Key Concepts
Regardless of the cause, intracranial masses present according to growth rate, location, and intracranial pressure. Slowly growing masses are frequently asymptomatic for long periods (despite relatively large size), whereas rapidly growing ones may present when the mass remains relatively small.
Computed tomographic and magnetic resonance imaging scans should be reviewed for evidence of brain edema, a midline shift greater than 0.5 cm, and ventricular displacement or compression.
Operations in the posterior fossa can injure vital circulatory and respiratory brainstem centers, as well as cranial nerves or their nuclei.
Venous air embolism can occur when the pressure within an open vein is subatmospheric. These conditions may exist in any position (and during any procedure) whenever the wound is above the level of the heart.
Optimal recovery of air following venous air embolism is provided by a multiorificed catheter positioned at the junction between the right atrium and the superior vena cava. Confirmation of correct catheter positioning can be accomplished by intravascular electrocardiography, radiography, or transesophageal echocardiography.
In a patient with head trauma, correction of hypotension and control of any bleeding take precedence over radiographic studies and definitive neurosurgical treatment because systolic arterial blood pressures of less than 80 mm Hg predict a poor outcome.
Massive blood loss from injuries to the great vessels can occur intraoperatively with thoracic or lumbar spine procedures.
Anesthesia for Neurosurgery: Introduction
Anesthetic techniques must be modified in the presence of intracranial hypertension and marginal cerebral perfusion. In addition, many neurosurgical procedures require patient positions (eg, sitting, prone) that further complicate management. This chapter applies the principles developed in Chapter 26 to the anesthetic care of neurosurgical patients.
Intracranial Hypertension
Intracranial hypertension is defined as a sustained increase in intracranial pressure (ICP) above 15 mm Hg. Intracranial hypertension may result from an expanding tissue or fluid mass, a depressed skull fracture, interference with normal absorption of cerebrospinal fluid (CSF), excessive cerebral blood volume (CBV), or systemic disturbances promoting brain edema (see below). Multiple factors are often simultaneously present. For example, tumors in the posterior fossa usually are not only associated with some degree of brain edema and mass effect, but they also readily obstruct CSF outflow by compressing the fourth ventricle (obstructive hydrocephalus).
Although many patients with increased ICP are initially asymptomatic, they typically develop characteristic symptoms and signs, including headache, nausea, vomiting, papilledema, focal neurological deficits, and altered consciousness. When ICP exceeds 30 mm Hg, cerebral blood flow (CBF) progressively decreases, and a vicious circle is established: ischemia causes brain edema, which in turn, increases ICP, resulting in more ischemia. If left unchecked, this cycle continues until the patient dies of progressive neurological damage or catastrophic herniation. Periodic increases in arterial blood pressure with reflex slowing of the heart rate (Cushing response) can be correlated with abrupt increases in ICP (plateau or A waves) lasting 1-15 min. This phenomenon is the result of autoregulatory mechanisms periodically decreasing cerebral vascular resistance and increasing arterial blood pressure in response to cerebral ischemia; unfortunately, the latter further increases ICP as CBV increases. Eventually, severe ischemia and acidosis completely abolish autoregulation (vasomotor paralysis).
An increase in brain water content can be produced by several mechanisms. Disruption of the blood-brain barrier (vasogenic edema) is most common and allows the entry of plasma-like fluid into the brain. Increases in blood pressure enhance the formation of this type of edema. Common causes of vasogenic edema include mechanical trauma, high altitudes, inflammatory lesions, brain tumors, hypertension, and infarction. Cerebral edema following metabolic insults (cytotoxic edema), such as hypoxemia or ischemia, results from failure of brain cells to actively extrude sodium causing progressive cellular swelling. Interstitial cerebral edema is the result of obstructive hydrocephalus and entry of CSF into brain interstitium. Cerebral edema can also be the result of intracellular movement of water secondary to acute decreases in serum osmolality (water intoxication).
