Key Concepts
All trauma patients should be presumed to have “full” stomachs and an increased risk for pulmonary aspiration of gastric contents.
Cervical spine injury is presumed in any trauma patient complaining of neck pain, or with any significant head injury, neurological signs or symptoms suggestive of cervical spine injury, or intoxication or loss of consciousness.
In the multiple-injury patient, providers should maintain a high level of suspicion for pulmonary injury that could evolve into a tension pneumothorax when mechanical ventilation is initiated.
In up to 25% of major trauma patients, trauma-induced coagulopathy is present shortly after injury and before any resuscitative efforts have been initiated.
Administering blood products in equal ratios early in resuscitation has become an accepted approach to correction of trauma-induced coagulopathy. This balanced approach to transfusion, 1:1:1 (red blood cell:fresh frozen plasma:platelet), is termed damage control resuscitation.
Noninfectious transfusion reactions are now the leading complication of transfusion and represent a more than 10-fold greater risk than blood-borne infection. Transfusion-related acute lung injury is the leading cause of transfusion-related death.
The assessment of blood consumption (ABC) score is an attempt to predict which patients are likely to require a massive transfusion protocol. The ABC score assigns 1 point for the presence of each of four possible variables: (1) penetrating injury; (2) systolic blood pressure less than 90 mmHg; (3) heart rate greater than 120 beats per minute; and (4) positive results of a focused assessment with sonography for trauma evaluation. Patients with ABC scores of 2 or higher are likely to require massive transfusion.
Any trauma patient with altered level of consciousness must be considered to have a traumatic brain injury (TBI) until proven otherwise. The most reliable clinical assessment tool in determining the significance of TBI in a nonsedated, nonparalyzed patient is the Glasgow coma scale.
Acute subdural hematoma is the most common condition warranting emergency neurosurgery and is associated with the highest mortality.
Systemic hypotension (systolic blood pressures <90 mm Hg), hypoxemia (Pao2 <60 mm Hg), hypercapnia (Paco2 >50 mm Hg), and hyperthermia (temperature >38.0°C) have a negative impact on morbidity and mortality following head injuries, likely because of their contributions to increasing cerebral edema and intracranial pressure (ICP).
Current guidelines recommend maintaining cerebral perfusion pressure between 50 and 70 mm Hg and ICP at less than 20 mm Hg for patients with severe head injury.
Maintaining supranormal mean arterial blood pressures to assure spinal cord perfusion in areas of reduced blood flow due to cord compression or vascular compromise is likely to be of more benefit than steroid administration.
Major burns (a second- or third-degree burn involving >20% total body surface area [TBSA]) induce a unique hemodynamic response. Cardiac output declines by up to 50% within 30 minutes in response to massive vasoconstriction, inducing a state of normovolemic hypoperfusion (burn shock).
In contrast to fluid management for blunt and penetrating trauma, which discourages use of crystalloid fluids, burn fluid resuscitation emphasizes the use of crystalloids, particularly lactated Ringer’s solution, in preference to albumin, hydroxyethyl starch, hypertonic saline, and blood.
Carbon monoxide poisoning should be considered in all serious burn injury cases, as well as with lesser TBSA burns occurring in enclosed spaces. Unconsciousness or decreased levels of consciousness following burn injuries should be presumed to represent carbon monoxide poisoning.
Beyond 48 h after a major burn, succinylcholine administration is likely to produce potentially lethal elevation of serum potassium levels.
Anesthesia for Trauma & Emergency Surgery: Introduction
Trauma is a leading cause of morbidity and mortality in all age groups, and is the leading cause of death in the young. All aspects of trauma care, from that provided at the scene, through transport, resuscitation, surgery, intensive care, and rehabilitation, must be coordinated if the patient is to have the greatest chance for full recovery. The Advanced Trauma Life Support (ATLS) program developed by the American College of Surgeons’ (ACS) Committee on Trauma has, over time, resulted in an increasingly consistent approach to trauma resuscitation. The development of criteria for level one trauma centers has also improved trauma care by directing severely injured patients to facilities with appropriate resources.
Primary Survey
Increasingly, emergency medical technician-paramedics and flight nurses are trained to intubate patients in the prehospital environment. More providers capable of airway management in the critically ill or injured patient are now available to intervene in the hospital setting as well. As a result, the anesthesiologist’s role in providing initial trauma resuscitation has diminished in North America. This also means that when called upon to assist in airway management in the emergency department, anesthesia providers must expect a challenging airway, as routine airway management techniques likely have already proved unsuccessful.
