Volume
Guidelines for administration of fluid are often based on static hemodynamic targets such as central venous pressure (CVP), and delayed volume status indexes such as blood pressure, heart rate, capillary refill, and urine output. Traditional fluid management protocols also rely on estimates of fluid deficit, intravascular fluid volume status, fluid loss, and basal fluid requirements to guide perioperative fluid administration. Recent advances in hemodynamic monitoring have produced sophisticated dynamic measures of volume status such as stroke volume variation (SVV) and pulse pressure variation (PPV), which may serve as functional indexes for fluid administration.1 Volume administration is a concern in the perioperative environment because a limited cardiopulmonary reserve is a major risk factor for increased morbidity and mortality, when patients cannot meet the increased oxygen demand incurred during major surgery (Figure 22-1).
Vascular Tone
Bedside assessments of cardiorespiratory status and evolving systemic processes such as sepsis, acute lung injury, and hemorrhage are always masked by the body’s own compensatory mechanisms until the pathology is so advanced that the patient decompensates. There are multiple processes that are activated, depending on the clinical cause: hemorrhage, associated with a low cardiac output and impaired cardiovascular reserve; ischemic cardiomyopathy associated with cardiac pump failure; and pulmonary hypertension associated with pulmonary obstruction. All are associated with increased sympathetic tone, increased vasoconstriction, and tachycardia. Vasodilated states such as sepsis and spinal shock also increase sympathetic tone, causing tachycardia, although sympathetic output is insufficient to overcome the dilated peripheral vasculature.2
The literature on the role of hemodynamic monitoring in improving outcomes for critically ill medical and surgical patients provides two constant suggestions: (1) implementation of treatment protocols with specific hemodynamic targets and early initiation of resuscitation, before significant oxygen debt is incurred, and (2) application of treatment protocols as early as possible in the disease process. The target goals should have multiple endpoints that, together, ensure tissue perfusion (Figure 22-1). Avoid the use of a single static endpoint.
Goal-Directed Therapy
Goal-directed therapy is a term generally used to predefine resuscitation endpoints, which guide the use of inotropic, volume, and vasopressor therapies. Historically, goals have been driven by mean arterial pressure (MAP), urine output, and central venous pressure (CVP) or pulmonary arterial occlusion (wedge) pressure (PAOP). Fluid therapy is considered the first step in the resuscitation of most patients with hypotension and shock. Uncorrected hypovolemia, leading to inappropriate infusions of vasopressor agents, may increase organ hypoperfusion and ischemia. However, overzealous fluid resuscitation has been associated with increased complications, increased length of stay in the critical care unit in the hospital, and increased mortality.3–5
Hemodynamic measures are frequently based on the components of cardiac output (heart rate and stroke volume) as well as the balance of oxygen delivery and consumption. Dynamic changes in pulse pressure, stroke volume, or plethysmographic variability index (PVI) to assess perfusion, together with echocardiographic assessment of left ventricular (LV) function and size, provide useful information for assessing preload and preload responsiveness; however, these techniques do not allow for the detection and quantification of fluid excess or deficit.
CVP and PAOP are poor measures of volume status and cannot be used reliably to detect volume overload.6 Radiographic and clinical signs of pulmonary edema are also late signs of volume overload and provide a poor endpoint for fluid resuscitation.
Without a doubt, having a goal, which directs daily practice and intervention, is reasonable, but identifying the specific components of that goal remains controversial.7 The target endpoints should afford protection of the mitochondria, perfusion of the cells, and modulation of organ dysfunction.
Historical Milestones
In 2001, Rivers and colleagues redefined the focus of resuscitation for patients with septic shock. They demonstrated that early, target-focused interventions reduced mortality in sepsis.8 The 2001 study was critiqued for its single-center design and for the use of a specialized catheter to measure saturation of central venous oxygen (ScvO2). In addition, there was significant concern regarding the learning curve required for monitoring central venous oxygen in the emergency department.
In recent years, the terms early and goal-directed have been applied to the care of the perioperative patient,9,10 the patient with pancreatitis, survivors of cardiac arrest, patients with sepsis, and those with brain injury. However, the lack of consensus regarding early endpoint definitions continues to be problematic.11
Shock and Oxygen Delivery
All types of shock develop along a continuum. A simple continuum merely displays the difference between “early” (compensated) and “late” (decompensated) shock. This continuum underscores the concept well known to those in trauma as the “golden hour.” Compensated shock may be veiled while the internal compensatory mechanisms sustain blood pressure, and support the circulating volume and metabolism via a series of neuroendocrine events. The golden hour of shock is based on appropriate evaluation and intervention before the oxygen debt is too large to be repaid. Patients with limited cardiopulmonary reserve are at particular risk of mortality and morbidity.
Early augmentation of the oxygen delivery index (DO2I) with intravenous fluids and inotropes, also known as early goal-directed therapy (EGDT) has been shown to reduce postoperative mortality and morbidity in high-risk patients. Concerns regarding cardiopulmonary complications associated with fluid challenges and inotropes may deter clinicians from using goal-directed therapy interventions. Normal ranges that may be used as target values are listed in Table 22-1.
