Stage
Description
Stage A
At high risk for HF, but without evidence of structural heart disease
Stage B
Evidence of structural heart disease, but without signs or symptoms of HF
Stage C
Clinical signs or symptoms of HF—current or past
Stage D
Refractory HF requiring specialized interventions
Pharmacologic Therapy and Implications
Ambulatory HF patients listed for heart transplant will be on neurohormonal antagonists and diuretics with or without inotropes. Neurohormonal blockers such as β blockers, angiotensin converting enzyme inhibitors (ACEI), angiotensin receptor blockers (ARBs), and aldosterone antagonists help in controlling the adaptive neurohormonal changes of HF with slowing down the progression of the disease. These medications are known to reverse the adverse remodeling and prevent left ventricular hypertrophy. They can also have a salient hemodynamic effect such as decreasing the afterload with improving myocardial performance. With anesthetic induction, some of these patients can have profound hypotension and may require high-dose vasopressors to maintain acceptable perfusion pressure. Even though there is lack of evidence in this subset of patients, one of the hypotheses is angiotensin being one of the important pressors maintaining blood pressure under conditions of anesthesia-mediated sympatholysis. These medications can result in hypotension by interfering with that pathway.
Diuretics are introduced into the treatment regime to treat fluid retention secondary to decreasing organ perfusion. They act at different sites on the renal tubule preventing sodium reabsorption resulting in diuresis (Table 13.2). Diuretics can cause significant electrolyte imbalance especially when associated with intravascular volume changes. Vasodilators are recommended to treat HF symptoms despite optimal medical treatment especially in the African American heart failure population and also as an adjunct in patients who cannot tolerate some neurohormonal antagonists. Hydralazine and nitrates are the common vasodilators used in current practice.
Table 13.2
Common diuretics used in advanced heart failure
Class of diuretics | Medications commonly used | Site of action |
---|---|---|
Loop diuretics | Furosemide, bumetanide, torsemide | Acts at distal ascending loop of Henle |
Thiazide diuretics | Hydrochlorthiazide, metolazone | Distal convoluted segments |
Potassium sparing diuretics | Spironolactone, triamterene, eplerenone | Antagonizes aldosterone effect on cortical collecting tubules |
Digoxin is a positive inotropic agent used in the treatment of symptomatic advanced HF resistant to other pharmacological therapy. It has a narrow therapeutic index and plasma levels that need to be monitored to prevent toxicity. Associated renal insufficiency and electrolyte abnormalities, which are common in this subset of patients, can further decrease the therapeutic window. Common features of toxicity are gastrointestinal disturbances (nausea, vomiting), neurological symptoms (confusion, yellow vision), and arrhythmias (conduction abnormalities and reentrant arrhythmias).
HF syndrome continues to progress relentlessly with decompensation of failure state and is a leading reason for hospital admission of elderly patients [18]. Readmission rates for acute decompensated heart disease remains the highest among all medical admissions to the hospital. Acute decompensation is often due to progression of underlying cardiomyopathic pathology with overwhelmed compensatory processes or can be de nova from acute myocardial injury (endocarditis, myocarditis, myocardial infarction). After treating precipitating cause (Table 13.3), treatment is directed towards improving cardiac performance by altering loading conditions and enhancing contractility or mechanical support, if necessary. These patients can be classified into different clinical profiles depending on the presenting signs and symptoms (Fig. 13.1). This profiling is derived from the initial Forrester Classification [19] of HF in patients presenting with acute myocardial infarction, and has shown to have prognostic implications with cold and wet patients having a 6-month mortality of up to 40 % [20].