Treatment of intracranial hypertension and cerebral edema is ideally directed at the underlying cause. Metabolic disturbances are corrected, and operative intervention is undertaken whenever appropriate. Vasogenic edema—particularly that associated with tumors—often responds to corticosteroids (dexamethasone). Vasogenic edema from trauma typically does not respond to corticosteroids. Blood glucose should be monitored frequently and controlled with insulin infusions (if indicated) when steroids are used. Regardless of the cause, fluid restriction, osmotic agents, and loop diuretics are usually effective in temporarily decreasing brain edema and ICP until more definitive measures can be undertaken. Diuresis lowers ICP chiefly by removing intracellular water from normal brain tissue. Moderate hyperventilation (Paco2 of 30-33 mm Hg) is often very helpful in reducing CBF, CBV, and ICP acutely, but may aggravate ischemia in patients with focal ischemia.
Mannitol, in doses of 0.25-0.5 g/kg, is particularly effective in rapidly decreasing intracranial fluid volume and ICP. Its efficacy is primarily related to its effect on serum osmolality. A serum osmolality of 300-315 mOsm/L is generally considered desirable. Mannitol can transiently decrease blood pressure by virtue of its weak vasodilating properties, but its principal disadvantage is a transient increase in intravascular volume, which can precipitate pulmonary edema in patients with borderline cardiac or renal function. Mannitol should generally not be used in patients with intracranial aneurysms, arteriovenous malformations (AVMs), or intracranial hemorrhage until the cranium is opened. Osmotic diuresis in such instances can expand a hematoma as the volume of the normal brain tissue around it decreases. Rapid osmotic diuresis in elderly patients can also occasionally cause a subdural hematoma due to rupture of fragile bridging veins entering the sagittal sinus. Rebound edema may follow the use of mannitol; thus, it is ideally used in procedures (such as a craniotomy for tumor resection) in which intracranial volume will be reduced.
Use of a loop diuretic (furosemide), although having a lesser maximal effect than mannitol and requiring up to 30 min, may have the additional advantage of directly decreasing formation of CSF. The combined use of mannitol and furosemide may be synergistic, but requires close monitoring of the serum potassium concentration.
Anesthesia & Craniotomy for Patients with Mass Lesions
Intracranial masses may be congenital, neoplastic (benign or malignant), infectious (abscess or cyst), or vascular (hematoma or arteriovenous malformation). Craniotomy is commonly undertaken for neoplasms of the brain. Primary tumors usually arise from glial cells (astrocytoma, oligodendroglioma, or glioblastoma), ependymal cells (ependymoma), or supporting tissues (meningioma, schwannoma, or choroidal papilloma). Childhood tumors include medulloblastoma, neuroblastoma, and astrocytoma. Regardless of the cause, intracranial masses present according to growth rate, location, and ICP. Slowly growing masses are frequently asymptomatic for long periods (despite relatively large size), whereas rapidly growing ones may present when the mass remains relatively small. Common presentations include headache, seizures, a general decline in cognitive or specific neurological functions, and focal neurological deficits. Symptoms typical to supratentorial masses include seizures, hemiplegia, or aphasia, whereas symptoms typical of infratentorial may include cerebellar dysfunction (ataxia, nystagmus, and dysarthria) or brainstem compression (cranial nerve palsies, altered consciousness, or abnormal respiration). As ICP increases, signs of intracranial hypertension also develop (see above).
The preoperative evaluation for patients undergoing craniotomy should attempt to establish the presence or absence of intracranial hypertension. Computed tomography (CT) and magnetic resonance imaging (MRI) scans should be reviewed for evidence of brain edema, a midline shift greater than 0.5 cm, and ventricular displacement or compression. The neurological examination should document mental status and any sensory or motor deficits. Medications should be reviewed with special reference to corticosteroid, diuretic, and anticonvulsant therapy. Laboratory evaluation should rule out corticosteroid-induced hyperglycemia, electrolyte disturbances due to diuretics, or abnormal secretion of antidiuretic hormone. Anticonvulsant blood concentrations may be measured, particularly when seizures are not well controlled.
Sedative or opioid premedication is best avoided if intracranial hypertension is suspected. Hypercapnia secondary to respiratory depression increases ICP. Corticosteroids and anticonvulsant therapy should be continued until the time of surgery.
