Fig. 5.1
Pathophysiologic changes during brain death. BBB blood brain barrier, DI diabetes insipidus, DIC disseminated intravascular coagulopathy, ICP intracranial pressure
First Phase: Peri-Death
Just prior to the development of brain death, it is very common to see the early development of hemodynamic instability and metabolic disruptions. The primary insult that progress to brain death often leads to cellular ischemia, disruption of the blood brain barrier, and release of cytokines resulting in a systemic inflammatory response. Systemically this could manifest early as vasodilatory shock.
Second Phase: Brain Stem Death
Once the elevated intracranial pressure (ICP) induces cerebral hypoperfusion, a vicious cycle ensues of global brain ischemia potentiating more edema, further compromising of blood flow until cessation of circulation occurs. In the last stages of this process, brain stem herniation results from mass effect and brain stem death. This occurs in a rostrocaudal direction. Pontine ischemia occurs first resulting in the classic “Cushing’s response ” from a mixed vagal and intense sympathetic stimulation causing bradycardia, hypertension, and an apneustic-breathing pattern. Medullary ischemia follows which is the beginning of the hypertensive and extensive systemic vasoconstrictive process given the unopposed sympathetic stimuli. This is followed by a dramatic release of catecholamines [3, 4]. Tissue hypoperfusion can develop given the systemic vasoconstriction. Finally, a sudden decrease in systemic vascular resistance manifesting as vasodilatory shock is the final step resulting in loss of spinal sympathetic pathways. The anterior and posterior pituitary gland impairment is also manifested at this stage.
Third Phase: Pathophysiologic and Homeostatic Changes After Brain Death
Hemodynamics
A complex pattern of hemodynamic changes can occur, which can rapidly progress to cardiac arrest, if untreated. Not all changes; however, are seen with every donor and factors associated with etiology of brain death, time course of the process of death and duration of support after brain death can alter the hemodynamic responses.
Cardiac Dysfunction
Cardiac dysfunction occurs after brain death due to a combination of catecholamine-mediated subendocardial ischemia during the sympathetic surge; increased intracellular calcium concentration inducing oxygen-free radicals; myocardial gene expression; and thyroid hormone depletion. Eight-nine percent of patients with no preexisting heart disease have been found to develop cardiac dysfunction following brain death [5, 6]. The role of thyroid hormone depletion remains an area of controversy; however, rapid loss of free triiodothyronine seen after brain death has been associated with depletion of ATP, increased anaerobic metabolism, and impaired cardiac contractility.
Vasomotor Tone
Loss of spinal sympathetic tone, cytokine, and inflammatory mediator release, and cortisol deficiency contribute to vasodilatory shock following brain stem death. Circulating cytokines and inflammatory mediators are released through three mechanisms: the sympathetic outflow surge during medullary death, necrotic brain tissue and ischemia-reperfusion injury from the hypertensive-vasoconstrictive crisis followed by a loss of vasomotor tone [7–9]. Brain death has been associated with more than 100-fold increased in concentrations of IL-6 and other inflammatory markers such as TNF and IL-10. Higher concentrations of IL-6 just prior to organ procurement has been associated with lower number of organs procured for transplantation and lower six-month hospital-free survival in recipients [10].
In addition, recruitment of leukocytes, oxygen-free radicals, and capillary leak are seen [11]. Central cortisol deficiency that results from loss of adrenocroticotropic hormone release can further potentiate the loss of vasomotor tone through down-regulation of catecholamine receptors.
Volume Status
Regardless of blood pressure, patients are often volume depleted. Unrecognized intravascular volume depletion due to factors before death is not uncommon and is often not manifested until loss of vasomotor tone. Blood loss associated with trauma, hyperosmotic therapy such as mannitol and third spacing of fluid in the setting of inflammatory mediators can all contribute to volume depletion. This is further exacerbated by the development of diabetes insipidus with loss of the posterior pituitary function and is seen in over 80 % of brain dead patients [3]. To further exacerbate this, hyperglycemia (from insulin deficiency or attempts to correct hypernatremia with a dextrose solution) can also result in a free water diuresis. Careful attention to volume status during donor management is crucial.
