and therefore significantly increases afterload. Aldosterone production is also increased by angiotensin II. Aldosterone has potent sodium-retaining properties and contributes to the general volume overload state of heart failure. Finally, angiotensin II has an important role in the stimulation of the cell growth and development that leads to myocyte hypertrophy of the heart (Fig. 11-2).
Atrial natriuretic peptide (ANP) is a hormone produced by the atrial tissue of the heart. Its level is also increased in chronic heart failure (Packer, 1988). ANP is a counter-regulatory hormone that opposes the action of many of the vasoconstricting and salt- and water-retaining effects of the renin–angiotensin–aldosterone system. ANP acts as a vasodilating agent, suppresses the formation of renin, and enhances the excretion of salt and water. However, because of its relatively weak action and short duration of effect, it cannot totally reverse the detrimental effects of the renin–angiotensin system and the sympathetic systems. Investigational pharmacologic agents that mimic the action of ANP may have an important role in the management of heart failure.
EPIDEMIOLOGY
Each year, more than 2 million Americans suffer from heart failure, and approximately 400,000 new cases are diagnosed. Despite recent advances in the management of heart failure,
both surgical and pharmacologic, the mortality rate of this disease remains high. The 5-year mortality rate is approximately 50%, and 92% of the deaths are in people older than 65. Potential causes of this phenomenon include the increasing average age of the American population and the longer survival of people with other symptomatic cardiac diseases who subsequently develop heart failure at an older age (Gillum, 1993). Race-specific death rates have also reflected a higher incidence for younger African Americans. This may suggest an earlier onset of disease and a greater severity of heart failure among African Americans (MMWR, 1994).
both surgical and pharmacologic, the mortality rate of this disease remains high. The 5-year mortality rate is approximately 50%, and 92% of the deaths are in people older than 65. Potential causes of this phenomenon include the increasing average age of the American population and the longer survival of people with other symptomatic cardiac diseases who subsequently develop heart failure at an older age (Gillum, 1993). Race-specific death rates have also reflected a higher incidence for younger African Americans. This may suggest an earlier onset of disease and a greater severity of heart failure among African Americans (MMWR, 1994).
The management of heart failure patients exerts a heavy economic burden on society. Based on the information supplied by the Health Care Financing Administration, Medicare spends an average of $2.4 billion per year on patients admitted to the hospital with a primary diagnosis of heart failure (Konstam et al, 1994).
DIAGNOSTIC CRITERIA
Although the definition of heart failure remains unclear, the condition may be characterized by a pathophysiologic state in which the heart cannot provide adequate forward cardiac output to meet the perfusion and oxygenation requirements of the body organs and tissues. Although the etiologies of heart failure are numerous, the majority of cases can be classified as either systolic dysfunction, in which there is impaired cardiac contractility, or diastolic dysfunction, in which decreased compliance of the heart (manifesting as the heart’s inability to relax) impairs ventricular filling. Distinguishing between these disorders is important clinically because they are treated very differently. Nevertheless, there is a common symptomatology, including fatigue, shortness of breath at rest, dyspnea on exertion, peripheral/pulmonary edema, and weight gain caused by fluid retention and congestion.
Systolic and diastolic heart failures are also commonly referred to in clinical situations as congestive heart failure. Systolic heart failure, which is the more common form, is characterized by a dilated ventricular chamber with a poorly contracting ventricle and usually a thinned ventricular wall, ultimately producing a reduced left ventricular ejection fraction (<45%) (Cohn, 1996). In most laboratories, an ejection fraction of >45% to 50% is considered normal. In patients with pure diastolic heart failure, the ventricular chamber size may not increase and the wall thickness is usually enhanced. The most important diagnostic criterion, which distinguishes systolic and diastolic heart failure, is the left ventricular ejection fraction. Patients with diastolic failure may have a normal or increased left ventricular ejection fraction. Many patients with heart failure have elements of both systolic and diastolic dysfunction. Table 11-3 summarizes the major characteristics and diagnostic criteria of systolic and diastolic heart failure.
HISTORY AND PHYSICAL EXAMINATION
Obtaining a complete medical history is extremely important in diagnosing heart failure. Symptoms suggestive of heart failure include:
Paroxysmal nocturnal dyspnea
Orthopnea
Dyspnea on exertion
Lower extremity edema
Decreased exercise tolerance
Unexplained confusion, altered mental status, or fatigue in an elderly patient
Abdominal symptoms associated with ascites or hepatic engorgement (eg, nausea or abdominal pain).
Patients should be questioned about a previous history of angina or the equivalent (such as flash pulmonary edema), myocardial infarction, hypertension, other heart diseases, diabetes, and renal, pulmonary, thyroid, or gastrointestinal diseases. A complete medication history is also important to obtain. Table 11-4 lists some common medications that may cause or exacerbate heart failure symptoms. If symptoms of progressive dyspnea, orthopnea, or paroxysmal nocturnal dyspnea are present in conjunction with the above cardiac history, the likelihood that the patient has heart failure dramatically increases.
The physical examination can provide important information about the etiology of the patient’s symptoms and the appropriate therapies. Physical signs suggesting heart failure include:
Elevated jugular venous pressure or positive hepatojugular reflux
Third heart sound (positive S3)
Laterally displaced apical impulse
Bibasilar pulmonary rales that do not clear up with cough
Peripheral edema not caused by venous insufficiency.
