The coagulation process is divided into three phases: initiation, amplification, and propagation (Fig. 24.2).
Although coagulation is initiated by the extrinsic pathway, subsequent reactions reflect overlapping contributions from both the extrinsic and intrinsic pathways. In initiation, an exposed tissue-factor-bearing cell (normally external to the circulation) binds to factor VII, activating it and leading to the production of a small amount of thrombin. This small amount of thrombin is the catalyst for several events in the amplification phase: activation of platelets to stick to the damaged vessel, activation of platelet-derived factors V and VIII (which complex to factors Xa and IXa, respectively), and activation of factor XI to initiate the intrinsic pathway and increase the supply of factor IX. The propagation phase is marked by explosive generation of thrombin, driven by the activated coagulation factors assembled on the platelet surface. Thrombin cleaves fibrinogen to form soluble fibrin strands, which then polymerize into cross-linked, insoluble fibrin. Clot is subsequently revised and limited by plasmin, which is generated from the cleavage of plasminogen by plasminogen activators. Plasmin cleaves fibrin into fibrin degradation products of varying molecular weights, the most clinically relevant being d-dimer.
Clinically available drugs that affect the clot formation and revision process can be divided into the following groups by mechanism: vitamin K antagonists (coumarin derivatives), direct and indirect thrombin inhibitors, direct factor Xa inhibitors, fibrinolytics, and antiplatelet agents. The ideal agent would be one with clinical efficacy over a wide range of indications at a once a day dose that does not require monitoring, but does have an easily assessed means of quantitating clinical effect that is not toxic, has a low incidence of bleeding complications, and can easily be reversed. All currently available agents have some of these qualities, but unfortunately, none has all.
Vitamin K Antagonists
Vitamin K antagonists (VKAs) inhibit vitamin K reductase and vitamin K epoxide reductase, preventing the vitamin K-mediated gamma-carboxylation of coagulation factors II, VII, IX, and X and anticoagulants protein S and protein C. Without carboxylation, these factors are nonfunctional. Since this is a posttranslational step, VKAs affect only factors synthesized after their administration. Those synthesized before are still fully functional. Because the half-lives of the already formulated vitamin K-dependent factors may range from 6 (factor VII) to 72 (factor II) hours, the anticoagulant effect is not immediate and makes dose titration cumbersome. It should be kept in mind that the relatively short half-life of protein C creates an initial imbalance favoring thrombosis on initiation of warfarin therapy. Thus the patient should be treated with another anticoagulant (typically unfractionated or low-molecular-weight heparin) until therapeutic INR is reached.
Vitamin K antagonists are approved for use in primary and secondary prevention of venous thromboembolism, for prevention of systemic embolism in patients with prosthetic heart valves or atrial fibrillation, for prevention of stroke, for prevention of acute myocardial infarction (AMI) in patients with peripheral arterial disease, and for prevention of recurrent MI or death in patients with AMI [3]. The classic vitamin K antagonist is warfarin. Warfarin is an oral agent that is readily absorbed and highly protein bound (99 %) [4]. It is essentially entirely eliminated by hepatic metabolism via the microsomal enzyme cytochrome P450-C29. Genetic mutations in this enzyme or alterations in hepatic function may increase sensitivity to warfarin. Conversely, genetic mutation of vitamin K epoxide reductase may account for observed resistance to warfarin [5]. The list of foods, drugs, and clinical conditions that may affect the metabolism of vitamin K or warfarin (and thus the state of anticoagulation) is lengthy and includes antibiotics, antimycotics, antidepressants, antiepilepsy drugs, antiarrythmics, statins, and food plants which contain the plant-based vitamin K, phylloquinone.