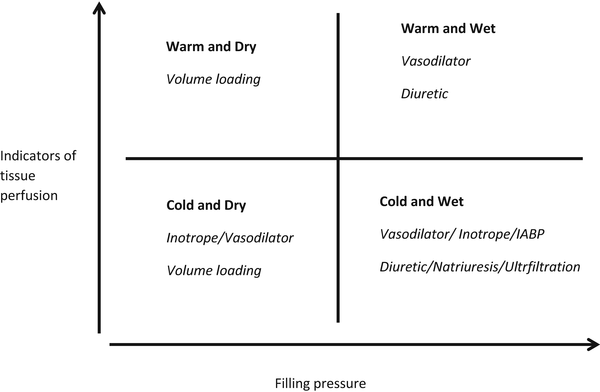
Table 13.3
Common precipitants for acute exacerbation of chronic heart failure
1. Noncompliance with heart failure treatment |
2. Arrhythmias |
3. Anemia |
4. Systemic infection |
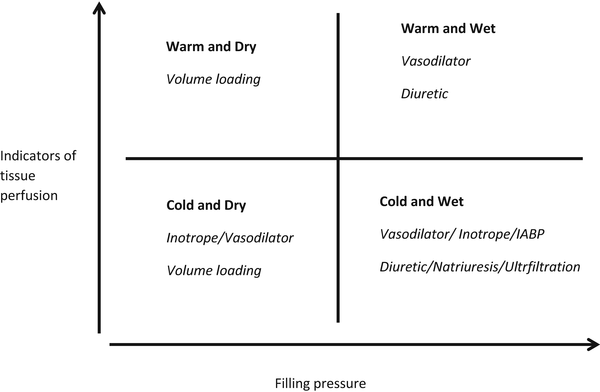
Fig. 13.1
Clinical profiles of decompensated heart failure and treatment options
These patients with significant perioperative implications (Table 13.4) are treated in monitored settings, quite often in intensive care units (ICU) for early detection of clinical deterioration and to titrate the treatment options to clinical effect. Supplemental oxygen is administered to maintain adequate peripheral oxygen saturation and, if necessary, noninvasive ventilation is used to decrease work of breathing. Caution needs to be exercised when altering the intrathoracic pressure in patients with right HF. Positive pressure ventilation and tracheal intubation is necessary if the patient is in respiratory distress or unable to protect the airway secondary to neurological deterioration.
Table 13.4
Preoperative implications of acute decompensated heart failure
1. Pulmonary edema and respiratory support |
2. High-dose diuretics and renal function |
3. Electrolyte imbalance |
4. Inotropic support and adequacy of systemic perfusion |
5. IABP in situ and position |
6. End organ function and altered pharmacokinetics |
Invasive monitoring is only indicated if the HF state is worsening with evidence of deteriorating organ functions in spite of adequate diuresis and afterload reduction. A pulmonary artery catheter (PAC) is used to guide the inotropic support by measuring the filling pressures and mixed venous saturation. In the failing heart, ventricles are operating on flat portion of Frank Starling relationship; increase in preload can have no incremental rise in cardiac output. Many of these patients are volume-overloaded upon admission and require parenteral high-dose diuretics. In resistant cases ultrafiltration is necessary to achieve desirable effect on preload. If the blood pressure is normal, afterload reducers are continued and intravenous vasodilators are introduced to achieve immediate reduction in afterload to improve cardiac output.
Common vasodilators used are sodium nitroprusside and Nitroglycerine (Table 13.5). Sodium nitroprusside is easily titrable and lacks tachyphylaxis, but has concerns for accumulation of toxic metabolites with organ dysfunction and higher total dose. Nitroglycerine can induce arterial vasodilation at higher doses and its use is limited by the development of tolerance within 24 h. Nesiritide, a recombinant product of human B-type natriuretic peptide is a potent vasodilator along with natriuretic properties that can decrease the filling pressures and improve congestive symptoms in decompensated HF. Given the associated cost without any demonstrable clinical benefits over other vasodilators, its use is limited in the current management of HF [21]. Vasodilators can result in significant hypotension in the setting of low intravascular volume and anesthetic induction. Given the fact that HF state can worsen with discontinuation of afterload reducers, and the intravenous vasodilators used have short effective plasma half-life, these medications are continued until anesthetic induction.