There are three important aspects of airway management in the initial evaluation of a trauma patient: (1) the need for basic life support; (2) the presumed presence of a cervical spinal cord injury until proven otherwise; and (3) the potential for failed tracheal intubation. Effective basic life support prevents hypoxia and hypercapnia from contributing to the patient’s depressed level of consciousness. When hypercarbia produces a depressed level of consciousness, basic airway interventions often lessen the need for endotracheal intubation as arterial carbon dioxide levels return to normal. Finally, all trauma patients should be presumed to have “full” stomachs and an increased risk for pulmonary aspiration of gastric contents. Assisted ventilation should be performed with volumes sufficient to provide chest rise. Some clinicians will apply cricoid pressure, although the efficacy of this maneuver is controversial.
Cervical spine injury is presumed in any trauma patient complaining of neck pain, or with any significant head injury, neurological signs or symptoms suggestive of cervical spine injury, or intoxication or loss of consciousness. The application of a cervical collar (“C-collar”) before transport to protect the cervical spinal cord will limit the degree of cervical extension that is ordinarily expected for direct laryngoscopy and tracheal intubation. Alternative devices (eg, videolaryngoscopes, fiberoptic bronchoscopes) should be immediately available. The front portion of the C-collar can be removed to facilitate tracheal intubation as long as the head and neck are maintained in neutral position by a designated assistant maintaining manual in-line stabilization.
Alternative devices for airway management (eg, esophageal-tracheal Combitube, King supralaryngeal device) may be used if direct laryngoscopy has failed, or in the prehospital environment. These devices, blindly placed into the airway, isolate the glottic opening between a large inflatable cuff positioned at the base of the tongue and a distal cuff that most likely rests in the proximal esophagus (Figure 39-1). The prolonged presence of these devices in the airway has been associated with glossal engorgement resulting from the large, proximal cuff obstructing venous outflow from the tongue, and in some cases, tongue engorgement has been sufficiently severe to warrant tracheostomy prior to their removal. There is limited evidence that prehospital airway management in trauma patients improves patient outcomes; however, failed tracheal intubation in the prehospital environment certainly exposes patients to significant morbidity.
Figure 39-1
The King LT supralaryngeal device. The glottic opening lies between the large cuff positioned at the base of the tongue and the smaller balloon positioned in the proximal esophagus. The airway is not secured but rather isolated between the oropharynx and the proximal esophagus. (Reproduced, with permission, from King Systems Corporation, KLTD/KLTSD Disposable Supralaryngeal Airways Inservice Program, August 23, 2006, with permission.)
Airway management of the trauma patient is uneventful in most circumstances, and cricothyroidotomy or tracheostomy is rarely required to secure the trauma airway. When trauma significantly alters or distorts the facial or upper airway anatomy to the point of impeding adequate mask ventilation, or when hemorrhage into the airway precludes the patient from lying supine, elective cricothyroidotomy or tracheostomy should be considered before any attempts are made to anesthetize or administer neuromuscular blocking agents to the patient for orotracheal intubation.
In the multiple-injury patient, providers should maintain a high level of suspicion for pulmonary injury that could evolve into a tension pneumothorax when mechanical ventilation is initiated. Attention must be paid to peak inspiratory pressure and tidal volumes throughout the initial resuscitation. Pulmonary injury may not be immediately apparent upon the patient’s arrival at the hospital, and abrupt cardiovascular collapse shortly after instituting mechanical ventilation may announce the presence of a pneumothorax. This should be managed by disconnecting the patient from mechanical ventilation and performing bilateral needle thoracostomy (accomplished by inserting a 14-gauge intravenous catheter into the second interspace in the midclavicular line), and then by thoracostomy tube insertion. Inspired oxygen concentrations of 100% are used routinely in this early phase of resuscitation.
During the primary trauma patient survey, signs of a pulse and blood pressure are sought. Unless the trauma patient arrives at the hospital other than by ambulance, the resuscitation team will likely have received information about the patient’s vital signs from the prehospital personnel (emergency medical technicians, flight nurses). The absence of a pulse following trauma is associated with dismal chances of survival. The ACS Committee on Trauma no longer endorses the use of emergency thoracotomy in treating patients without blood pressure or palpable pulse following blunt trauma, even in the presence of organized cardiac activity, given the lack of evidence supporting survival following this intervention. Retrospective review of emergency thoracotomy in Europe failed to demonstrate resuscitation benefit of this procedure following either blunt or penetrating trauma in the setting of cardiac arrest. In the setting of chest trauma without detectable blood pressure or palpable pulse, current practice supports reserving resuscitative thoracotomy for patients who experience penetrating trauma and have preserved, organized cardiac rhythms or other signs of life.