In addition to standard monitors, direct intraarterial pressure monitoring and bladder catheterization are used for most patients undergoing craniotomy. Rapid changes in blood pressure during anesthetic procedures, positioning, and surgical manipulation are best managed with guidance from continuous invasive monitoring of blood pressure. Moreover, arterial blood gas analyses are necessary to closely regulate Paco2. Many neuroanesthesiologists zero the arterial pressure transducer at the level of the head (external auditory meatus)—instead of the right atrium—to facilitate calculation of cerebral perfusion pressure (CPP). End-tidal CO2 measurements alone cannot be relied upon for precise regulation of ventilation; the arterial to end-tidal CO2 gradient must be determined. Central venous access and pressure monitoring should be considered for patients requiring vasoactive drugs. Use of the internal jugular vein for access is theoretically problematic because of concern that the catheter might interfere with venous drainage from the brain. Some clinicians avoid this issue by passing a long catheter into the central circulation from the median basilic vein. The external jugular, subclavian, and femoral veins may be suitable alternatives for intraoperative use. A bladder catheter is necessary because of the use of diuretics, the long duration of most neurosurgical procedures, and its utility in guiding fluid therapy. Neuromuscular function should be monitored on the unaffected side in patients with hemiparesis because the twitch response is often abnormally resistant on the affected side. Monitoring visual evoked potentials may be useful in preventing optic nerve damage during resections of large pituitary tumors. Additional monitors for surgery in the posterior fossa are described below.
Management of patients with intracranial hypertension may be guided by monitoring ICP perioperatively. Various ventricular, intraparenchymal, and subdural devices can be placed by neurosurgeons to provide measurements of ICP. The transducer should be zeroed to the same reference level as the arterial pressure transducer (usually the external auditory meatus; see above). A ventriculostomy catheter provides the added advantage of allowing removal of CSF to decrease ICP.
Induction of anesthesia and tracheal intubation are critical periods for patients with compromised intracranial pressure to volume relationships, particularly if there is an elevated ICP. Intracranial elastance can be improved by osmotic diuresis, dexamethasone, or removal of small volumes of CSF via a ventriculostomy drain. The goal of any technique should be to induce anesthesia and intubate the trachea without increasing ICP or compromising CBF. Arterial hypertension during induction increases CBV and promotes cerebral edema. Sustained hypertension can lead to marked increases in ICP, decreasing CPP and risking herniation. Excessive decreases in arterial blood pressure can be equally detrimental by compromising CPP.
The most common induction technique employs propofol together with modest hyperventilation to reduce ICP and blunt the noxious effects of laryngoscopy and intubation. Cooperative patients can be asked to hyperventilate during preoxygenation. All patients receive controlled ventilation once the propofol has been injected. A neuromuscular blocker (NMB) is given to facilitate ventilation and prevent straining or coughing, both of which can abruptly increase ICP. An intravenous opioid given with propofol blunts the sympathetic response, particularly in young patients. Esmolol, 0.5-1.0 mcg/ kg, is effective in preventing tachycardia associated with intubation in lightly anesthetized patients.
The actual induction technique can be varied according to individual patient responses and coexisting diseases). Succinylcholine may theoretically increase ICP, particularly if intubation is attempted prior to the establishment of deep anesthesia. Succinylcholine, however, remains the agent of choice for rapid sequence induction or when there are concerns about a potentially difficult airway, as hypoxemia and hypercarbia are much more detrimental than any effect of succinylcholine to the patient with intracranial hypertension.
Hypertension during induction can be treated with β1-blockers or by deepening the anesthetic with additional propofol. Modest concentrations of volatile agents (eg, sevoflurane) may also be used, provided that hyperventilation is also used. Sevoflurane best preserves autoregulation of CBF and produces limited vasodilatation; it may be the preferred volatile agent in patients with elevated ICP. Because of their potentially deleterious effect on CBV and ICP, vasodilators (eg, nicardipine, nitroprusside, nitroglycerin, and hydralazine) are avoided until the dura is opened. Hypotension is generally treated with incremental doses of vasopressors (eg, phenylephrine).
Frontal, temporal, and parietooccipital craniotomies are performed in the supine position. The head is elevated 15-30° to facilitate venous and CSF drainage of CSF. The head may also be turned to the side to facilitate exposure. Excessive flexion or rotation of the neck impedes jugular venous drainage and can increase ICP. Before and after positioning, the tracheal tube should be secured, and all breathing circuit connections checked. The risk of unrecognized disconnections may be increased because the patient’s airway will not be easily assessed after surgical draping; moreover, the operating table is usually turned 90° or 180° away from the anesthesiologist.