Table 13.5
Dosing of intravenous vasodilators in decompensated heart failure
Nitroglycerine | Start at 5–25 μg/min Titrate up to 200 μg/min | Hypotension, tolerance |
Sodium nitroprusside | Start at 0.2–0.4 μg/kg/min Titrate up to 5 μg/kg/min | Hypotension, cyanate toxicity |
Nesiritide | Bolus 2 μg/kg Infusion 0.015–0.03 μg/kg/min | Hypotension |
If the congestive state does not improve or the low output state continues to worsen with adequate diuresis and afterload reduction, inotropic support (Table 13.6) is considered. There should be evidence of organ hypo perfusion and low measured cardiac output before administering inotropic agents [22, 23]. Inotropic agent should be viewed as an intermediary bridge to transfer the critically ill patient to more definitive treatment options. They need to be discontinued as early as possible as the clinical condition is stabilized, as evidence points towards increase in short- and long-term mortality with their use [24]. Invasive blood pressure and filling pressure monitoring are essential for optimal titration of the vasodilators and inotropic medications to desirable effects avoiding hemodynamic side-effects. Epinephrine is reserved for cardiac arrest or impending hemodynamic collapse and is not recommended for the treatment of HF. Levosimendan being a calcium sensitizer and KATP inhibitor has a theoretical benefit over adrenergic agonist by not downregulating the adrenergic receptors. Conclusive clinical evidence is lacking even though a recent meta-analysis from 45 randomized clinical trials suggests a mortality benefit of using levosimendan in cardiac surgery patients [25]. Levosimendan is not yet approved by the Federal Drug Administration (FDA) for the treatment of HF. Vasopressors (nor-epinephrine) are only indicated in a resistant heart failure state when inotropic support and fluid challenge fail to increase the systolic blood pressure above 90 mmHg and improve the organ perfusion.
Table 13.6
Common inotropes used in treating acute decompensation of heart failure
Dobutamine | 2–20 μg/kg/min | Dose-dependent adrenergic agonist |
Milrinone | Optional bolusa—50 μg/kg over 20 min Infusion—0.25–0.75 μg/kg/min | Type 3 phosphodiesterase inhibitors |
Levosimendan | Optional bolusa—12 μg/kg over 10 min Infusion—0.05–0.2 μg/kg/min | Calcium sensitizer Binds to Troponin-C |
Preoperative Mechanical Circulatory Support
In resistant cases of acute exacerbation of chronic HF, an intra-aortic balloon pump (IABP) is inserted to effectively reduce the afterload and augment the diastolic pressure, improving the coronary perfusion. It is used to avoid impending hemodynamic collapse and subsequently bridge the patient to advanced therapies. On very rare occasions heart transplantation is considered in HF patients on extracorporeal circulatory support (Table 13.7). With recent developments in MCS, patients who are clinically deteriorating while waiting for a suitable donor organ can be safely transitioned to a left ventricular assist device (LVAD). Improved circulation should not only improve the physiological condition, but also enhance end-organ function. The number of patients with assist devices used as bridge to transplantation is on the rise and as per the 2013 ISHLT report, 37 % of the patients receiving HT had LVAD [1]. Patients with assist devices have diverse anesthetic considerations (Table 13.8) and may need invasive monitoring prior to induction. Sternal reentry plan should be discussed with the surgical service, especially if the outflow graft is close to the sternum. These patients are at increased risk of intraoperative bleeding secondary to dissection of dense mediastinal adhesions and preoperative anticoagulation. Coagulation profile is obtained on arrival and warfarin induced anticoagulation can be safely reversed with either fresh frozen plasma (FFP, 10–15 mL/kg) or pro-thrombin complex concentrates (PCC) . Vitamin K 10 mg is routinely administered intravenously once the decision is made to reverse the anticoagulation. FFP is associated with transfusion related risks and volume overload in these tenuous patients. PCC can reverse vitamin K antagonist anticoagulation rapidly and reliably [26, 27]. Dosing of PCC depends on presenting International Normalized Ratio (INR), and in spite of variability in dose response, INR can be reduced to 1.5 or below with a total dose of 10–30 units/kg. Appropriate response can be expected in 15 min and redosing can be considered if the INR is higher than 1.5. Right heart failure is a well-known complication after LVAD implantation; albeit lower incidence has been reported with newer generation pumps, it has significant implications [28, 29]. A failing right heart is sensitive to changes in loading conditions and these changes should be minimized prior to cardiopulmonary bypass. Controlled device-related infection is an important indication for transplantation and appropriate antibiotics should be continued perioperatively.