In light of these recommendations, prompt placement of bilateral chest tubes and administration of a 500-1000 mL fluid bolus should be implemented in the pulseless victim of penetrating trauma. If return of spontaneous circulation does not occur promptly, more aggressive interventions are not indicated and resuscitation efforts can be terminated.
Once the presence of circulation is confirmed, a brief neurological examination is conducted. Level of consciousness, pupillary size and reaction, lateralizing signs suggesting intracranial or extracranial injuries, and indications of spinal cord injury are quickly evaluated. As noted earlier, hypercarbia often causes depressed neurological responsiveness following trauma; it is effectively corrected with basic life support interventions. Additional causes of depressed neurological function—eg, alcohol intoxication, effects of illicit or prescribed medications, hypoglycemia, hypoperfusion, or brain or spinal injury—must also be addressed. Mechanisms of injury must be considered as well as exclusion of other factors in determining the risk for central nervous system trauma. Persistently depressed levels of consciousness should be considered a result of central nervous system injury until disproved by diagnostic studies.
The patient must be fully exposed and examined in order to adequately assess the extent of injury, and this physical exposure increases the risk of hypothermia. The presence of shock and intravenous fluid therapy also place the trauma patient at great risk for developing hypothermia. As a result, the resuscitation bay must be maintained at near body temperature, all fluids should be warmed during administration, and the use of forced air patient warmers, either below or covering the patient, should be utilized.
Resuscitation
Certain trauma-related terminology must be understood and utilized in order to effectively communicate with surgeons during trauma resuscitations or surgeries in which blood loss is occurring. Hemorrhage classifications I-IV, damage control resuscitation, and damage control surgery are terms that quickly convey critical information between surgeons and anesthesia personnel, ensuring a common understanding of the various interventions that may be required to resuscitate a trauma or surgical patient experiencing bleeding. The ACS identifies four classes of hemorrhage. Understanding this classification scheme promotes more effective communication between surgeons and anesthesiologists.
Class I hemorrhage is the volume of blood that can be lost without hemodynamic consequence. The heart rate does not change and the blood pressure does not decrease in response to losing this volume of blood. In most circumstances, this volume represents less than 15% of circulating blood volume. The typical adult has a blood volume equivalent to 70 mL/kg. A 70-kg adult can be presumed to have nearly 5 L of circulating blood. Children are considered to have 80 mL/kg and infants, 90 mL/kg blood volume. Intravenous fluid is not required if the bleeding is controlled, as in brief, controlled bleeding encountered during an elective surgical procedure.
Class II hemorrhage is the volume of blood, that, when lost, prompts sympathetic responses to maintain perfusion; this usually represents 15-30% of circulating blood volume. The diastolic blood pressure will increase (a reflection of vasoconstriction) and the heart rate will increase to maintain cardiac output. Intravenous fluid or colloid is usually indicated for blood loss of this volume. Transfusions may be required if bleeding continues, suggesting progression to class III hemorrhage.
Class III hemorrhage represents the volume of blood loss (30-40% of circulating blood volume) that consistently results in decreased blood pressure. Compensatory mechanisms of vasoconstriction and tachycardia are not sufficient to maintain perfusion and meet the metabolic demands of the body. Metabolic acidosis will be detected on arterial blood gas analysis. Blood transfusions are necessary to restore tissue perfusion and provide oxygen to tissues. The patient may transiently respond to fluid boluses given in response to hemorrhage; however, if bleeding persists or given time for the fluid bolus to redistribute, the blood pressure will decline. Surgeons should be advised when this pattern persists, particularly during elective surgical cases where the development of shock is not expected. Class III hemorrhage may prompt an intervention such as a damage control procedure (see below).
Class IV hemorrhage represents life-threatening hemorrhage. When more than 40% of circulating blood volume is lost, the patient will be unresponsive and profoundly hypotensive. Rapid control of bleeding and aggressive blood-based resuscitation (ie, damage control resuscitation) will be required to prevent death. Patients experiencing this degree of hemorrhage will likely develop a trauma-induced coagulopathy, require massive blood transfusion, and experience a high likelihood of death.