The vitamin K-dependent coagulation factors are all part of the extrinsic/common pathway and accordingly are monitored for clinical effect by measurement of the prothrombin time (PT). PT varies by the thromboplastin used in the assay and thus for the purposes of standardization is adjusted for the particular thromboplastin used and reported as the INR. Target INR recommended by the American Academy of Chest Physicians is 2.0–3.0. Options for reversal of VKAs include withholding the drug and/or administration of vitamin K, fresh-frozen plasma (FFP), or prothrombin complex concentrates (PCCs) [6]. While it is recommended that patients may routinely receive vitamin K for an INR >10.0 without evidence of bleeding, the use of FFP or PCC should be reserved for clinically significant bleeding. In the case of severe, life-threatening bleeding, a PCC may be preferable to FFP administration [7], and if available a four-factor complex is preferable to a three-factor complex [8]. In countries where four-factor complexes are not available (e.g., the United States), there is evidence that a three-factor complex plus RVIIa [9], three-factor complex plus FFP and vitamin K [10], or possibly RVIIa alone may control bleeding [6].
Indirect Thrombin Inhibitors
Currently available indirect thrombin inhibitors include unfractionated heparin (UFH), low-molecular-weight heparin (LMWH), and fondaparinux. UFH, LMWH, and fondaparinux may all be administered subcutaneously, but only UFH may be administered as a continuous infusion.
Heparin is a mucopolysaccharide that binds to, and potentiates the activity of the naturally occurring serine protease inhibitor, antithrombin III (ATIII). Many of the coagulation factors are serine proteases, and thus the heparin/ATIII complex inactivates several coagulation factors, most importantly thrombin (factor IIa) and factor Xa. Heparin/ATIII binding occurs via a unique pentasaccharide sequence present on only some heparin molecules, accounting for some of the variability in dose-response among patients.
The clinical effect of heparin is monitored with the activated prothrombin time (aPTT) or activated clotting time (ACT). Although there are no randomized prospective trials examining the appropriate aPTT target for prevention of recurrent VTE, a range of 1.5–2.5 times control is generally accepted [11]. This acceptance is complicated by the fact that the measured aPTT varies by the reagents and instruments used to obtain it. Heparin resistance is the state characterized by the requirement of unusually high doses of heparin to achieve a therapeutic aPTT. This may be caused by ATIII deficiency (in which the patient paradoxically requires FFP to achieve anticoagulation because of the ATIII it provides) or increased heparin clearance or heparin binding. UFH may be reversed by protamine sulfate in a ratio of 1 mg per 100 units of UFH.
Unfractionated heparin consists of molecules ranging in molecular weight from 3,000 to 30,000 kDa. Only about one-third of these molecules contain the requisite ATIII-binding pentasaccharide sequence. Within this fraction, smaller heparin molecules containing fewer than 18 saccharide units (roughly 6,000 kDa) are not sufficiently long to mediate ATIII binding to thrombin, but can still catalyze ATIII/factor Xa inactivation. Since factor Xa inactivation is not reflected in the aPTT, this may be another reason for variability in observed patient response. UFH is cleared by both a rapid, saturable reticuloendothelial system and slower, largely renal mechanism. Although a large proportion of clearance is nonrenal, patients with severe renal disease may require dosage adjustment. Because of this two-system clearance, UFH has a context-sensitive half-life ranging from 30 min after an IV bolus of 25 U/kg to 150 min with a bolus of 400 U/kg [11].
Among the feared complications of heparin administration is heparin-induced thrombocytopenia. This is an immune-mediated, prothrombotic phenomenon caused by the production of IgG antibodies against a heparin-platelet factor 4 (PF4) complex. These antibodies are capable of platelet activation, followed by thrombin generation, further platelet consumption, and culminating in the clinical picture of thrombocytopenia and thrombosis. Because the formation of a heparin/PF4 complex depends on the size of the heparin molecule, the incidence of HIT with UFH is three times higher than with LMWH [12].
Low-molecular-weight heparin molecules range in molecular weight from 2,000 to 9,000 kDa. Compared to UFH fewer of these molecules are sufficiently long to bridge ATIII to thrombin, thus LMWH exerts more of its effect by inactivating factor Xa. With 90 % bioavailability, lack of significant protein binding, and a 3–6 h half-life, LMWH is appropriate for subcutaneous injection and is approved for VTE prophylaxis and treatment in addition to treatment of non-ST elevation acute coronary syndromes [11]. It is typically administered in a fixed or weight-adjusted dose depending on indication. The predictability of LMWH makes monitoring generally considered unnecessary, but if needed the anti-Xa level is the test of choice.