Table 13.7
Pretransplant mechanical circulatory support in recipients between 2006 and June 2011
Left ventricular assist device (LVAD) | 27.3 % |
Right ventricular assist device (RVAD) | 3.8 % |
Total artificial heart (TAH) | 0.9 % |
Intra aortic balloon pump (IABP) | 6.2 % |
Extracorporeal membrane oxygenation (ECMO) | 1 % |
Table 13.8
Considerations in LVAD patient with perioperative implications
1. Assure proper device functioning |
2. Anticoagulation status |
3. Acquired Von Willebrand disorder |
4. Appropriate intravascular volume prior to anesthetic induction |
5. Right heart function and positive pressure ventilation |
6. Associated infection and continue antimicrobials |
Cardiac Implantable Electronic Devices (CIED)
Significant numbers of advanced heart failure patients have cardiac resynchronization therapy (CRT) with or without cardioverter defibrillator (ICD). CRT reduces the morbidity and mortality in selected individuals (Left ventricular ejection fraction [LVEF] ≤35 % and QRS duration >120 ms) by optimizing the interventricular and intraventricular conduction [30–32]. ICD is often employed for both primary and secondary prevention in heart failure individuals [33]. Evidence for clinical utility of ICDs for primary prevention is more robust for HF from ischemic etiology than non-ischemic etiology [33, 34]. These Cardiac implantable electronic devices (CIED) need to be interrogated prior to surgery. ICD therapy function should be turned off and transcutaneous defibrillator pads be applied. All rate responsive functions and any enhancements on CIED are discontinued. Monopolar cautery during surgery should be employed in brief bursts to prevent inhibition of a pacemaker. The device should not be in the current pathway between the cautery applicator and return pad. If electromagnetic interference is resulting in hemodynamic compromise and patient is pacemaker dependent, CIED can be programmed to asynchronous mode. Placing central lines in these patients can be challenging at times with indwelling wires and sclerosis of the veins.
Right Heart Function and Preoperative Pulmonary Hemodynamics
Morphologically adult right ventricle (RV) with a wall thickness of less than 5 mm is designed to pump blood into low pressure pulmonary circulation and is relatively sensitive to afterload changes. It will adapt to chronic increase in pulmonary arterial hypertension (PHT) with increase in wall thickness and decrease in wall stress. But acute increase in pulmonary arterial pressure (PAP) is poorly tolerated by the RV [35] especially when exposed to ischemia reperfusion as in transplantation. Chen et al. [36] designed an experimental animal model to study the performance of the RV in a transplanted heart in the setting of pharmacologically induced chronic PHT . They found that RV could maintain the blood flow at increased energy expenditure and decreased efficiency. Brain death is also demonstrated to decrease the RV functional reserve against acute increase in afterload [37, 38]. It is not clear whether this detrimental effect can be improved or reversed by changes in donor management.
Pulmonary circulation in end stage HF is exposed to long-standing increased left atrial pressure resulting in passive venous congestion and secondary changes in pulmonary vasculature. This results in secondary PHT from both anatomical remodeling of the pulmonary vascular tree and endothelial dysfunction [39]. There is a gradual progression of PHT as the HF state worsens and increased PAP can be reactive to a variable degree. From early experience in 46 recipients, Kirklin et al. [40] identified the pulmonary vascular resistance (PVR) and pulmonary vascular resistance index (PVRI) as important predictors of early and late outcomes after HT. They found that the effect of PVR on risk of death was incremental with increasing PVR. Stanford experience [41] from 301 transplants between 1980 and 1988 identified that preoperative PVR of more than 2.5 Woods units (WU) increased the mortality rate within 90 days by more than twofold (17.9 % vs. 6.9 %). In their series, transpulmonary gradient (TPG) of 15 mmHg differentiated high-risk recipients for early postoperative mortality (90 days) from low risk recipients. They checked the responsiveness of pulmonary vasculature to sodium nitroprusside in patients with pulmonary artery systolic pressures (PASP) more than 40 mmHg. Patients who were responsive to nitroprusside as defined by decrease in PVR to less than 2.5 WU without inducing systemic hypotension (systolic arterial pressure less than 85 mmHg) were identified to have five to six times lower mortality than high risk group (6 % vs. 33.3 %). Murali et al. [42] retrospectively analyzed their HT cohort between 1980 and 1991 to identify preoperative TPG more than 15 mmHg (not PVR), recipient age, female sex, era of transplantation as the independent predictors of early post-transplant mortality. They postulated that TPG being a flow independent variable may be more predictive of outcomes after HT. The risk of RV failure will increase if the PASP exceeds 60 mmHg along with other aforementioned variables. Efforts should be directed towards decreasing the PAP by unloading the failing left ventricle (LV) in these patients. Failure of optimal medical therapy including inotropic support should be demonstrated by serial right heart catheterization before considering MCS such as IABP or LVAD [43]. Over the years, even though listing criteria has been proposed by the ISHLT, it is difficult to define a cut-off value for PAP above which HT is absolutely contraindicated. This can be because of improved understanding and treatment of RV physiology and failure.