Hormonal Changes
Anterior and posterior pituitary failure renders the clinical picture susceptible to further imbalances that potentiate instability. The degree of hormonal deficiency present has been a significant area of controversy and while largely supported in animal studies, has not consistently been seen in human studies [12–17].
Cortisol
A state of cortisol deficiency occurs after loss of release of adrenocorticotropic hormone from the anterior pituitary. Impairment in the donor’s stress response and sensitivity to catecholamines ensues following brain death. Activated inflammation leads to pro-inflammatory mediators and expression of their receptors on organs which make them more susceptible to immune-mediated injury [18] putting them at higher risk of acute rejection compared to living donors. Modulation of the immune response at the level of the donor is an area of much interest [19–23].
Antidiuretic Hormone
Diabetes insipidus occurs in over 80 % of brain dead donors [24]. The resulting inappropriate diuresis, hyperosmolality, and hypernatremia can affect the viability of potential organs. If the etiology of the shock is unrecognized, aggressive resuscitation with normal saline has the potential to worsen the hypernatremic state.
Thyroid Hormone
Impaired inotropy, depletion of ATP, and the development of lactic acidosis may stem from a rapid drop in free triiodothyronine levels. Impaired secretion of thyroid stimulating hormone and loss of peripheral conversion is thought to be the mechanism for depletion of free triiodothyronine.
Insulin
A drop in insulin secretion results in systemic hyperglycemia, intracellular hypoglycemia, and a resulting energy deficit. This can result in a transition to anaerobic metabolism, further exacerbating a lactic acidosis that may be present from tissue hypoperfusion and thyroid depletion.
Coagulopathy
Disseminated intravascular coagulopathy and platelet dysfunction are common causes of coagulopathy seen after brain death. Necrotic tissue induces the release of thromboplastin and plasminogen activator leading to DIC in 28 % of donors [25]. In addition, microthrombus formation can be seen from stasis resulting from vasoconstriction during the sympathetic surge.
Temperature Regulation
Given the central role of hypothalamus in temperature regulation, this process is disrupted following brain death. In addition, the loss of muscular activity leads to an impairment in heat production and this is process is exacerbated by the vasodilated state that ensures following spinal sympathetic outflow cessation.
Donor Management
The most significant principle behind donor management is that best practice ICU care continues beyond brain death including resuscitation end points, lung protective ventilation, and preventative infectious measures. Physicians must be cognoscenti of the fact that the care and interventions they provide during the donor management will carry over to impact graft function following transplantation.
Hemodynamics
Arterial hypertension occurs early during brain ischemia but is often self limited. Treatment with short acting agents such as sodium nitroprusside or esmolol are recommended in these cases [26] to keep systolic blood pressure <160 mmHg and mean arterial pressure <90 mmHg.
Fluid
When hemodynamic instability develops, fluid repletion is the first step to reverse tissue hypoperfusion as more than 80 % of brain-dead organ donors have shock. Careful attention to the development of diabetes insipidus and appropriate early management is crucial before a significant diuresis and hypovolemia ensues. Specific end points of fluid resuscitation include a MAP >65 mmHg; a systolic blood pressure >90 mmHg; heart rate of 60–120 min−1; urine output of at least 0.5 cc/kg/h; a central venous pressure of 8–12 cm H2O and hemoglobin of at least 10 g/dl (or hematocrit of 30) [26–28]. Early serial lactate monitoring is also recommended to evaluate success of resuscitative efforts [26, 29]. One observational study found that inadequate resuscitation of donors was associated with increased inflammatory response (IL-6 and TNF) and loss of organs for transplantation [30].
