Anemia
Duke Kasprisin M.D.
The term “anemia” literally means “without blood” and is often used as if it were a disease, rather than a manifestation of an underlying disease process. Anemia is a condition of a decrease in the volume of blood, the number of erythrocytes, or the quantity of hemoglobin. Tissue hypoxia caused by the loss of oxygen is the principal defect caused by anemia.
Because of the numerous etiologies of anemia, they are frequently subdivided into their distinguishing characteristics, such as morphology, red cell size (microcytic, normocytic, macrocytic), or laboratory screening tests. An often-used method to categorize anemias is to describe them as decreased production, increased destruction (hemolysis), or bleeding. In reality, such clear distinctions are artificial. For example, a patient with chronic blood loss may have iron deficiency with a decrease in production. Still, these subdivisions are useful in making anemia more understandable.
ANATOMY, PHYSIOLOGY, AND PATHOLOGY
The most important function of erythrocytes is to transport oxygen to the tissues through hemoglobin. Hemoglobin accounts for 95% of erythrocyte protein (with most of the rest as enzymes, which provides energy for oxygen transfer and protection from oxidation and denaturation). Hemoglobin consists of a complex iron containing a porphyrin molecule called heme and a protein, globin.
The globin protein consists of two pairs of different polypeptides forming four intertwining chains. These chains are called alpha (α), beta (β), gamma (γ), and delta (δ). The type of polypeptide chain varies with age. The combination of globin chains determines the name of the hemoglobin.
In later fetal development, α and γ chains form fetal hemoglobin, (Hb F or α2γ2), which is the first hemoglobin to exist postnatally. Hb F is the predominant hemoglobin of fetal life and decreases rapidly after birth. In adults, the majority of hemoglobin consists of two α chains and two β chains, named hemoglobin A (Hb A or α2β2). Synthesis of this hemoglobin increases rapidly after birth and becomes the dominant form by the first few months of life. In addition to Hb A and the small remaining Hb F, an adult has minimal Hb A2, which consists of α2δ2. The heme portion of hemoglobin is consistent throughout life and does not vary.
Both the percentage of erythroid precursors and bone marrow cellularity decrease shortly after birth, returning to normal adult level at 1 to 3 months of age. During the decrease in erythrocyte production in this postnatal period, insults cannot be compensated for by increasing red cell production. Therefore, this period of life is an especially vulnerable time.
In adults, replication and maturation of erythrocytes occur in the bone marrow. As the erythrocyte matures, it accumulates hemoglobin, loses its nucleus, and develops its final appearance of a biconcave disc. A critical characteristic of erythrocytes is deformability, which allows for negotiation through the capillary channels of the microcirculation.
As the erythrocyte ages, the cell begins to fragment and progressively loses its deformability. Changes in the biophysical characteristics cause the erythrocyte to become phagocytised by the macrophages in the spleen, liver, and bone marrow. The hemoglobin is degraded, the iron is reused or stored, and the protein portion of hemoglobin is metabolized to bile pigments, biliverdin and bilirubin. When these pigments are overproduced, the patient appears jaundiced.
The life span of erythrocytes is approximately 120 days. One percent of new cells are released each day to compensate for an equal number being destroyed. If this equilibrium is not maintained, tissue hypoxia results. Hypoxia is the primary stimulus for erythrocyte production through the release of erythropoietin. Erythropoietin is a hormone produced in the kidneys and to a lesser extent in the liver. Erythropoietin is the most important regulatory factor for erythropoiesis and is responsible for the proliferation and differentiation of erythroid progenitor cells. In the presence of anemia, erythropoietin production increases and stimulates a six- to eightfold increase of red cell production in the bone marrow.
TYPES OF ANEMIA
The subdivision of anemia by etiology is useful but does not account for the multiple mechanisms often present. For instance, chronic renal failure causes a loss of erythropoietin production, but hemolysis and coagulopathy can be components as well. Therefore, the etiology of anemia will be categorized by the principal mode of action, understanding these limitations.
Decreased Production
Effective erythropoiesis requires a functional marrow, adequate erythropoietin stimulation, and a sufficient quantity and proper assimilation of nutrients. Any problem maintaining effective erythropoiesis leads to a decrease in red cell production.
Some of the more common causes of anemia caused by decreased production are listed in Table 36-1.
MYELOPHTHISIC ANEMIA
Myelophthisic anemia or marrow failure is a consequence of the infiltration of nonhematopoietic-producing cells. This can be caused by the abnormal proliferation of marrow components, as in the leukemias and multiple myeloma, or by metastasis from cancers, such as breast or prostate cancer or neuroblastoma, or from noncancerous cells’ crowding normal marrow components, as in the osteopetroses (marble bone disease), the lipid storage diseases, and myelofibrosis.
Myelofibrosis, the replacement of the bone marrow by fibrosis, can be primary (idiopathic) or secondary to other diseases, such as myeloproliferative disorders (eg, chronic myelogenous leukemia). Myelofibrosis is often associated with myeloid metaplasia that is extramedullary.