Transpulmonary Gradient (TPG) = Mean pulmonary artery pressure (MPAP) – Pulmonary capillary wedge pressure (PCWP)
PVR in WU = TPG mmHg/Cardiac output (liters/min).
Vasodilator challenge after optimization of HF therapy, to define the reactiveness of the pulmonary vasculature, can help in identifying patients who are at high risk of complications after HT. If the PHTN is nonreactive or fixed, it may portend a worse prognosis after HT [41]. If PVR can be reduced to <2.5 WU without systemic hypotension, post-HT outcomes are comparable to patients without PHT [41, 44]. In contemporary practice, common vasodilators used in the vasodilator challenge are nitric oxide, prostaglandin, sodium nitroprusside, and sildenafil. The protocol and hemodynamic end points for clinical decision-making vary from one institution to another. Advances in assist device technology and patient management have resulted in long-term outcome of patients with assist devices rivaling the transplant results. This opens up another avenue for patients with fixed PHT to receive transplant if there is demonstrable decrement in PAP after MCS.
Anesthetic Management
HT almost always is performed as an emergency procedure as it depends on the availability of a donor organ. Though these patients undergo a thorough evaluation and preparation there is usually a time interval between the evaluation and the actual surgery. Therefore, careful preoperative exam is essential to manage these patients and full stomach precautions are employed if the fasting status is not desirable. These patients can have varied level of hemodynamic stability depending on the level of heart failure severity. Ambulatory patients will be on multiple medications to alter the loading conditions and inotropy favorably to alleviate the congestive state. Patients in higher INTERMACS levels may be on inotropic infusions and may also be on mechanical assist devices like IABP or extracorporeal membrane oxygenation (ECMO) . Historically LVAD recipients receive 30 days elective period of status 1A after the initial peri-implantation period. This practice has been questioned recently as the technology and clinical outcome of these patients has improved substantially over the years introducing disparity in severity of status 1A patients [45].
Close communication between the transplant coordinator, the team harvesting the donor heart and the team preparing the recipient remains vital to minimize the ischemic time of the donor organ. The recipient should be on cardiopulmonary bypass (CPB) , with the recipient heart dissected when the donor heart arrives; however, induction of the recipient and incision should not take place until the harvesting team is in the operating room and has the opportunity to actually examine the donor heart to be certain it is suitable.
Anesthetic Induction
It is now well appreciated that the failing heart is preload dependent and afterload sensitive, and these patients do not tolerate even the most trivial perturbations in these and other parameters such as heart rate, rhythm and contractility that the anesthesiologist is well versed in manipulating. There is no evidence that any particular anesthetic agent is preferable in HF patients. It is important to recognize the physiological changes these agents induce and identify these changes with sensitive monitoring. Invasive monitoring such as arterial line and PAC are placed pre-induction to facilitate making right interventional decisions during hemodynamic deterioration. If the invasive monitoring is placed preoperatively to optimize the HF status, consideration should be given to change the invasive lines prior to sternotomy, to decrease the possibility of line-acquired blood stream infection in immunosuppressed patients. The anesthesiologist and the surgical team should be ready to escalate the circulatory support in the event of hemodynamic compromise with anesthetic induction. Spontaneous inhalational induction offers an advantage of minimizing rapid decrease in preload, which is not tolerated well in HF patients, especially those with RV dysfunction. In emergency situations, and if patient is at risk of pulmonary aspiration, rapid sequence induction is performed. With the diminished cardiopulmonary reserves in end stage HF, efforts should be directed towards preserving these compensatory mechanisms. Sympatholysis with high-dose opioids is avoided unless somebody is tolerant to opioids. Post intubation ventilator parameters are adjusted to prevent hypercarbia and optimize oxygenation in order to minimize the effect on pulmonary vascular resistance. In patients on preoperative inotropes, it is important to continue these infusions during the induction phase of the anesthesia. It may also be necessary to start an infusion of vasopressors, typically norepinephrine to offset the effects of the general anesthesia and all the induction medications.