In donors with refractory hypotension or low ejection fraction more hemodynamic monitoring is recommended. Traditionally monitoring has been performed using a pulmonary artery catheter; however, given the limitations associated with the use of central venous pressure and pulmonary artery occlusion pressures to predict preload responsiveness and due to increasing availability of less invasive strategies, clinicians are moving away from placing pulmonary artery catheters to determine fluid responsiveness [31, 32]. More sensitive parameters of fluid responsiveness such as pulse pressure variability (PPV), stroke volume variability (SVV), systolic pressure variability, extravascular lung water measurements and transpulmonary thermodilution and ultrasound assessment of inferior vena cava are all minimally invasive or noninvasive novel techniques that are increasingly being used in organ donor management.
A challenge in donor management, particularly for the multiorgan donor, stems from the fact that different organ systems have different desirable targets for fluid status with the lungs preferring a more intravascularly deplete environment; while the kidneys require more judicious fluid administration (see Table 5.1 for organ specific considerations). Use of highly sensitive and specific measures of fluid responsiveness such as PPV and SVV helps with precise volume control in multiorgan donors. A large randomized control trial is underway to examine whether protocolized donor care using PPV, CI, and MAP, targets are associated with increased organ recovery from brain dead donors (MOnIToR Trial) [33].
Table 5.1
Organ-specific considerations
Lung transplant | Judicious fluid administration Minimization of ventilator associated lung injury via lung protective ventilation Protocolized donor management strategies Consideration of ex-vivo lung perfusion for marginal lungs |
Heart transplant | Serial echocardiograms if initial demonstrates impairment Minimization of high-dose beta agonists (leads to down regulation of beta receptors) |
Liver transplant | Avoid excessive increase in central venous pressure (congestion) Avoid hypernatremia (aim Na <150 meq) |
Kidney transplant | Euvolemia Avoid nephrotoxic insults (medications, contrast if can avoid or adequately hydrate) Lowest dose vasopressors |
Traditionally during a period of instability and increased oxygen demand, a red cell transfusion to achieve hematocrit >30 % or hemoglobin >10 g/dl is recommended [34]; however, in more recent years after extrapolation from other transfusion trials in the intensive care settings and the inflammatory risks associated with older blood, more conservative thresholds are recommended (hemoglobin of 7–9 g/dl) [29, 35, 36].
Use of hydroxyethyl starches for fluid resuscitation in organ donors has been associated with increased risk of acute kidney injury, renal replacement therapy, and delayed graft function and thus should be avoided [37, 38]. While initial favorable experiences were reported with their use for liver transplant leading to less fluid administration, no outcomes of number of organs procured or organ function were reported in its initial evaluation [39]. While the use of albumin makes sense physiologically, particularly in the case of significant volume resuscitation, no good evidence to this date supports its use as initial choice for resuscitation. After significant resuscitation with crystalloid, if a patient proves to have ongoing fluid requirements, it is not unreasonable to consider albumin in the setting of low serum albumin levels [40].
Inotropes and Vasopressors
Often fluid resuscitation is inadequate to meet hemodynamic and tissue perfusion goals and therefore vasopressors and or inotropes have become the mainstay of therapy. The critical care community has been moving away from using dopamine as first line agent given the side effect profile including significant arrhythmias [41]. However, in potential kidney transplant donors, the use of low-dose dopamine was associated with the reduced need for dialysis after transplant [42]. For pure vasodilatory shock, we recommend arginine vasopressin as first line given its dual impact of blunting diabetes insipidus as well as its catecholamine sparing action by targeting vasopressin receptors. Low-dose vasopressin (<2.5 units/h) is not only sufficient to raise the mean arterial pressure but has also been found to be associated with favorable kidney, liver, and heart graft function [43]. A recent retrospective review evaluating patients managed with vasopressin compared to its absence was associated with increased graft recovery rates in the vasopressin group [44]. Finally, one study comparing vasopressin to epinephrine, demonstrated a higher incidence of cardiac arrest amongst brain dead donors in the epinephrine group compared to vasopressin [45].
