Chronic Renal Failure
Richard MacDougall RPA-C
The kidney has many important regulatory functions, such as body fluid volume, solute dilution and concentration, acid–base balance, and excretion of waste products. The kidney also secretes hormones that regulate red blood cell production, blood pressure, and calcium metabolism. Progressive loss of renal function—chronic renal failure (CRF)—regardless of cause affects these vital processes, with changes seen throughout all organ systems. The kidneys exhibit remarkable adaptive abilities, and symptomatic changes usually do not become apparent until the glomerular filtration rate (GFR) declines to less than 25% of normal.
When dealing with CRF, watchful waiting is the key phrase. The progression of the disease is slow, and the presenting symptoms are nonemergent. Baseline laboratory values are obtained. If hypertension is present, it is pharmacologically managed. The class of drug chosen depends on the etiology of the renal failure. Once the blood pressure is stabilized and any other presenting symptoms are dealt with, it becomes a matter of time. The creatinine level does not rise quickly, as it does in acute renal failure. In 1 year’s time, the creatinine level might rise one tenth of a point; the next year it may rise three tenths of a point. As long as the patient is hemodynamically stable, intervention is unnecessary.
While monitoring the patient’s renal function, the process that brought the patient to this stage must be understood. The goal here is to slow the progression toward dialysis or renal transplant. For this to happen, it is important to become familiar with the anatomy and physiology of the kidney, as well as the most common causes of CRF.
ANATOMY, PHYSIOLOGY, AND PATHOLOGY
Anatomy
The kidneys are paired organs located on the posterior abdominal wall, outside the peritoneal cavity. They lie on either side of the vertebral column, with upper and lower poles extending from the 12th thoracic to the 3rd lumbar vertebrae. Each kidney is approximately 11 cm long, 5 to 6 cm wide, and 3 to 4 cm thick. The right kidney is displaced downward by the overlaying liver and thus is lower than the left kidney. Each kidney is surrounded by the tightly adhering renal capsule and embedded in a mass of fat. The capsule and fatty layer are covered with a double layer of renal fascia, a fibrous tissue that attaches the kidney to the posterior abdominal wall. The medial indentation, called the hilus, serves as the entry and exit site for the renal blood vessels, nerves, lymphatic vessels, and ureters (see Fig. 30-1).
The major components of the kidney are the renal cortex and the renal medulla. The renal cortex contains all the glomeruli and portions of the tubules. The renal medulla contains a series of wedges called the renal pyramids. These pyramids are divided into outer and inner zones. The renal columns extend from the renal cortex down between the pyramids. The apex, which is the point of the renal pyramid, extends down into the minor calyx (a cup-shaped cavity) and joins with other minor calyxes to form the major calyx. The major calyxes join to form the renal pelvis, an extension of the upper end of the ureter.
INTERNAL STRUCTURE
The nephron is the functional unit of the kidney (Fig. 31-1). There are approximately 1.2 million nephrons per kidney. The nephron is a tubular structure made up of many subunits. The glomerulus is the tuft of capillaries that loop into a circular space called the Bowman’s capsule. The space within the capsule, known as Bowman’s space, descends toward the renal medulla. Bowman’s capsule then merges into the proximal convoluted tubule. The proximal convoluted tubule is made up of two segments. The initial convoluted segment is the pars convoluta. The straight separate segment is the pars recta, which descends to the renal medulla, forming the loop of Henle. As the loop of Henle emerges from the renal medulla, it becomes the distal convoluted tubule. The distal tubule is formed by straight and convoluted segments and extends from the macula densa to the collecting ducts. These ducts are large tubules that descend down the renal cortex and through the renal pyramids of the inner and outer medulla into a minor calyx. The glomerulus is lined with epithelial cells. Mesangial cells lie between the capillaries of the glomeruli and hold them together, forming Bowman’s capsule.
![]() FIGURE 31-1 Nephron, showing the glomerular and tubular structures along with the blood supply. (From Porth, Carol M. (1998). Pathophysiology, 5/e. Philadelphia: Lippincott-Raven Publishers. ) |
There are two kinds of nephrons. First are the cortical nephrons, which extend only partially into the renal medulla. They originate close to the surface of the renal cortex. The juxtamedullary nephrons, the second type, extend deep into the medulla. They are important for concentration of urine. The juxtamedullary nephrons are located deep inside the renal cortex, close to the renal medulla. There are structural differences between the two types of nephrons. The cortical nephrons have a short loop, so they may not extend into the renal medulla. The juxtamedullary nephrons have a loop that may extend the whole length of the medulla (40 mm); these represent approximately 12% of all nephrons.