Immunosuppression in the Perioperative Period
The goal of optimal immunosuppression is to maintain native host immune reactivity suppression in order to prevent rejection of the graft and balance the risk of side-effects, including increased risk of opportunistic infection from over-immunosuppression (Table 13.9). This can be achieved by closely monitoring for adverse effects and measuring blood levels of immunosuppressive drugs. Corticosteroids (CS) are weaned if tolerated and it is observed that the use of CS declines but the use of proliferation signal inhibitors (PSI) doubles between 1 and 5 years after HT [1]. With the available evidence, it is not possible to advocate a preferred combination of drugs for immunosuppression following heart transplantation. The selection is essentially driven by the side effect profile in the given patient and organ function. Higher incidence of acute rejection in the early postoperative period has led to the practice of inducing intense immunosuppression during the early perioperative period (Table 13.10). According to 2013 ISHLT report, this practice of empirical induction therapy has decreased in 2012, with overall 47 % during the first 6 months of 2012 [3]. Induction of immunosuppression is usually achieved by monoclonal or polyclonal antibodies. Decision to utilize induction therapy, essentially depends on institutional practice as there is no conclusive evidence demonstrating their utility (Table 13.11) [48]. They have been associated with adverse effects such as infection, prolonged leukopenia, and risk of malignancy [49]. Prior to induction therapy with antibodies, patients are routinely premedicated with corticosteroids, antihistamines, and antipyretics.
Table 13.9
Applied pharmacology of common immunosuppressants during perioperative course of heart transplantation
Class | Medications | Mechanism of action | Adverse effects |
---|---|---|---|
Corticosteroids (CS) | Methylprednisone Prednisone | Alter gene transcriptional regulation with suppressed inflammatory and immune response of WBCs | Psychiatric effects, poor wound healing, hypertension, adrenal suppression |
Calcineurin inhibitors (CNI) | Cyclosporine Tacrolimus | Inhibits transcription of cytokines (IL-2) involved in immune response | Hypertension, renal insufficiency, neurologic toxicity, dyslipidemia, hyperglycemia |
Antimetabolites | Azathioprine Mycophenolate mofetil | Interferes with cell cycle regulation | Myelosuppression, nausea/vomiting, diarrhea |
Proliferation signal inhibitors (PSI) | Sirolimus (FDA approved for OHT) | Inhibits a kinase (TOR) controlling the proliferation of lymphocytes | Renal dysfunction, impaired wound healing, diarrhea, myelosuppression |
Table 13.10
Immunosuppression induction agents prior to heart transplantation
Monoclonal Antibodies—28 % of heart transplants | |
CD25 antagonists (IL-2 receptor on T lymphocytes) | Basiliximab (Simulect) Daclizumab |
CD52 binding (T and B lymphocytes) | Alemtuzumab |
Polyclonal Antibodies—11 % of heart transplants Rabbit anti-thymocyte globulin (RATG) Horse anti-thymocyte globulin (HATG) |
Table 13.11
Proposed indications of induction therapy
1. Decreased risk of acute rejection in allosensitized recipients |
2. Rapid induction of immunosuppression during rejection |
3. Delay the initiation of CNIs in patients with renal insufficiency |
4. Permit delay in introduction of CNIs |
5. Facilitate regimens with low-dose steroids |
6. Provides flexibility for corticosteroid weaning |
Perioperative Implications of Allosensitization
Allosensitization is defined as development of antibodies to human leukocytic antigen (HLA) molecules secondary to a sensitizing event. These antibodies were initially recognized to be responsible for poor renal allograft function and rejection in 1969 [50]. HF patients are often exposed to these during their disease course. Common sensitizing events are identified in the Table 13.12. It is important to recognize that even leukocyte reduced red blood cell (RBC) transfusion can result in sensitization and red cells also present major histocompatibility complex (MHC) class 1 antigens on their cell membrane, albeit in lower concentrations than lymphocytes [51]. Allosensitization is important because of its relevance in adverse outcomes after organ transplantation. Patients with immunological sensitization as measured by panel reactive antibodies (PRA) tend to have a worse outcome after HT than those without sensitization [52, 53]. It is routine in current practice to check serial PRAs on potential recipients to identify development of sensitization. Antibodies tend to disappear after the initial triggering event without further exposure. Antibodies formed after transfusions disappear in 5–11 months after the trigger. Multiple triggering events can induce broader sensitization as measured by sensitive cytotoxic methods [54]. The broader sensitization will decrease the available donor pool, resulting in longer waiting period for HT.