Norepinephrine, epinephrine, or phenylephrine are all reasonable second agents of choices depending upon whether the predominant feature is vasodilatory shock or whether there is a lower cardiac contractility. Caution must be exercised with excessive beta agonist therapies as they lead to a down-regulation of beta receptors which has the potential to impair cardiac contractility in the recipient [27].
Regarding inotropic support, dobutamine or milrinone can be used; however, given the high likelihood of component volume depletion or the potential to develop or likely concurrent drop in the systemic vascular resistance, caution must be exercised. In shock due to impaired cardiac contractility and vasodilation, norepinephine with dobutamine or epinephrine may be reasonable choices. Avoiding or minimizing the time exposure to high dose inotropic support, if at all possible, should be strived for given its association with moderate to severe myocardial injury in heart donors [46].
Hormonal Therapy
There has been substantial debate surrounding whether hormonal dysfunction occurs during brain death, its impact on hemodynamics and whether exogenous replacement is beneficial. The largest review to date involved a retrospective analysis of hormonal resuscitation of brain dead donors that demonstrated a greater yield in suitable organs; however, results from subsequent studies and meta-analysis call into question their role [47, 48].
Cortisol
The potential role of cortisol replacement is twofold in donor management: blunting the inflammatory response and for hemodynamic support. The pro-inflammatory setting that ensues following brain death has the potential to render the organs to ischemia reperfusion injury and increased risk for allograft rejection. Early methylprednisone administration (15 mg/kg) is recommended to enhance hemodynamic stability and enhance organ function post transplant. Lower administration of steroids doses from 15 mg/kg of methylprednisone compared to hydrocortisone 300 mg has been evaluated and has been found to be noninferior with regard to pulmonary or cardiac function. In addition, less hyperglycemia was noted [49].
Antidiuretic Hormone
Diabetes insipidus is present in the majority of brain dead donors and if left untreated can induce profound hypotension and hypernatremia. Desmopressin or vasopressin can be administered to reverse the adverse effects of DI and often both agents are needed [43, 50]. Titrating the agents to a goal of 0.5–3 cc/kg/h is desirable.
Thyroid Replacement
Traditionally levothyroxine or triiodothyronine has been recommended in unstable donors, in particular for cardiac support. Conflicting results have called into question their central role in donor management. In a recent meta-analysis evaluating any randomized placebo controlled trials, the role for routine administration was not supported as no significant effect on cardiac index was noted in the pooled analysis. The major limitation of this study lies in the fact that there was only a small proportion of patients who were hemodynamically unstable. One could postulate that this underrepresented population are those ones who could have retrieved the greatest benefit [51]. A large randomized controlled trial that was recently published evaluated the role of oral vs. intravenous thyroid replacement in brain dead donors (NCT00238030, Sharpe MD). The recently published results have shown that orally administered T4 is well absorbed and achieves a bioavailability of approximately 91–93 % of intravenous T4 in organ donors. Inotropic/vasopressor requirements and hemodynamic responses following oral or intravenous thyroxine administration were comparable.
Hyperglycemia
Insulin administration is currently the standard of care for brain dead donors in the setting of hyperglycemia. Recommended targets vary from institution to institution with some that are more intensive than other (<180 mg/dl vs. <108 mg/dl) given the results of the more recent critical care trial evaluating intensive insulin therapy [52]. This study, which evaluated intensive vs. conservative insulin protocol found that the intensive arm was associated with a higher mortality. Interestingly; however, the primary cause of death was cardiovascular suggesting a relationship between intensive insulin therapy and cardiovascular harm. The results of a randomized controlled trial of intensive vs. conservative insulin therapy for brain dead donors to improve renal allograft function is currently underway and could better inform the optimal threshold for the care of the brain dead donor (NCT01140035, Niemann C).