The walls of the glomerular capillaries act as a filtration membrane and comprise three layers. The first is the inner capillary endothelium, which is composed of cells in continuous contact with the basement membrane. It is perforated by many small openings called fenestrae. The second layer is the middle basement membrane, a selectively permeable network of glycoproteins and mucopolysaccharides. The third layer is the epithelium.
This layer has specialized cells called podocytes, footlike structures that radiate from the epithelium and adhere to the basement membrane. The pedicle of one podocyte interlocks with the pedicle of an adjacent podocyte, forming an elaborate network of intercellular clefts called filtration slits. The glomerular filtration membrane separates the blood of the glomerular capillaries from the fluid in Bowman’s space. The glomerular filtrate passes through the three layers of the glomerular membrane and forms the primary urine.
This layer has specialized cells called podocytes, footlike structures that radiate from the epithelium and adhere to the basement membrane. The pedicle of one podocyte interlocks with the pedicle of an adjacent podocyte, forming an elaborate network of intercellular clefts called filtration slits. The glomerular filtration membrane separates the blood of the glomerular capillaries from the fluid in Bowman’s space. The glomerular filtrate passes through the three layers of the glomerular membrane and forms the primary urine.
As the glomerular filtrate leaves Bowman’s capsule, it enters the proximal tubular lumen, which consists of one layer of cells. This is the only surface inside the nephron that contains microvilli (a brush border). The microvilli create an expanded surface area inside the tubule, enhancing its reabsorptive function. The glomerular filtrate that is not reabsorbed in the proximal tubule then enters the loop of Henle. The loop is composed of two separate segments: a thin segment, composed of thin squamous cells with no active transport, and a thick segment. This area, constructed of cuboidal cells, actively transports several solutes out of the filtrate.
Toward the end of the loop of Henle, at its ascending loop, a transitional segment is formed. This is known as the macula densa. Here the distal tubule coming off the ascending loop passes between and contacts the afferent and efferent arterioles at their point of attachment to the macula densa and glomerulus. This linkage forms the juxtaglomerular apparatus. The juxtaglomerular apparatus has the important function of controlling renal blood flow, glomerular filtration, and renin secretion. Specialized cells in the walls of afferent and efferent arterioles secrete the hormone renin, which contributes to the control of arterial blood pressure.
BLOOD VESSELS
The renal arteries arise from the fifth branch of the abdominal aorta. As the renal artery enters the kidney at the renal hilus, it divides into anterior and posterior branches. These branches further divide into lobar arteries. The lobar arteries supply blood to the lower, middle, and upper thirds of the kidney. The interlobar arteries are further subdivisions of the lobar arteries that travel down between the renal columns and between the renal pyramids. At the corticomedullary junction, the interlobar arteries branch into the arcuate arteries. These vessels arch over the base of the renal pyramids and run parallel to the surface of the kidney. These further branch into the interlobular arteries, which extend through the renal cortex toward the periphery and form the afferent arterioles. These arterioles subdivide
into fistlike structures of four to eight glomerular capillaries. The blood supply proceeds through the glomerular capillaries and empties into the efferent arterioles, conveying blood into a second capillary bed, the peritubular capillaries. This is the only place in the body where an arteriole is positioned between two capillary beds. The positioning of the peritubular capillaries is important for glomerular filtration. The capillaries surround the convoluted portions of the proximal and distal tubules of the loop of Henle. There are two types of peritubular capillaries. The first type supplies the cortical nephrons. These capillaries are similar to the capillaries of other tissues. The second are the capillaries that supply the juxtamedullary nephrons. These capillaries, called vasa recta, form loops and closely follow the loop of Henle. The peritubular capillaries of the vasa recta are the capillaries that influence the osmolar concentration of the medullary extracellular fluid, which is important to the formation of a concentrated urine. These capillaries are the only blood supply to the renal medulla.
into fistlike structures of four to eight glomerular capillaries. The blood supply proceeds through the glomerular capillaries and empties into the efferent arterioles, conveying blood into a second capillary bed, the peritubular capillaries. This is the only place in the body where an arteriole is positioned between two capillary beds. The positioning of the peritubular capillaries is important for glomerular filtration. The capillaries surround the convoluted portions of the proximal and distal tubules of the loop of Henle. There are two types of peritubular capillaries. The first type supplies the cortical nephrons. These capillaries are similar to the capillaries of other tissues. The second are the capillaries that supply the juxtamedullary nephrons. These capillaries, called vasa recta, form loops and closely follow the loop of Henle. The peritubular capillaries of the vasa recta are the capillaries that influence the osmolar concentration of the medullary extracellular fluid, which is important to the formation of a concentrated urine. These capillaries are the only blood supply to the renal medulla.