Coagulation abnormalities are common following major trauma, and trauma-induced coagulopathy is an independent risk factor for death. Recent prospective clinical studies suggest that in up to 25% of major trauma patients, trauma-induced coagulopathy is present shortly after injury and before any resuscitative efforts have been initiated. In one report, acute traumatic coagulopathy was only related to the presence of a severe metabolic acidosis (base deficit ≥6 mEq/L) and appeared to have a dose-dependent relationship with the degree of tissue hypoperfusion; 2% of patients with base deficits less than 6 mEq/L developed coagulopathy compared with 20% of patients with base deficits greater than 6 mEq/L. Although injury severity scores were likely high in those developing coagulopathy, only the presence of the metabolic acidosis correlated to developing trauma-induced coagulopathy.
Global tissue hypoperfusion appears to have a key role in the development of trauma-induced coagulopathy. During hypoperfusion, the endothelium releases thrombomodulin and activated protein C to prevent microcirculation thrombosis. Thrombomodulin binds thrombin, thereby preventing thrombin from cleaving fibrinogen to fibrin. The thrombomodulin-thrombin complex activates protein C, which then inhibits the extrinsic coagulation pathway through effects on cofactors V and VIII (Figure 39-2). Activated protein C also inhibits plasminogen activator inhibitor-1 proteins, which increases tissue plasminogen activator, resulting in hyperfibrinolysis (Figure 39-3). One prospective clinical study found the following effects of hypoperfusion on coagulation parameters: (1) progressive coagulopathy as base deficit increases; (2) increasing plasma thrombomodulin and falling protein C (indicating activation of the protein levels with increasing base deficit), supporting the argument that the anticoagulant effects of these proteins in the presence of hypoperfusion are related to the prolongation of prothrombin and partial thromboplastin times; and (3) an influence of early trauma-induced coagulopathy on mortality.
Figure 39-2
Mechanism of trauma-induced coagulopathy. During periods of tissue hypoperfusion, thrombomodulin (TM) released by the endothelium complexes with thrombin. The thrombin-TM complexes prevent cleavage of fibrinogen to fibrin and also activate protein C (PC), reducing further thrombin generation through cofactors V and VIII. (Reproduced, with permission, from Brohi K, Cohen MJ, Davenport RA: Acute coagulopathy of trauma: mechanism, identification and effect. Curr Opin Crit Care 2007;13:680.)
Figure 39-3
Mechanism of hyperfibrinolysis in tissue hypoperfusion. Tissue plasminogen activator (tPA) released from the endothelium during hypoperfusion states cleaves plasminogen to initiate fibrinolysis. Activated protein C (aPC) consumes plasminogen activator inhibitor-1 (PAI-1) when present in excess, and reduced PAI-1 leads to increased tPA activity and hyperfibrinolysis. FDPs, fibrin degradation products; PC, protein C; TM, thrombomodulin. (Reproduced, with permission, from Brohi K, Cohen MJ, Davenport RA: Acute coagulopathy of trauma: Mechanism, identification and effect. Curr Opin Crit Care 2007;13:680.)
Trauma-induced coagulopathy is not solely related to impaired clot formation. Fibrinolysis is an equally important component as a result of plasmin activity on an existing clot. Tranexamic acid administration is associated with decreased bleeding during cardiac and orthopedic surgeries, presumably because of its antifibrinolytic properties. A randomized control study involving 20,000 trauma patients with or at risk of significant bleeding found a significantly reduced risk for death from hemorrhage when tranexamic acid therapy (loading dose, 1 g over 10 min followed by an infusion of 1 g over 8 h) was initiated within the first 3 h following major trauma. Figure 39-4 demonstrates the benefit of initiating this therapy in relation to the time of injury.
Figure 39-4
Influence of tranexamic acid in preventing death from bleeding. Outcomes ratios (OR) of tranexamic acid with 95% confidence interval (green area) on the x-axis and time (h) to treatment on the y-axis demonstrate improved survival if tranexamic acid therapy is initiated within 3 h of injury. The area of the curve to the left of OR 1.0 demonstrates the benefits of therapy, while that to the right demonstrates harm from intervention. (Reproduced, with permission, from Roberts I, Shakur H, Afolabi A, et al: The importance of early treatment with tranexamic acid in bleeding trauma patients: An exploratory analysis of the CRASH-2 randomised controlled trial. Lancet 2011;377:1096.)
Early coagulopathy of trauma is associated with increased mortality.