The most sensitive physical finding is the third heart sound (Mattleman et al, 1983). Jugular venous distention and peripheral edema appear to be the least sensitive signs (Harlan et al, 1977). Because physical signs may not be highly sensitive and specific indications of heart failure, and some patients with moderate to severe left ventricular dysfunction do not manifest any physical signs, patients with symptoms highly suggestive of heart failure should be referred for echocardiography or radionuclide ventriculography to measure ejection fraction.
DIAGNOSTIC STUDIES
Recommended routine diagnostic studies for patients with suspected heart failure are listed in Table 11-5 (ACC/AHA, 1995). Many of these tests are recommended to rule out other diseases that have clinical symptoms similar to those of heart failure and to delineate the underlying causes of heart failure so they can be managed properly to reverse or prevent further progression of symptoms.
TREATMENT OPTIONS, EXPECTED OUTCOMES, COMPREHENSIVE MANAGEMENT
Systolic Heart Failure
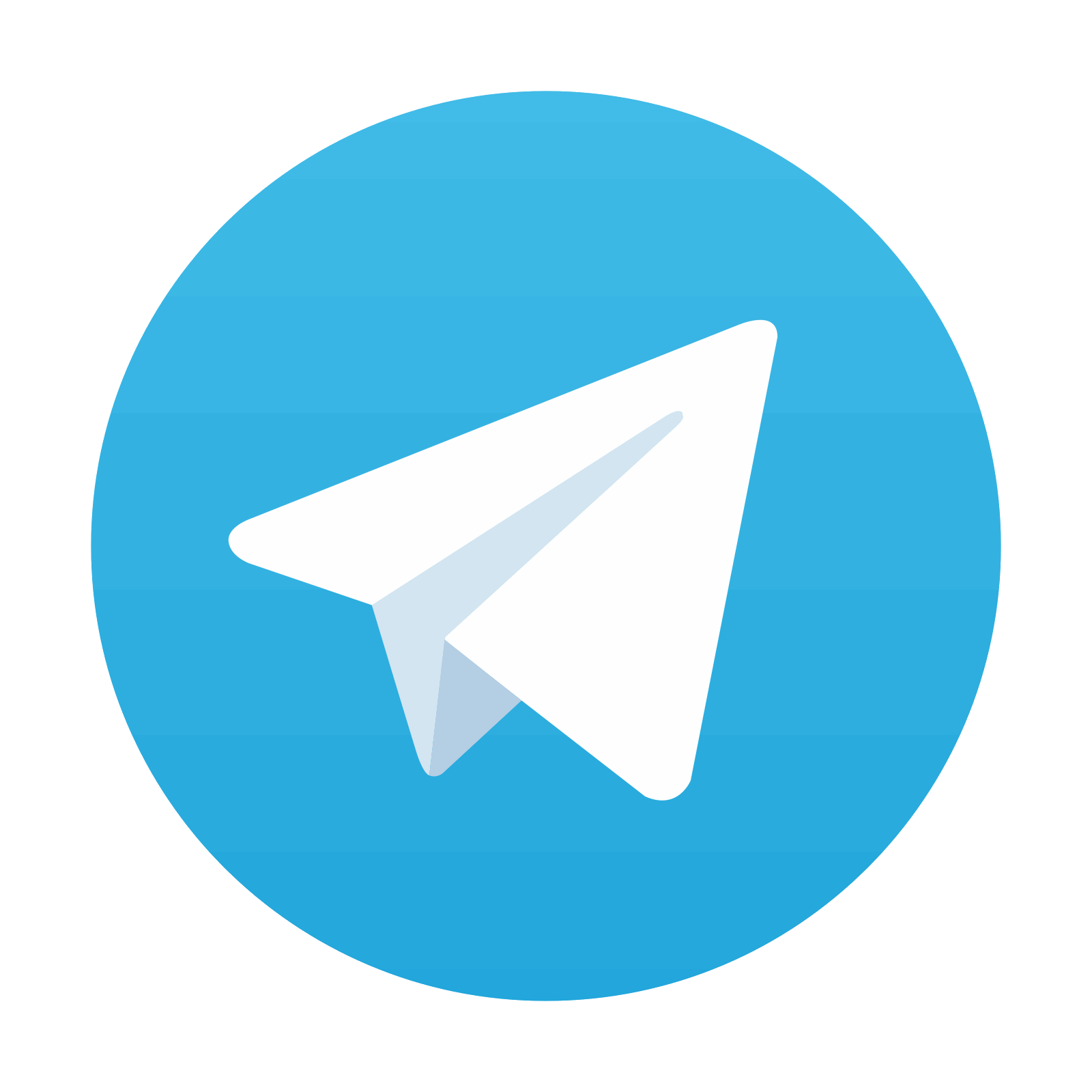
Stay updated, free articles. Join our Telegram channel

Full access? Get Clinical Tree
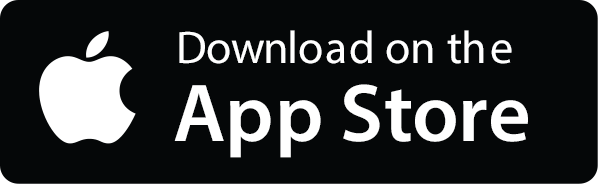
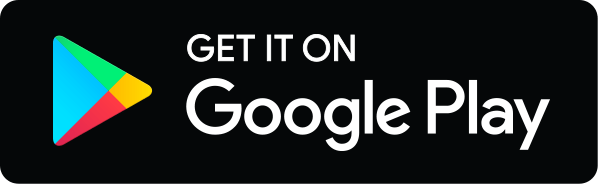