Elimination of LMWH is largely renal, and there is uncertainty when and how to adjust dosing in the setting of renal insufficiency. It is recommended that consideration be given either to an alternate agent or to dose adjustment and monitoring with anti-Xa levels for patients with a creatinine clearance of <30 cc/min [11]. Protamine sulfate has only partial efficacy in reversing the effects of LMWH, neutralizing its antithrombin activity but only a portion of its anti-Xa activity. Nonetheless, it is recommended that in the event reversal is required within 8 h of a dose of LMWH, protamine be administered in a ratio of 1 mg per 100 anti-Xa units of LMWH [11].
Fondaparinux is a synthetic pentasaccharide developed to mimic the action of the necessary ATIII-binding pentasaccharide present in UFH and LMWH. The molecular weight of fondaparinux is 1,728 kDa. Thus it is not sufficiently long to bridge ATIII and thrombin to inactivate thrombin, but is capable of anti-Xa activity. The half-life of fondaparinux is 17 h, and it is almost entirely eliminated by renal excretion. Like LMWH fondaparinux has high bioavailability after subcutaneous injection and negligible binding to plasma proteins other than ATIII, not only making monitoring unnecessary but also making it appropriate for once a day administration. However, almost exclusive renal elimination mandates dose adjustment in moderate renal impairment and avoidance in severe renal insufficiency (creatinine clearance <30 mL/min). Reversal is problematic as well because fondaparinux does not bind protamine. Fondaparinux is indicated for VTE prophylaxis in joint replacement or abdominal surgery. Evidence for the association of fondaparinux with HIT is on the order of isolated case reports. Nonetheless it is not recommended for use in HIT [13].
Direct Thrombin Inhibitors
Direct thrombin inhibitors exert their action without the involvement of ATII and by directly binding thrombin. There are four drugs of this class commercially available: hirudin, bivalirudin, argatroban, and dabigatran. Of these, only dabigatran is administered orally. The rest are parenteral agents. The parenteral direct thrombin inhibitors are primarily used to treat patients with HIT or who require anticoagulation and are considered at risk to develop HIT. Dabigatran is poised to rival warfarin as the agent of choice for chronic anticoagulation.
Of the two hirudin derivatives, lepirudin and desirudin, only desirudin is currently still available. The hirudins may be administered either intravenously or subcutaneously, with half-lives of 60 and 120 min, respectively [11, 14]. They bind to both free and fibrin-bound thrombin in an essentially irreversible complex which makes reversal problematic in the acute setting. Desirudin is approved for postoperative thromboprophylaxis in hip replacement surgery and in that role does not require monitoring [15]. The hirudins are almost exclusively cleared by the kidneys and thus must be dose-adjusted when creatinine clearance is below 60 mL/min. Additionally, they are highly immunogenic and have been associated with anaphylactoid reactions [16].
Bivalirudin is a hirudin analog with two advantages over the hirudins: it is less dependent on renal excretion, which accounts for 20 % of its elimination, and it has a half-life of 25 min [15]. It is approved as an alternative to heparin for patients who either have or are at risk for HIT and are undergoing percutaneous cardiac intervention. The currently recommended dose regimen is a bolus of 0.75 mg/kg followed by an infusion of 1.75 mg/kg/h. Bivalirudin may be monitored by aPTT measurement, with a target of 1.5–2.5 times control [17].
Argatroban is a small molecule derived from L-arginine that reversibly binds to thrombin at its active catalytic site. It is approved for treatment and prevention of HIT and as an alternative to heparin in patients undergoing percutaneous cardiac intervention who have HIT or who are considered at risk to develop HIT. It has a plasma half-life of 45 min and is metabolized in the liver by the P450 3A4/5 system. Thus it is particularly attractive for patients with renal impairment. Argatroban is administered as an intravenous infusion of 1–2 mcg/kg/min and titrated to an aPTT of 1.5–2.5 times control.