Table 13.12
Risk factors for allosensitization
1. Blood and blood product transfusion |
2. Previous allografts |
3. Pregnancy |
4. Prior cardiac surgery with allografts |
5. Cardiac assist devices |
6. Hematological malignancies |
MCS especially LVAD are increasingly used more to treat end stage HF patients on transplant waiting list [3]. It is well known now that these devices can induce immune sensitization independently. In spite of modern rotary pumps not having bioprosthetic material in their design, there is evidence of allosensitization albeit to a lower degree than older pulsatile pumps [55]. Risk of sensitization appears to be higher in the first 3 months after implantation [56]. Pre-sensitized patients may be at risk of broader sensitization after implantation of pulsatile flow devices. Retrospective analysis of patients transplanted on assist device from United Network for Organ Sharing (UNOS) database between 2004 and 2009 revealed a longer waiting time for those with PRA > 10 % (205 days [interquartile range, 81–344] vs. 124 days [interquartile range, 51–270]) [57]. Notably, alloimmunization after assist devices has not been shown to result in poor survival or translate into higher rejection rates after HT [55, 57, 58]. This brings up the question of functional relevance of these antibodies after device implantation. Advances in immunological testing and clinical experience with these devices should shed some light on this question.
This immunological sensitization is identified by screening the recipients for anti-HLA antibodies directed at set of panel antigens of random donors (e.g., PRA). The threshold to define significant sensitization is still debatable and varies from PRA level of 10–25 % [53, 59]. The UNOS online calculator can be used to define the calculated PRA (cPRA), which signifies the percentage of the general donor pool against what recipient demonstrates antibodies. Once allosensitization is identified by PRA level, specificity of these antibodies are further improved by identifying antibodies against specific human HLA molecules. Modern solid-phase assays can identify antibodies against both classes of MHC antigens and their binding strengths. This will enable the transplant team to confirm donor specific antibodies (DSA) when there is an offer, by matching against antigens identified by donor tissue typing. This process is termed virtual cross-matching (VxM) and is widely used in confirming the immunological compatibility of donor organs [60]. Stehlik and colleagues studied the utility of VxM in HT by comparing it with prospective cross match, and in their series of 14 patients VxM had a negative predictive value of 92 % and positive predictive value of 79 % [61]. It helps in defining the functional relevance of the identified antibodies and accepting donors without the need for a prospective cross match [62]. This should help in accepting organs from a wider geographical zone [63]. Retrospective cross-matching is performed in all sensitized recipients to guide the management of immunosuppression. Aggressive immunosuppression with close surveillance for rejection may be required in those with positive retrospective cross-match results.
Some recipients are very highly sensitized, and preoperative management of these patients is challenging considering balancing the risk of graft failure versus death on the waiting list. Therapeutic immunomodulatory strategies to alleviate this immunological disadvantageous state by desensitization techniques using plasmapheresis, IV Immunoglobulin (IVIG), monoclonal antibodies, or proteasome inhibitors have been advocated by certain transplant centers [64, 65]. It has yet to be proven that these strategies have predictable success. Moreover, the effectiveness of these strategies is negatively affected by serious complications like infections and morbidity associated with these medications. According to the survey conducted across multiple transplant centers, 8 % of the transplant patients underwent desensitization therapy prior to HT [66]. There is lack of evidence to support desensitization therapy in this fragile group of patients over watchful avoidance of mismatch donors using modern immunological methods.
Intraoperative Transesophageal Echocardiography (TEE)
TEE is an essential intraoperative monitor, and history should be sought to rule out any contraindications before insertion of the esophageal probe. Intraoperative TEE is ideally suited to identify acute complications during cardiac transplantation and titrate the hemodynamic support to the cardiac function especially in the post-implantation stage [67, 68] (Table 13.13).