All capillaries then drain into the venous system. The renal veins follow the same path as the renal arteries, only in the reverse direction.
Physiology
The kidney is a highly vascular organ, receiving approximately 20% to 25% of the cardiac output (1000 to 1200 mL blood/min). Approximately 20% of the plasma is filtered (120 to 140 mL/min) at the glomerulus and passes into Bowman’s capsule. The GFR is the filtration of plasma per unit of time. The GFR is directly related to the perfusion pressure in the glomerular capillaries. The rest of the plasma (80%) flows through the efferent arterioles to the peritubular capillaries. The ratio of glomerular filtration to renal plasma flow per minute is called the filtration fraction. All but 1 to 2 mL of the glomerular filtrate is normally reabsorbed and returned to the circulation by the peritubular capillaries.
The GFR is directly related to renal blood flow. This is regulated by three factors. Intrinsic autoregulatory mechanisms help keep the GFR stable and constant over changing arterial pressures (80 to 180 mmHg). Renal blood flow and GFR are constant; thus, solute and water excretion are constant. The second factor affecting renal blood flow is neural regulation. Neural regulation results in interruption of renal blood flow by the autonomic nervous system through sympathetic fibers that can cause vasoconstriction. Hormonal regulation is the third factor affecting renal blood flow. The renin angiotensin system produces an enzyme formed and stored in the cells of the arterioles of the juxtaglomerular apparatus. Numerous factors stimulate the release of renin, including:
Decreased plasma sodium
Decreased plasma potassium
Decreased blood pressure in the afferent arterioles (this reduces the stretch of the juxtaglomerular cells)
Sympathetic stimulation of the beta-adrenergic receptors on the juxtaglomerular cells.
The release of renin cleaves an alpha-globulin molecule (angiotensinogen) in the plasma to form angiotensin I. In the presence of a converting enzyme, angiotensin I is converted to angiotensin II and III. Angiotensin II stimulates the secretion of aldosterone by the adrenal cortex; this is a potent vasopressor and in turn inhibits renin release (a biofeedback mechanism).
Kidney Function
The function of the nephron is to form a filtrate of protein-free plasma. This process, known as ultrafiltration, occurs across the glomerular capillaries. The nephron then regulates the filtrate to help maintain body fluid volume and electrolyte composition within narrow limits. This fluid regulation happens through two processes. The first, tubular reabsorption, consists of movement of fluids and solutes from the proximal convoluted lumen into the peritubular capillary plasma. The second process, tubular secretion, is the active and passive transfer of substances from the plasma of the peritubular capillary into the lumen of the proximal convoluted tubule.
GLOMERULAR FILTRATION
The total volume filtered by the kidney in 24 hours is approximately 180 L. Changes in the GFR can occur with changes to the arterioles. For instance, constriction of the afferent arteriole will decrease the amount of blood entering Bowman’s capsule, thereby decreasing the GFR. On the other hand, constriction of the efferent arteriole will cause an increase in the pressure in the capillaries inside Bowman’s capsule, causing an increase in GFR. An endpoint to this is an increase in urinary excretion. By the time the filtrate travels to the end of the proximal tubule, 60% to 70% of the filtered sodium and water and 50% of the urea has been reabsorbed. In addition, 90% of the potassium, glucose, bicarbonate, calcium, phosphate, and uric acid have also been reabsorbed. All this is done by active transport. In the proximal tubule, active reabsorption of sodium is the primary function. Cotransported with the sodium are water, most electrolytes, and other organic substances.
At the loop of Henle, concentration or dilution of urine occurs, with additional concentration or dilution at the collecting ducts. When the process occurs at the loop of Henle, the strength of the concentrated filtrate is related to the length of the loop and the depth of penetration the loop has into the renal medulla. The primary function of the loop is to establish a hyperosmotic state within the medullary interstitial fluid. Each portion of the loop of Henle is permeable to different aspects of the filtrate. The thin descending segment is highly permeable to water and moderately permeable to sodium, urea, and other solutes. The thin ascending segment is more permeable to sodium but almost impermeable to water. The thick ascending portions of the loop of Henle are highly permeable to sodium, potassium, and chloride and less so to urea and water. The convoluted portion of the distal tubule is poorly permeable to water but readily absorbs ions and contributes to the dilution of the tubular fluid. Finally, the straight segment of the distal tubule and the collecting duct are permeable to water and sodium. The absorption rate of the water is controlled by antidiuretic hormone (ADH). Sodium is readily absorbed by the distal tubule and collecting duct under the regulation of the hormone aldosterone.