Dabigatran is an oral direct thrombin inhibitor that binds reversibly to the active site of thrombin. Although it is under evaluation for VTE prophylaxis after joint replacement, for secondary prevention of VTE, and for use in acute coronary syndromes, in the United States and Canada it is currently approved only for the prevention of stroke or systemic embolism in non-valvular atrial fibrillation. The pivotal trial supporting this was the Randomized Evaluation of Long-Term Anticoagulation (RE-LY) trial, which concluded that when used for stroke prevention in non-valvular atrial fibrillation, dabigatran at a dose of 150 mg twice a day was more effective than warfarin and associated with a similar rate of major hemorrhage. At a dose of 110 mg twice a day, dabigatran was as effective as warfarin and associated with lower rates of major hemorrhage [18].
Dabigatran is not well absorbed after oral administration and so is given as the prodrug dabigatran etexilate. Dabigatran is predominantly excreted by the kidneys and has a plasma half-life of 12–14 h [19]. Gender and body weight do not affect pharmacokinetics, but renal insufficiency and age do. Although age >75 years was not a condition of exclusion in RE-LY, a creatinine clearance of <30 mL/min was. Thus dabigatran is contraindicated in severe renal insufficiency, but dose adjustment may be appropriate in the elderly.
Unlike warfarin, dabigatran has no interactions with foods, is not metabolized by any enzymes of the cytochrome P450 complex, and has few drug interactions. For these reasons, monitoring is felt to be unnecessary. However, in the setting of emergency surgery or major hemorrhage, standard coagulation tests are likely to be inadequate to define drug effect. Although dabigatran prolongs aPTT and ACT, there is poor correlation between dabigatran plasma levels and measured aPTT or ACT. Thrombin time (TT) is overly sensitive to dabigatran, but may be used to determine presence of the drug. Both ecarin clotting time (ECT) and the proprietary HEMOCLOT thrombin inhibitor assay show a linear correlation between assay measurements and plasma concentrations of dabigatran at clinically relevant levels [20, 21]. However, neither is currently readily available.
Also problematic is the lack of a reversal agent for dabigatran. In the setting of major hemorrhage or emergency surgery, unlike the intravenous direct thrombin inhibitors, the half-life of dabigatran is sufficiently long that withdrawing the drug and waiting for the effect to dissipate may not be an option. Treatment is anecdotal and empirical but on the basis of animal studies, it appears that while 3- or 4-factor PCC, RVIIa, or an activated prothrombin complex concentrate (aPCC, e.g., FEIBA) may all be considered for reversal of catastrophic bleeding related to dabigatran, aPCC may be preferred if available [22–25]. Other measures to consider are activated charcoal in the setting of recent ingestion and dialysis since dabigatran is not highly protein bound. An open label study of a single dose dabigatran in patients with end-stage renal failure on dialysis found a mean difference in drug levels of 62 % between inlet and outlet lines after 2 h of dialysis [26].
Factor Xa Inhibitors
The limitations of warfarin prompted the development of new oral anticoagulants that target factor Xa. Factor Xa binds platelet-bound Va to form prothrombinase, the complex that converts prothrombin to thrombin. Each molecule of factor Xa generates about 1,000 molecules of thrombin through the prothrombinase complex in a key amplification step that results in a flare of thrombin generation at sites of injury [27]. These drugs are unaffected by dietary vitamin K intake, have a wide therapeutic index, and can be administered in fixed doses without routine coagulation monitoring [3, 8].
Oral factor Xa inhibitors are active compounds that interact with the catalytic pocket of factor Xa. They have mixed renal and fecal excretion and a rapid onset of action with good oral bioavailability [28]. Factor Xa inhibitors do not require monitoring and theoretically do not prevent the generation of sufficient amounts of thrombin for hemostasis.
In the orthopedic setting, rivaroxaban at 10 mg daily has been compared with enoxaparin for thromboprophylaxis in the four trials (Regulation of Coagulation in Orthopedic Surgery to Prevent Deep Vein Thrombosis and Pulmonary Embolism, RECORD 1, 2, 3, and 4). The rate of venous thromboembolism was significantly lower with rivaroxaban than with enoxaparin in patients undergoing elective hip or knee arthroplasty [29–32] with no significant increase in life-threatening hemorrhage. However, in a trial of medically ill patients (Venous Thromboembolic Event Prophylaxis in Medically Ill Patients, MAGELLAN), the rate of VTE was reduced with extended rivaroxaban prophylaxis, but this group also experienced more bleeding complications [33]. In patients with atrial fibrillation (Rivaroxaban Once Daily Oral Direct Factor Xa Inhibitor Compared with Vitamin K Antagonism for Prevention of Stroke and Embolism Trial in Atrial Fibrillation, ROCKET-AF), Rivaroxaban at 10 mg daily has been found to work as well as warfarin in the prevention of stroke or system embolism and significantly lowers rates of hemorrhagic stroke and fatal bleeding [34]. Rivaroxaban is licensed in the United States as an alternative to warfarin for stroke prevention in AF and for VTE prophylaxis after elective hip or knee arthroplasty.