Table 22-1
Early Goal-Directed Therapy Target Values
>, Greater than; <, less than; ↑, increased; ↓, decreased; BSA, body surface area.
Of the various forms of circulatory shock, three distinct groups can be defined. Group 1 exhibits low stroke volume, or an inadequate cardiac output based on tissue demand, which profoundly impacts arterial oxygen delivery (DaO2) and mixed venous oxygenation (SvO2). Low stroke volume is a hallmark sign of hypovolemic, cardiogenic, and obstructive shock.
Group 2 has difficulty maintaining vascular tone and may have inappropriate flow. Low blood flow affects tissue perfusion pressure and may increase shunting, which, in turn, impairs organ perfusion. Loss of vascular tone occurs in septic, anaphylactic, and neurogenic shock.
Group 3 is identified by a failure to utilize oxygen, despite adequate delivery, as seen in severe sepsis and septic shock, where metabolic cellular defects impair the uptake and utilization of oxygen.
The role of a protocol is to provide a roadmap for clinicians to respond early and appropriately. In group 1 shock, low stroke volume is the problem. The vascular tone is generally responsive to endogenous and exogenous stimulants, depressants, and hormones. If the global problem is corrected by intravenous fluid administration, improvement in myocardial function, or relief of the obstruction, the peripheral tissue consequences of prolonged inadequacy of global oxygen delivery may not develop.
In group 2 shock, the failure of sympathetic nervous system activation, or the local opposition of mediators, promotes a widespread vasodilation that requires exogenous alpha activation and possibly neuroendocrine support. If treatment is delayed or ineffective, shock progresses to an anaerobic state, and organ failure rapidly follows.
Group 3 is the late-stage group, where manipulation of the “global” or delivery components of oxygen alone will be ineffective. Prompt effective treatment of “early” shock or compensated shock, may prevent progression to “late” shock or decompensated shock and organ failure.
Volume Resuscitation Assessment
Debate exists as to the optimal method of assessing volume responsiveness. The EGDT algorithm, adopted by the Surviving Sepsis Campaign (see Figure 20-1 in Chapter 20), relies heavily on CVP, MAP, and ScvO2 measurements. Other researchers have compared this algorithm against newer technologies to identify best patient outcomes.12–15