Coagulopathy
To date, no directive evidence based guidelines exist on coagulopathy management specific to donor management; however, some centers aim for a platelet count of >50,000 μ1−1 and an international normalized ratio <1.5. Administration of platelets and fresh frozen plasma may be warranted prior to retrieval. Alternatively, other centers will only transfuse to reverse coagulopathy in the setting of hemorrhage given the risks associated with transfusion [26, 53].
Hypothermia
While hypothermia has the potential to be associated with adverse hemodynamic consequences including arrhythmias, myocardial suppression, cold-induced diuresis and potassium shifts, mild hypothermia may have a beneficial effect on organ preservation in the pre-retrieval phase and pilot trials are underway to examine the feasibility of therapeutic hypothermia as an-in-vivo organ preservation strategy initiated soon after brain death (NCT01680744, Niemann, C).
Ventilatory Management
Ventilatory management for the brain dead donor has undergone significant evolution over the past few years as there had been a lag in the adoption of lung protective ventilatory strategies in the management of the multiorgan donor. A landmark trial by Mascia and colleagues in 2010 demonstrated a significant increase in the number of lung eligible for donation after employment of a lung protective strategy. They ventilated brain dead donors with 6–8 cc/kg, performed recruitment maneuvers after any disconnection from the ventilator, performed the apnea test on continuous positive airway pressure, and