Anesthesia can be maintained with inhalation anesthesia, total intravenous anesthesia techniques (TIVA), or a combination of an opioid and intravenous hypnotic (most often propofol) and a low-dose inhalation agent. Even though periods of stimulation are few, neuromuscular blockade is recommended—unless neurophysiological monitoring contradicts its use—to prevent straining, bucking, or movement. Increased anesthetic requirements can be expected during the most stimulating periods: laryngoscopy-intubation, skin incision, dural opening, periosteal manipulations, including Mayfied pin placement and closure. TIVA with remifentanil and propofol facilitates rapid emergence and immediate neurological assessment. Likewise, the α2-agonist dexmedetomidine can be employed during both asleep and awake craniotomies to similar effect.
Hyperventilation should be continued intraoperatively to maintain Paco2 at roughly 30-35 mm Hg. Lower Paco2 tensions provide little additional benefit and may be associated with cerebral ischemia and impaired oxygen dissociation from hemoglobin. Positive end-expiratory pressure (PEEP) and ventilatory patterns resulting in high mean airway pressures (a low rate with large tidal volumes) should be avoided because of a potentially adverse effect on ICP by increasing central venous pressure and the potential for lung injury. Hypoxic patients may require PEEP and increased mean airway pressures; in such patients, the effect of PEEP on ICP is variable.
Intravenous fluid replacement should be limited to glucose-free isotonic crystalloid or colloid solutions. Hyperglycemia is common in neurosurgical patients (corticosteroid effect) and has been implicated in increasing ischemic brain injury. Colloid solutions can be used to restore intravascular volume deficits, whereas isotonic crystalloid solutions are used for maintenance fluid requirements. Neurosurgical procedures are often associated with “occult” blood loss (underneath surgical drapes or on the floor).
Most patients undergoing elective craniotomy can be extubated at the end of the procedure, provided that neurological function is intact. Patients who will remain intubated should be sedated to prevent agitation. Extubation in the operating room requires special handling during emergence. Straining or bucking on the tracheal tube may precipitate intracranial hemorrhage or worsen cerebral edema. As the skin is being closed, the patient should resume breathing spontaneously. Should the patient’s head be secured in a Mayfield pin apparatus, care must be taken to avoid any patient motions (eg, “bucking on the tube”), which could promote neck or cranial injuries. After the head dressing is applied and full access to the patient is regained (the table is turned back to its original position as at induction), any anesthetic gases are completely discontinued, and the neuromuscular blockade is reversed. Rapid awakening facilitates immediate neurological assessment and can generally be expected following an appropriate anesthetic. Delayed awakening may be seen following opioid or sedative overdose, when the end-tidal concentration of the volatile agent remains >.2 minimum alveolar concentration (MAC), because of various metabolic derangements, or when there is a perioperative neurological injury. Patients may need to be transported to the CT scanner directly from the operating room for evaluation when they do not respond as predicted. Immediate reexploration may be required. Most patients are taken to the intensive care unit postoperatively for close monitoring of neurological function.
Anesthesia for Surgery in the Posterior Fossa
Craniotomy for a mass in the posterior fossa presents a unique set of potential problems: obstructive hydrocephalus, possible injury to vital brainstem centers, pneumocephalus, and, with unusual positioning, postural hypotension and venous air embolism.
Infratentorial masses can obstruct CSF flow through the fourth ventricle or the cerebral aqueduct of Sylvius. Small but critically located lesions can markedly increase ICP. In such cases, a ventriculostomy is often performed under local anesthesia to decrease ICP prior to induction of general anesthesia.