Bouferrache and associates (2013) compared use of transesophageal echocardiography with the recommendations in the Surviving Sepsis Campaign guidelines for consensus about when to administer fluid volume and inotropes in 46 mechanically ventilated patients with septic shock.12 Only a limited agreement existed between the two methods. Fluid loading was initiated when the index of collapsibility of the superior vena cava was more than 36%, and inotropic support was initiated when the LV fractional area change was less than 45% without collapse of the vena cava or increased vasopressor support. Only 70% of patients responded to volume resuscitation. In the remaining 30%, fluid loading did not lead to volume expansion, even though the CVP was less than 12 mm Hg. Transesophageal echocardiography demonstrated that 30% of the patients had poor LV function and received inotropes, whereas only 9% of the patients would have been started on an inotrope if the Surviving Sepsis Campaign guidelines were applied.12
Keller and colleagues retrospectively studied whether PPV coupled with CVP and PAOP impacted identification of fluid responsiveness of cardiac surgery patients under general anesthesia.13 Only 67% (31 of 46 patients) responded to fluid therapy. Neither CVP nor PAOP was a helpful predictor of fluid responsiveness, either alone or combined with PPV.
Broch and colleagues prospectively studied 92 patients undergoing coronary artery bypass surgery.14 The global end-diastolic volume index and respiratory variations in LV outflow tract velocity were analyzed and compared against PPV and SVV. The PiCCO system monitor, transesophageal echocardiography, and CVP measurements were obtained in all patients. Fluid responsiveness was identified by a greater than 15% increase in the stroke volume index in response to a passive leg raise test. In this study, both PPV and SVV were highly accurate in determining fluid responsiveness.14
Salzwedel and associates used a hemodynamic goal-directed algorithm incorporating PPV, cardiac index, and MAP in a prospective study of 160 patients undergoing major abdominal surgery. Compared with control, use of an algorithm decreased postoperative complications.15
Velissaris and associates examined whether ScvO2 values were associated with fluid responsiveness in 65 critically ill patients with severe sepsis. In this study, baseline ScvO2 was not related to fluid responsiveness.16
At least 10 randomized controlled trials have demonstrated that hemodynamic strategies based on PPV or SVV monitoring are associated with a significant reduction in postsurgical complications and hospital length of stay.17 An algorithm for stroke volume optimization is shown in Figure 22-2. Ongoing controversies about optimal volume assessment include the use of CVP-guided fluid management,18,19 the use of blood products to augment oxygen delivery,20,21 and vasopressor selection for the augmentation of MAP.22–25
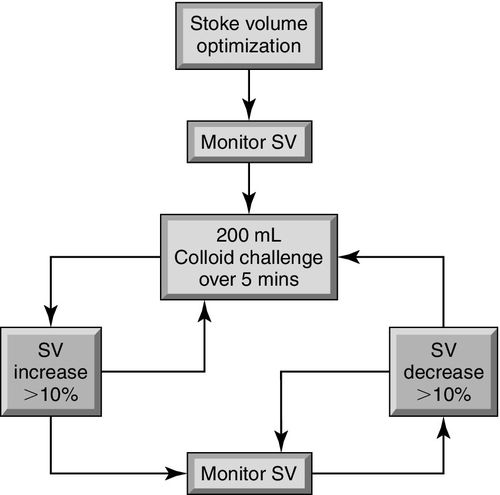
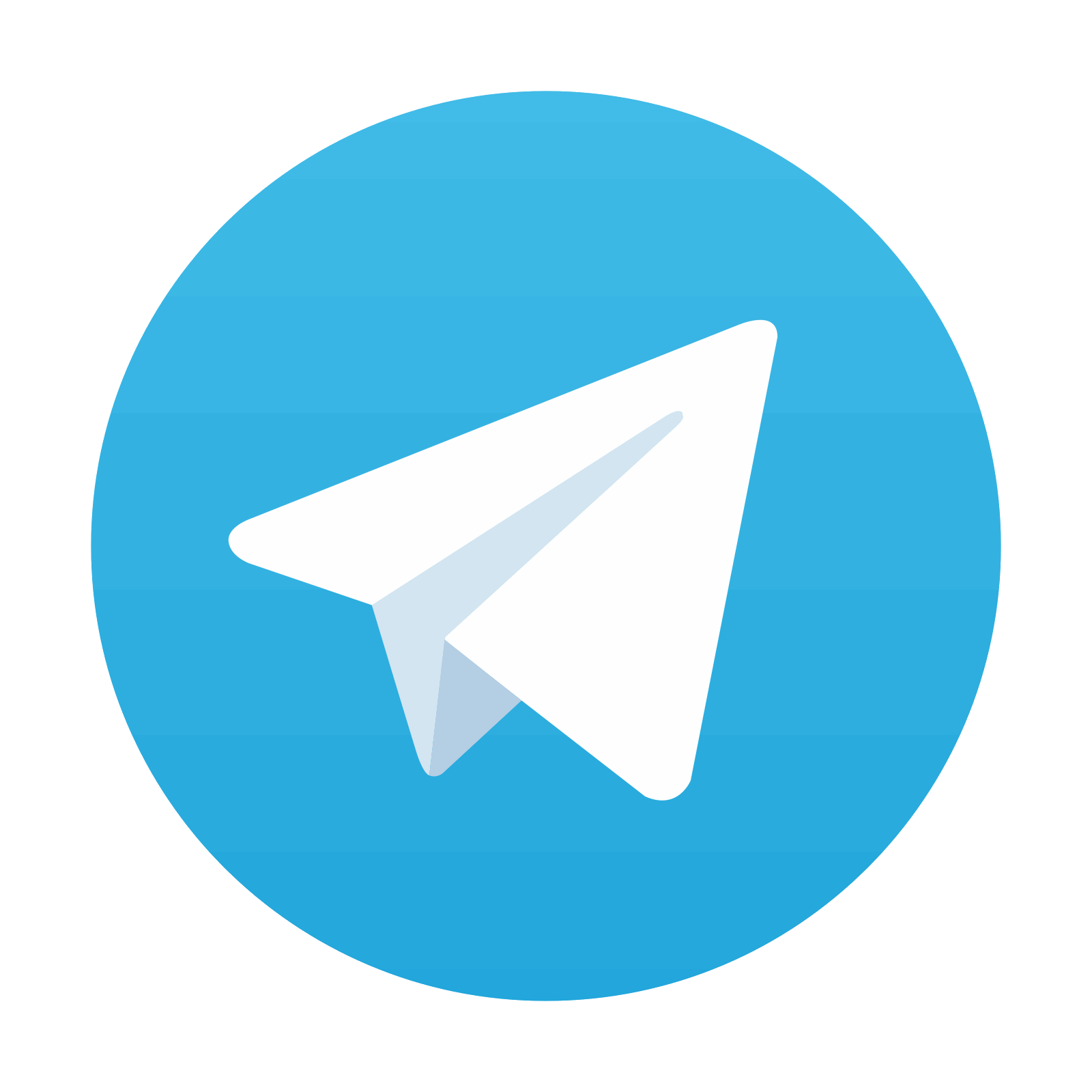
Stay updated, free articles. Join our Telegram channel

Full access? Get Clinical Tree
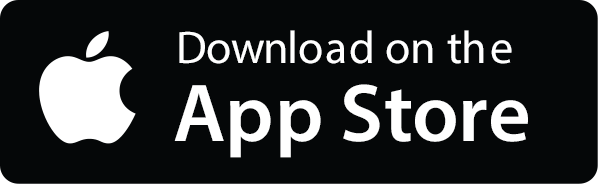
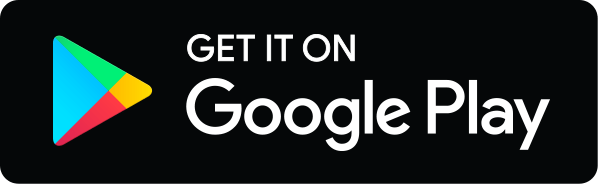