used higher positive end expiratory pressures (PEEP) over 6 h. This was compared to standard ventilatory management with tidal volumes in the range of 10–12 cc/kg. Low tidal volume and high PEEP resulted in a significant increase in donor lung eligibility and procurement [1]. The major limitations of this study; however, included that it remains unclear which particular intervention in their strategy has the greatest impact and the implication for retrieval of other organs other than lungs. If we were to extrapolate from studies of ventilator associated lung injury and its potential to worsen end organ function, one could postulate that this strategy would have the potential to improve all organs (Fig. 5.2).
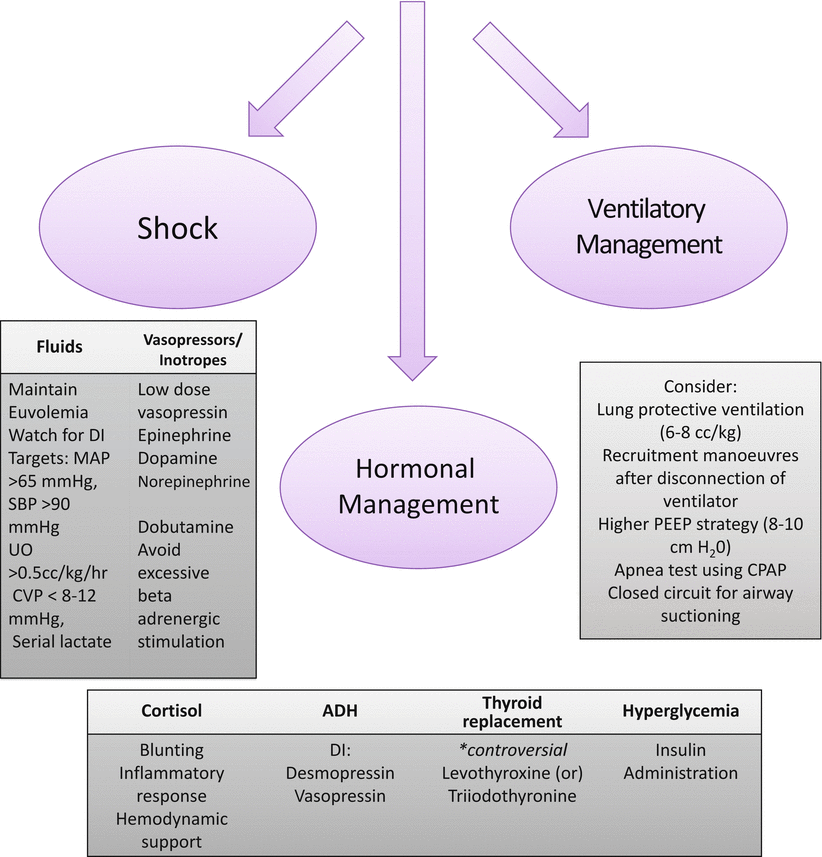
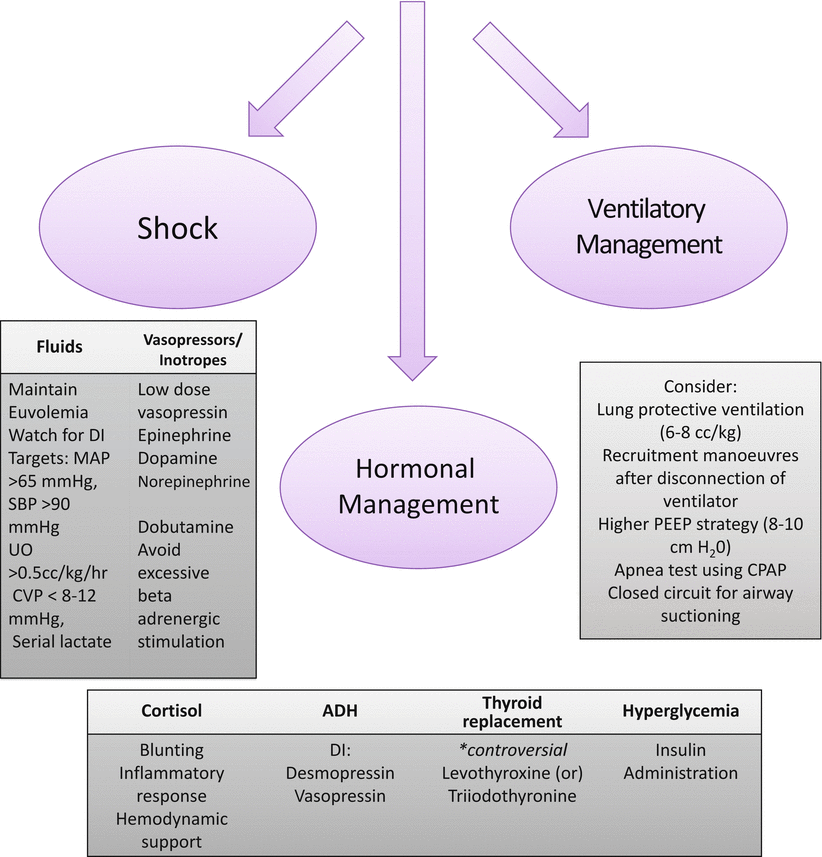
Fig. 5.2
Donor management
Organ-Specific Considerations
Lungs
Unfortunately , lungs are the most fragile organs in the multiorgan donor and most susceptible to the hostile environment of the brain dead donor. Less than 10 % of lungs are procured for transplantation. Therefore, meticulous attention to prevent iatrogenic lung injury is crucial to increase lung procurement. Fluid administration and atelectasis are two crucial reversible factors that have the potential to impair lung function. Previous studies have demonstrated that maintaining a more restrictive fluid balance, whether it be by titrating fluids to a more conservative central venous pressure measurement or extravascular lung water threshold, improve the number of organs that are eligible [43, 54].