Operations in the posterior fossa can injure vital circulatory and respiratory brainstem centers and cranial nerves or their nuclei. Such injuries may occur as a result of direct surgical trauma or ischemia from retraction or other interruptions of the blood supply. Damage to respiratory centers is said to nearly always produce circulatory changes; therefore, abrupt changes in blood pressure, heart rate, or cardiac rhythm should alert the anesthesiologist to the possibility of such an injury. Such changes should be communicated to the surgeon. Isolated damage to respiratory centers may rarely occur without premonitory circulatory signs during operations in the floor of the fourth ventricle. Historically, some clinicians have employed spontaneous ventilation during these procedures as an additional monitor of brain function. At completion of the surgery, brainstem injuries may present as an abnormal respiratory pattern or an inability to maintain a patent airway following extubation. Monitoring brainstem auditory evoked potentials may be useful in preventing eighth nerve damage during resections of acoustic neuromas. Electromyography is also used to avoid injury to the facial nerve, but requires incomplete neuromuscular blockade intraoperatively.
Although most explorations of the posterior fossa can be performed with the patient in either a modified lateral or prone position, the sitting position may be preferred by some surgeons.
The patient is actually semirecumbent in the standard sitting position (Figure 27-1); the back is elevated to 60°, and the legs are elevated with the knees flexed. The head is fixed in a three-point holder with the neck flexed; the arms remain at the sides with the hands resting on the lap.
Careful positioning and padding helps avoid injuries. Pressure points, such as the elbows, ischial spines, heels, and forehead, must be protected. Excessive neck flexion has been associated with swelling of the upper airway (due to venous obstruction), and, rarely, quadriplegia (due to compression of the cervical spinal cord). Preexisting cervical spinal stenosis probably predisposes patients to the latter injury.
The sitting position increases the likelihood of pneumocephalus. In this position, air readily enters the subarachnoid space, as CSF is lost during surgery. In patients with cerebral atrophy, drainage of CSF is marked; air can replace CSF on the surface of the brain and in the lateral ventricles. Expansion of a pneumocephalus following dural closure can compress the brain. Postoperative pneumocephalus can cause delayed awakening and continued impairment of neurological function. Because of these concerns, nitrous oxide is rarely used for sitting craniotomies (see also below).
Venous air embolism can occur when the pressure within an open vein is subatmospheric. These conditions may exist in any position (and during any procedure) whenever the wound is above the level of the heart. The incidence of venous air embolism is greater during sitting craniotomies (20% to 40%) than in craniotomies in any other position. Entry into large cerebral venous sinuses increases the risk.
The physiological consequences of venous air embolism depend on the volume and the rate of air entry and whether the patient has a right-to-left intracardiac shunt (eg, patent foramen ovale [10% to 25% incidence]). The latter are important because they can facilitate passage of air into the arterial circulation (paradoxical air embolism). Modest quantities of air bubbles entering the venous system ordinarily lodge in the pulmonary circulation, where they are eventually absorbed. Small quantities of embolized air are well tolerated by most patients. When the amount entrained exceeds the rate of pulmonary clearance, pulmonary artery pressure rises progressively. Eventually, cardiac output decreases in response to increases in right ventricular afterload. Preexisting cardiac or pulmonary disease enhances the effects of venous air embolism; relatively small amounts of air may produce marked hemodynamic changes. Nitrous oxide, by diffusing into the bubbles and increasing their volume, can markedly accentuate the effects of even small amounts of entrained air. The dose for lethal venous air embolism in animals receiving nitrous oxide anesthesia is one-third to one-half that of control animals not receiving nitrous oxide.
Definitive signs of venous air embolism are often not apparent until large volumes of air have been entrained. A decrease in end-tidal CO2 or arterial oxygen saturation might be noticed prior to hemodynamic changes. Arterial blood gas values may show only slight increases in Paco2 as a result of increased pulmonary dead space (areas with normal ventilation but decreased perfusion). Conversely, major hemodynamic manifestations, such as sudden hypotension, can occur well before hypoxemia is noted. Moreover, large amounts of intracardiac air impair tricuspid and pulmonic valve function and can produce sudden circulatory arrest by obstructing right ventricular outflow.