Apixaban has also been evaluated for stroke prevention in AF (Apixaban for the Prevention of Stroke in Subjects with Atrial Fibrillation, ARISTOTLE). Compared with warfarin, apixaban at 5 mg daily was superior in preventing stroke or systemic embolism and produced significantly less major bleeding [35]. Compared to aspirin in the AVERROES trial, apixaban was also better at preventing stroke or systemic embolism in patients with AF and had similar rates of bleeding [36]. For thromboprophylaxis in the orthopedic setting (ADVANCE 1, 2, 3), apixaban was better than enoxaparin in patients undergoing knee or hip replacement surgery at preventing clots. Rates of bleeding were not significantly different [37–39]. Apixaban is FDA approved for use in stroke prevention in AF and in Canada and Europe for VTE prophylaxis after hip or knee arthroplasty.
Both agents are partially excreted by the kidneys, and the drugs are contraindicated in patients with a creatinine clearance (CrCl) less than 30 mL/min for VTE prophylaxis and less than 15 mL/min in AF. Rivaroxaban is excreted partially by the liver in a CYP-dependent manner. It has strong interactions with azole drugs such as ketoconazole and fluconazole. Amiodarone, diltiazem, and azithromycin are expected inhibitors of rivaroxaban metabolism, while rifampin, Dilantin, and St. John’s wort are potential inducers. Apixaban does not affect CYP enzymes and is expected to have few drug-drug interactions, though this has not been directly studied [40]. Factor Xa inhibitors should be stopped 24 h prior to procedures in patients with normal renal function and 2 days earlier for those with CrCl less than 50.
There is currently no reversal protocol for bleeding patients on these new agents. Use should be avoided in patients with hepatic disease associated with coagulopathy. They are highly protein bound and hemodialysis is ineffective. Activated charcoal can be used as an antidote to the drugs if recently ingested. A small study of healthy volunteers given rivaroxaban was able to show immediate reversal of drug and normalization of PT, PTT, and endogenous thrombin potential with nonactivated prothrombin complex concentrate (PCC) [41], though this has yet to be replicated in a larger clinical setting.
Fibrinolytic Drugs
Thrombolytic agents lyse fibrin by initiating the conversion of plasminogen to plasmin, which then degrades fibrin and fibrinogen. Several enzymes found in urine, blood, and tissues serve as physiologic activators of thrombolysis and served as a basis to develop the first generation of fibrinolytic drugs.
Of these, streptokinase is indicated for use in lysis of pulmonary emboli, extensive deep vein thromboses, and arterial emboli. In the setting of acute myocardial infarction, it has shown associated improvement in ventricular function and a mortality benefit when used to lyse intracoronary clots. Streptokinase may also be used to clear occluded arteriovenous cannulae. The streptokinase protein is derived from hemolytic streptococci and initiates fibrinolysis by forming an active complex with plasminogen. It is highly antigenic due to its bacterial origin, which limits its use. Another major limitation is the potential to cause life-threatening bleeding due to its systemic fibrinolytic activity [42]. Streptokinase has largely been supplanted by newer agents.