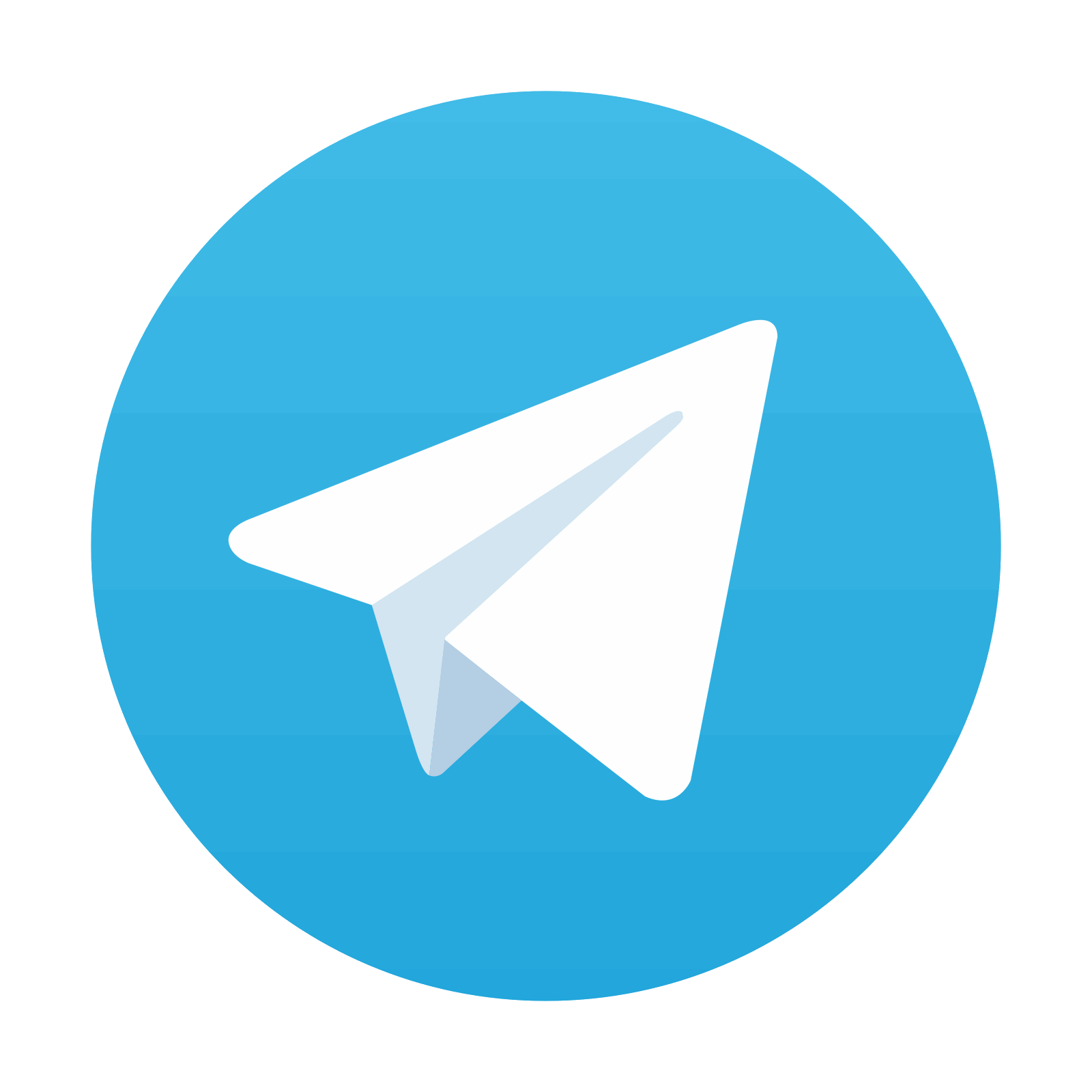
Stay updated, free articles. Join our Telegram channel

Full access? Get Clinical Tree
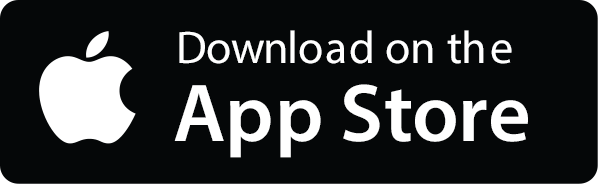
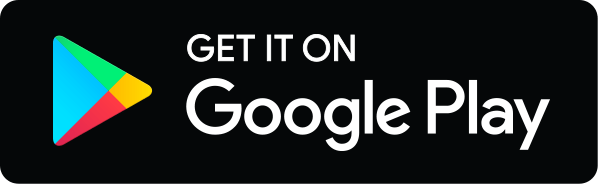