CLINICAL PEARL
When the GFR increases or decreases, the renal tubules, primarily the proximal tubules, automatically adjust their rate of reabsorption of sodium and water to balance the change in GFR.
URINE
Urea is an end product of protein metabolism and is the major constituent of urine, along with water. The glomerulus freely filters urea, and tubular reabsorption of urea depends on the urine flow rate. There is less reabsorption at higher flow rates. About 50% of the urea is excreted in the urine; the other 50% is recycled within the kidney. The recycling of urea from the tubules and collecting ducts contributes to an osmotic gradient in the renal medulla and is necessary for the concentration and dilution of urine. Because urea is an end product of protein metabolism, persons with protein deprivation will not be able to concentrate their urine maximally.
The final concentration of urine is controlled by ADH. ADH increases the permeability of water in the last segment of the distal tubule and along the entire length of the collecting ducts. The collecting ducts pass through the inner and outer zones of the renal medulla.
In the presence of ADH, water reabsorption is high. Most of the water is reabsorbed in the medullary collecting ducts because of the high osmotic gradient in the medullary interstitium. The water diffuses into the ascending limb of the vasa recta and returns to the systemic circulation. Excreted urine can have a high osmotic concentration (up to 1400 mOsm). The volume is normally reduced to about 1% of what is filtered at the glomerulus.
ADH secretion is one cause of oliguria (urine excretion of less than 30 mL/hr or 400 mL/day). In the absence of ADH, water diuresis takes place. The distal tubule and collecting ducts become impermeable to water. The water remains in the tubular lumen and is excreted as a diluted and large volume of urine. ADH has no effect on sodium reabsorption; it continues to be transported actively from the distal tubule and back into the renal vasculature.
RENAL HORMONES
Vitamin D is a hormone that can be obtained from the diet or synthesized by the action of ultraviolet light or cholesterol in the skin. These forms of vitamin D3 (cholecalciferol), from the diet or skin, are inactive and require two hydroxylations, one hepatic and one renal, to establish a metabolically active form, 1,25-dihydroxycholecalciferol (OH2D3). Vitamin D is necessary for the absorption of calcium and phosphate by the small intestine. The renal hydroxylation step is stimulated by the parathyroid hormone. A decreased calcium level (less than 10 mL/dL) stimulates the secretion of parathyroid hormone.
Serum phosphate fluctuation can also influence the renal hydroxylation of vitamin D. Decreased serum levels of phosphate stimulate active 1,25-OH2D3 formation. Increased phosphorus levels inhibit formation of 1,25-OH2D3. This results in compensatory phosphate absorption from the bone and intestine. The clinical significance of the role of the kidney in calcium and phosphate metabolism is evident in renal disease: patients with renal disease have a deficiency of 1,25-OH2D3 and manifest symptoms of disturbed calcium and phosphate balance.
Erythropoietin is a renal hormone that stimulates the bone marrow to produce red blood cells in response to perceived tissue hypoxia. The stimulus for increased erythropoietin release is a lowered oxygen delivery to the kidneys. The anemia of chronic disease produced by CRF may be caused by nonfunctional kidney cells and the lack of this hormone.
Natriuretic hormone is also referred to as atrial natriuretic peptide (ANP). Although not a renal hormone, it is a hormone that can affect the loss of sodium and water and the regulation of fluid balance. When the extracellular fluid volume is expanded, ANP secretion is increased and sodium reabsorption is depressed. This causes increased amounts of sodium and water to be excreted in the urine. ANP is released from cardiocyte granules located in the atria of the heart in response to the increased atrial stretch.
EPIDEMIOLOGY
Data on the incidence and prevalence of end-stage renal disease (ESRD) are available from the U.S. Renal Data System (USRDS, 1997). Based on these data, 68,870 patients developed ESRD in 1995, and 257,266 ESRD patients were alive in the continental United States. The incidence and prevalence of ESRD have grown each year; however, the growth rates may be decreasing. The incidence and prevalence of ESRD increase with increasing age, peaking in the eighth decade, with the mean age around 60 to 64 years. ESRD is more common in men than women and is highest in the African American population.
Diabetes, hypertension, and primary glomerulonephritis are the three most common attributed causes of ESRD. The incidence of diabetic, hypertensive, and glomerulonephritic ESRD from 1993 to 1995 was 94, 70, and 27 per million persons for each year, respectively; the prevalence was 274, 233, and 167 per million persons for each year. The difference between the diagnosis-specific prevalence and incidence rates indicates variations in average survival, with smaller differences suggesting higher mortality rates.