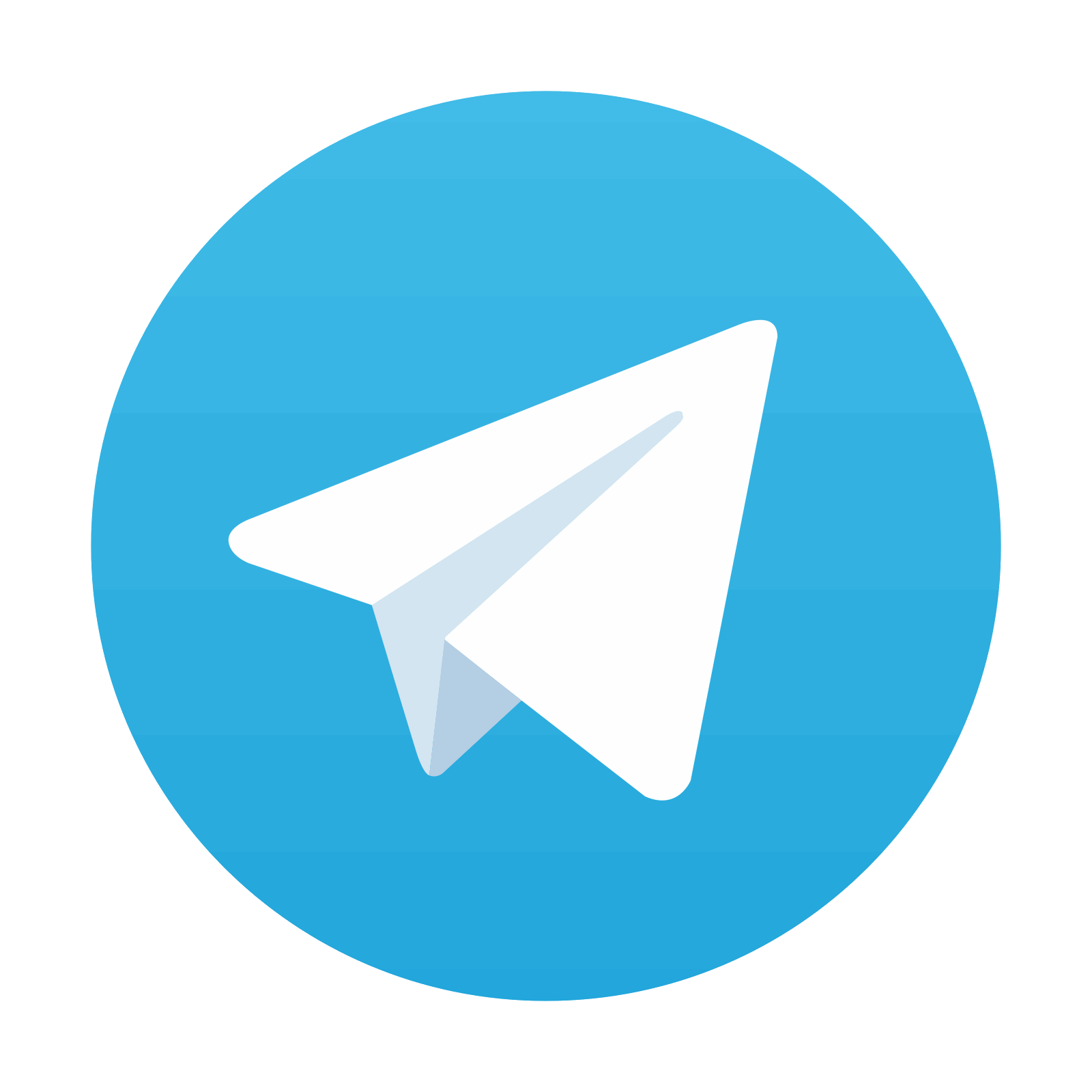
Stay updated, free articles. Join our Telegram channel

Full access? Get Clinical Tree
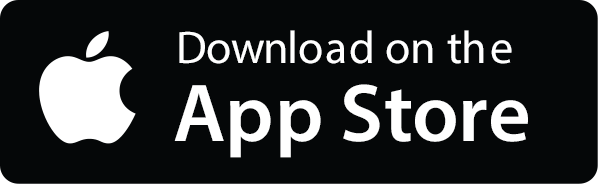
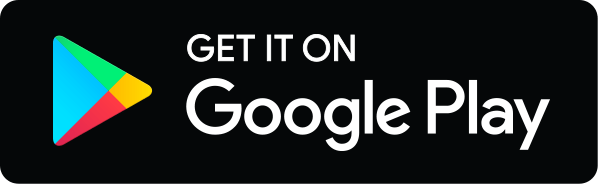