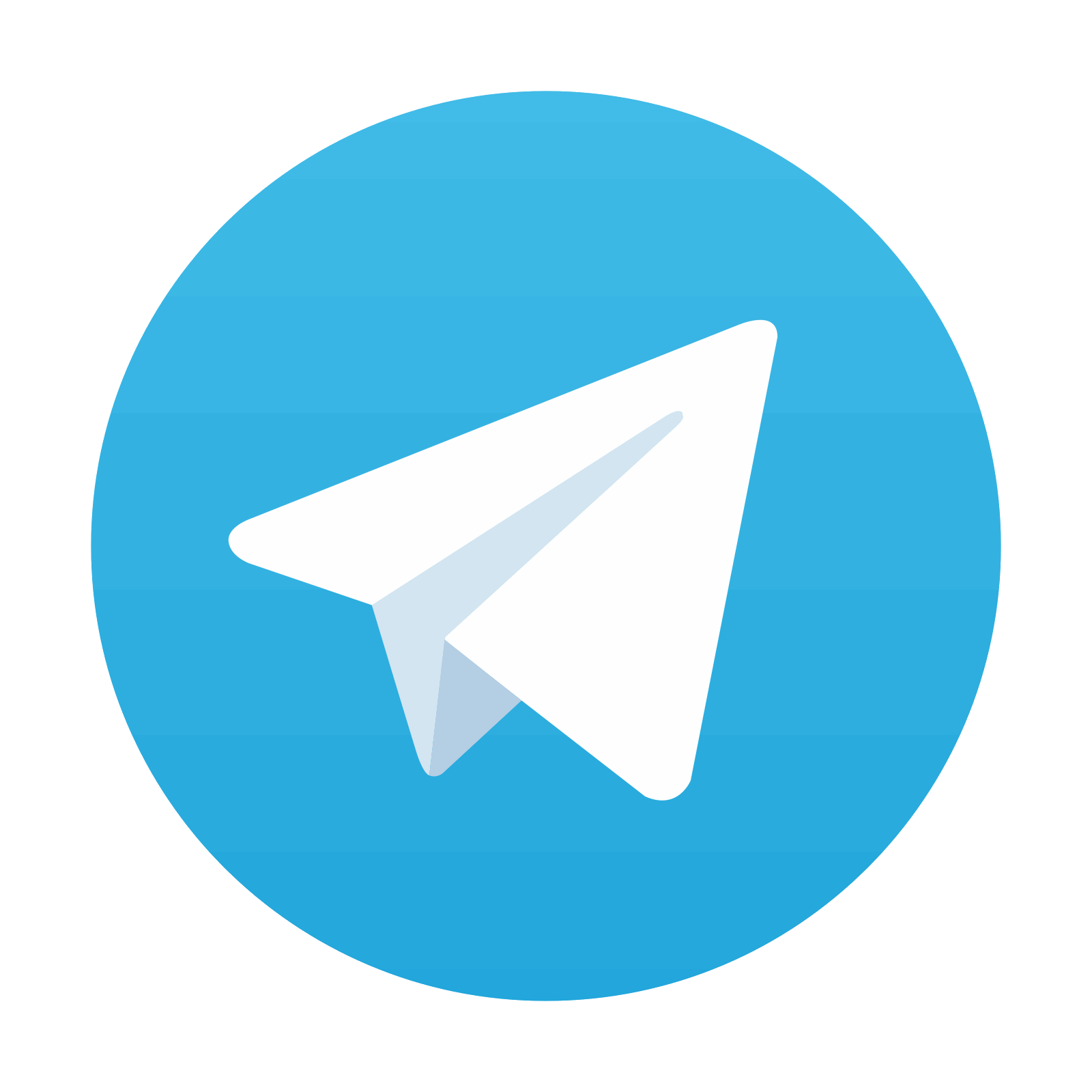
Stay updated, free articles. Join our Telegram channel

Full access? Get Clinical Tree
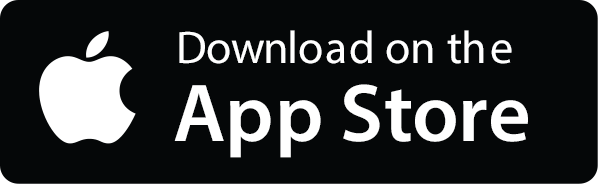
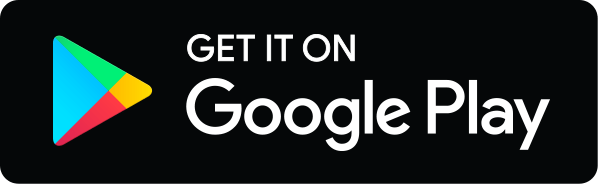