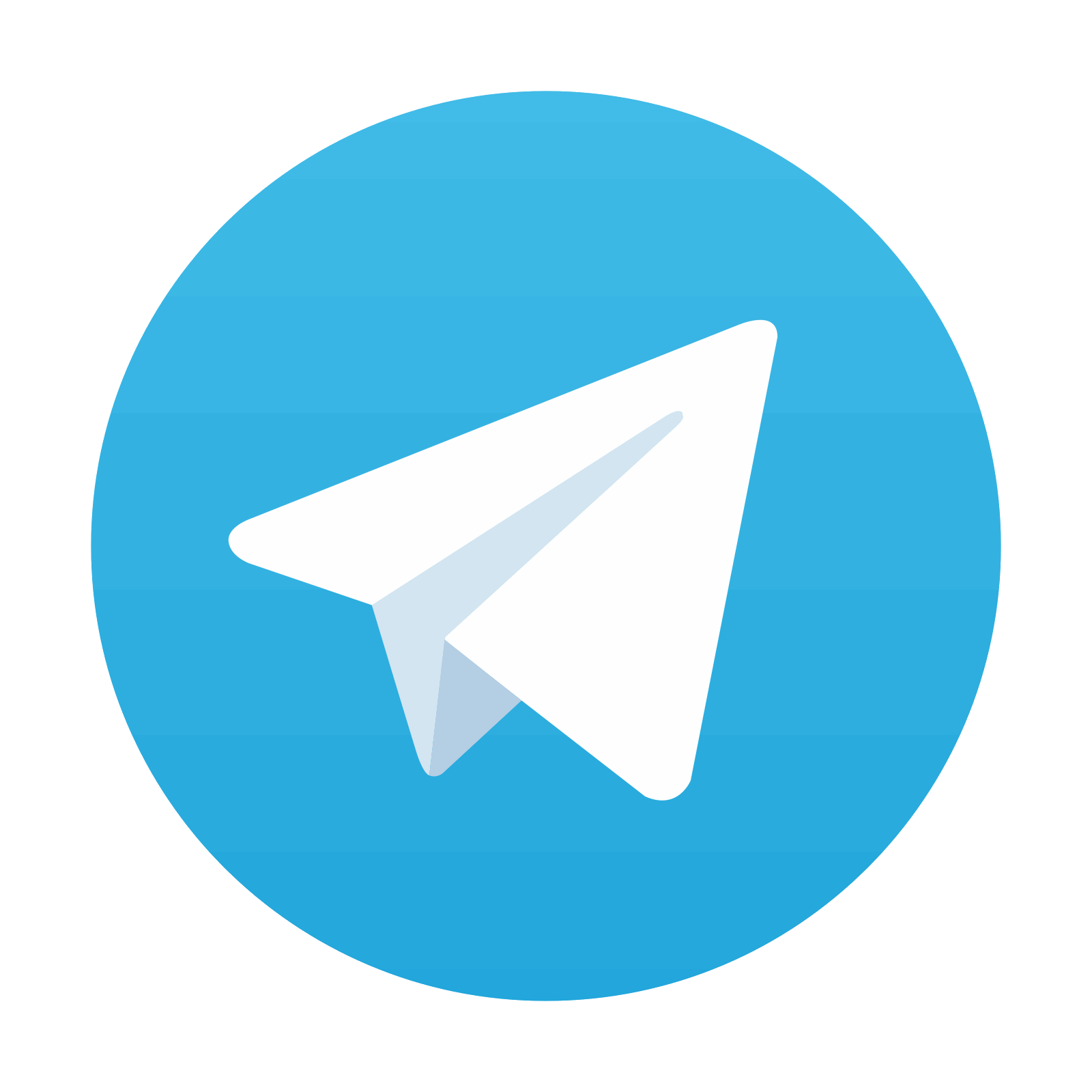
Stay updated, free articles. Join our Telegram channel

Full access? Get Clinical Tree
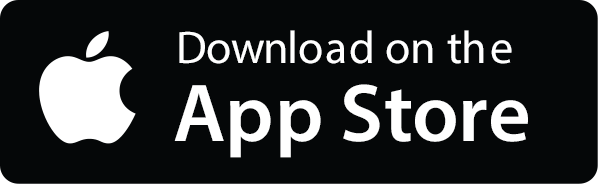
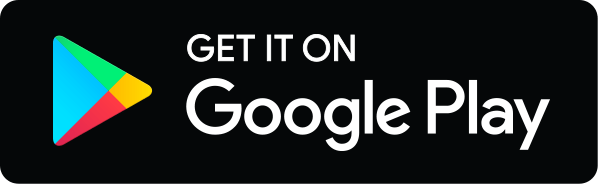