Table 13.13
Utility of TEE during orthotopic heart transplantation
Preoperative period |
1. Ventricular function |
2. Rule out intracavitary thrombus |
3. Significant aortic atherosclerotic disease |
4. Assist in placement of cannulae for CPB |
Post-CPB |
1. Ventricular and valvular function |
2. De-airing of the graft |
3. Rule out intracardiac shunt |
4. Anastomotic complications |
5. Assist in placement of cannulae for mechanical circulatory support |
6. Confirm IABP position |
7. Rule out aortic dissection post decannulation |
Conduct of Cardiopulmonary Bypass and Surgical Techniques
With more than 99,000 adult HT performed worldwide, surgical technique of implantation has been refined, and there is gradual adoption of the bicaval technique to implant the graft [69]. Original Lower and Shumway technique involves bilateral atrial anastomosis, with retention of some native atrium [70]. In the early 1990s, the bicaval technique was described in two different clinical series, with complete excision of native atrium and direct anastomosis of superior and inferior vena cava [71, 72]. Preserving normal atrial anatomy and function was the objective of this technique. Potential advantages of bicaval technique includes decrease in:
- 1.
Atrial dysfunction
- 2.
Sinus nodal dysfunction
- 3.
Valvular insufficiency
- 4.
Thrombus formation
Longer surgical time can be a disadvantage with bicaval technique with a possibility of prolonging graft ischemic time. Although many single center studies have described decreased complications (atrial arrhythmias, tricuspid regurgitation, nodal dysfunction) immediate post transplantation with bicaval technique [73–76], evidence for long-term survival benefit is not clear [77]. A retrospective UNOS data analysis of 11,931 primary HT between 1999 and 2005 found no difference in survival between matched groups with bi-atrial vs. bicaval techniques [78]. Nonetheless, the bicaval technique was associated with lower permanent pacemaker (PPM) implantation and shorter hospital length of stay. Another multivariate analysis of UNOS database [79], over 10 years (1997–2007) demonstrated small but significant survival advantage of the bicaval technique and improved PPM free period. Authors attributed this discrepancy to the longer period of analysis, the increase in bicaval technique, and difference in statistical methods.
Anticoagulation for cardiopulmonary bypass (CPB) is achieved by unfractionated heparin 350–450 units/kg, unless contraindicated, to achieve an activated clotting time (ACT) above 480 prior to proceeding on CPB. Despite, the lack of correlation between ACT and heparin levels, ACT remains a reliable and safe monitoring technique in managing heparin-based anticoagulation for CPB. Certain physiological changes (hemodilution, hypothermia) and pathological conditions (conditions with release of inflammatory mediators, protein S resistant states) can make ACT monitoring less reliable. If these clinical circumstances are suspected, monitoring methods using Heparin Dose Response (HDR) can be used to maintain certain plasma concentration of heparin.
If the patient is diagnosed with heparin induced thrombocytopenia (HIT) and the anti-PF4 antibody titers were elevated within 100 days, anticoagulation is typically achieved by direct a thrombin inhibitor such as bivalirudin (Table 13.14). Risk of bleeding increases with bivalirudin as the anticoagulation is not reversible. This is especially true in patients with renal insufficiency. Heparin can be used for CPB only in somebody with remote history of HIT, as the anti PF4 antibodies decrease to clinically insignificant levels in 3 months of non-exposure. It is important for all the personnel involved in patient care to understand that this patient with remote history cannot be reexposed to heparin after CPB and all precautions should be taken to prevent reexposure (Table 13.15).
Table 13.14
Recommended bivalirudin dosing and management plan for cardiac surgery on cardiopulmonary bypass
Dose prior to CPB | Dose during CPB | Dose after CPB | |
---|---|---|---|
PATIENT | 1.0 mg/kg IV bolus followed by 2.5 mg/kg/h IV infusion ACT >4 times the baseline | • 2.5 mg/kg/h IV infusion • Stop infusion 15 min prior to anticipated CPB discontinuation • If not possible to separate from CPB and decannulate in 20 min rebolus 0.5 mg/kg and restart infusion at 2.5 mg/kg/h | None |
Flush solutions | 0.1 mg/mL bivalirudin | Same as dose prior to CPB
![]() Stay updated, free articles. Join our Telegram channel![]() Full access? Get Clinical Tree![]() ![]() ![]() |