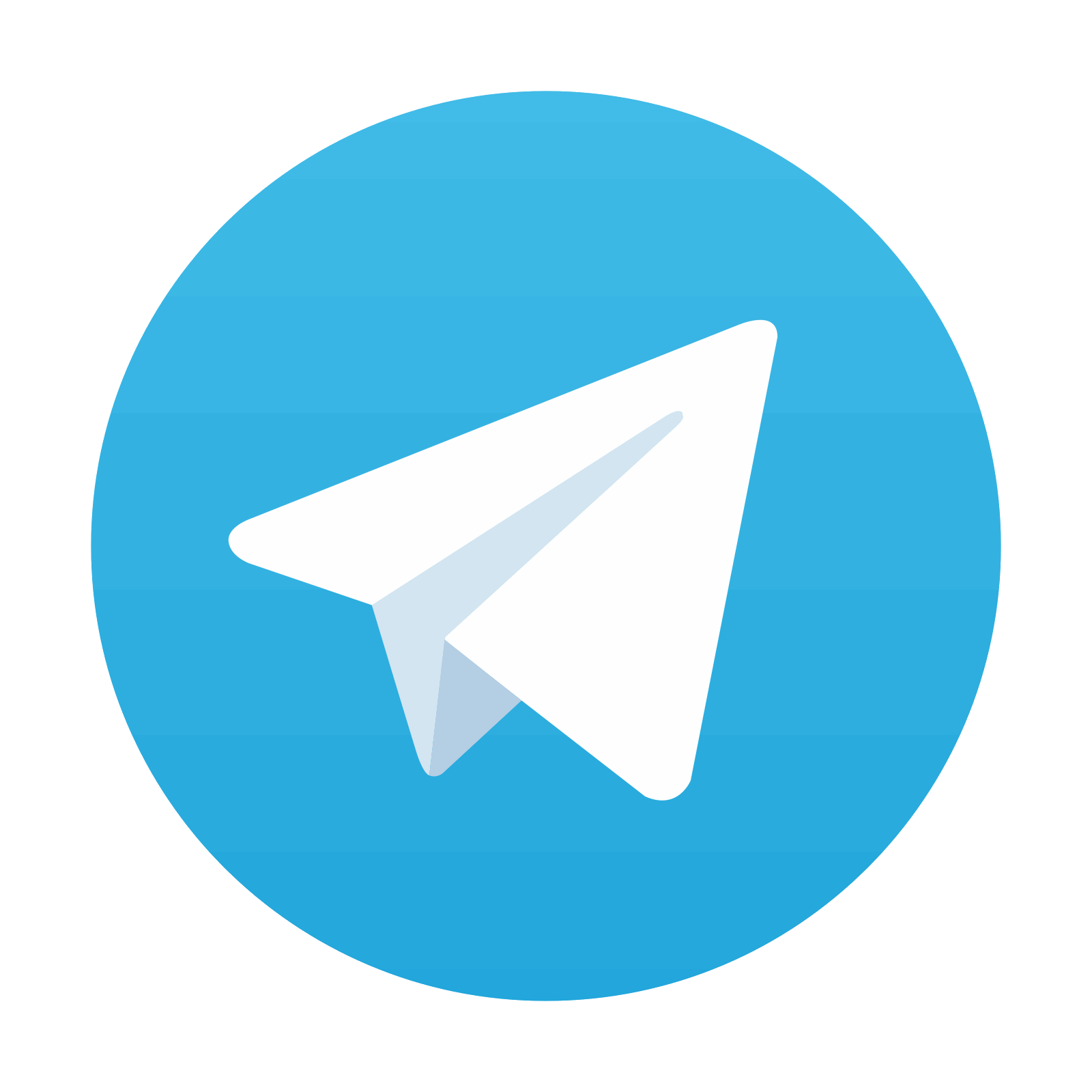
Stay updated, free articles. Join our Telegram channel

Full access? Get Clinical Tree
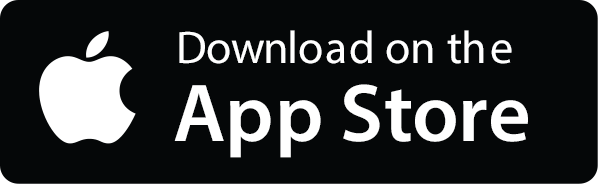
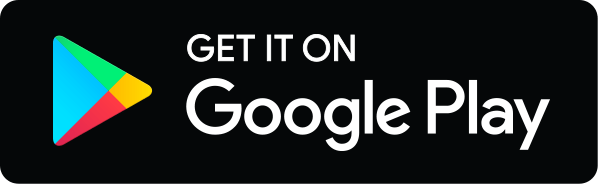