The myelophthisic anemias produce a normocytic, normochromic anemia. If the infiltration of the bone marrow is extensive, there is considerable variation of red cell shapes and immature red cells and granulocytes in the peripheral smear (leukoerythroblastosis) (Table 36-2).
MYELODYSPLASTIC SYNDROME
This syndrome is characterized by a clonal hematopoietic stem cell anomaly with abnormal maturation and ineffective hematopoiesis. The bone marrow can be either hypercellular or normocellular. Red cells are macrocytic but not as large as in megaloblastic anemia; they are occasionally normocytic.
In the past, this syndrome was identified as preleukemia, refractory anemia, or sideroachrestic anemia. The French-American-British classification system includes refractory anemia, refractory anemia with sideroblasts, refractory anemia with excess blasts, chronic myelomonocytic leukemia, and refractory anemia with excess blasts in transformation (Kouides & Bennett, 1996). The distinction between these categories is based on morphology and the number of blasts seen. The abnormal clone of cells is not stable and can gradually degenerate into a less favorable classification, including acute nonlymphocytic leukemia.
Treatment is controversial. Early therapy does not appear to affect the progression of the disease.
Marrow failure can also result from an acquired absence of erythrocyte precursors (pure red cell aplasia) or of hematopoietic cells in general (aplastic anemia). Drugs are a frequent cause of marrow failure, including cancer chemotherapeutic drugs, resulting in a usually reversible marrow aplasia. Chloramphenicol has been associated with a rare idiosyncratic aplastic anemia.
CONGENITAL PURE RED CELL APLASIA
Congenital pure red cell aplasia (congenital hypoplastic anemia, Blackfan-Diamond syndrome) has multiple etiologies. Acquired pure red cell aplasia often is autoimmune, with the autoantibody directed against erythroid precursors or erythropoietin. Many adult cases are associated with thymoma. Other causes include drugs, toxins, riboflavin deficiency, infection, renal failure, malnutrition, and leukemia. The anemia is normochromic and normocytic or less commonly macrocytic. The reticulocyte count is reduced and the bone marrow shows an absence of red cell precursors, although precursors to other hematopoietic cell lines are abundant.
APLASTIC ANEMIA
The aplastic or hypoplastic anemias are characterized by a hypocellular bone marrow with fatty replacement as well as thrombocytopenia and leukopenia, leaving patients at risk for hemorrhage and infection. The best-known congenital or constitutional aplastic anemia is Fanconi’s anemia, an autosomal recessive disorder associated with pancytopenia and skeletal anomalies, mostly of the hands and forearms. Other signs include café-au-lait spots, short stature, renal anomalies, microcephaly, and other central nervous system disease. Acquired aplastic anemias are most often idiopathic (50%), although drugs, toxins, radiation, paroxysmal nocturnal hemoglobinuria, and infections such as hepatitis can all play a role.
INEFFECTIVE ERYTHROPOIESIS
Ineffective erythropoiesis occurs in renal failure from lack of erythropoietin production. Lack of an adequate quantity or assimilation of nutrients is another common cause of this anemia.
Iron Deficiency Anemia
Iron deficiency anemia is the most common type of anemia. Anemia caused by dietary deficiency of iron is most often seen in infants and children and during pregnancy, when rapid growth requires increased amounts of iron. Dietary iron deficiency anemia in adults is uncommon in this country, although not in nations with severely inadequate diets. However, iron deficiency without anemia is extremely common in the United States.
In this country, iron deficiency anemia in adults is more often related to chronic blood loss. Many women are iron-deficient secondary to menstrual bleeding or pregnancy. Adolescent
girls are particularly prone to iron deficiency anemia because of menstruation combined with a diet lacking adequate iron content.
girls are particularly prone to iron deficiency anemia because of menstruation combined with a diet lacking adequate iron content.
In men, iron deficiency anemia is usually pathologic and often the first sign of colon cancer. Intestinal parasites are a major cause of iron loss in some areas of the world. Deficient iron ingestion may also be seen in the elderly or in alcoholics, and inadequate absorption can be seen in severe inflammatory bowel disease.
Iron deficiency can be associated with pica, the craving to ingest nonfood items; this is most common in children and pregnant women. Ice-eating (pagophagia) and dirt-eating (geophagia) are two of the most common, but a great variety of objects may be ingested.
Iron-Related Anemias
Other iron-related anemias include defects in the iron transfer to red cell precursors. In atransferrinemia, the protein transferrin, which transports iron from storage sites to the erythrocyte precursors, is either absent or abnormal. Total iron-binding capacity is low, in contrast to iron deficiency anemia, in which it is high.
In the sideroblastic anemias, iron is not properly used for hemoglobin synthesis. Iron stores are increased, with erythroid hyperplasia in the bone marrow. Using special stains to detect iron, ringed sideroblasts or iron deposits on developing erythrocytes can be seen. This anemia is primary, idiopathic, or secondary to drugs, toxins, alcohol, and other diseases. Some of the hereditary forms of this disease respond to pyridoxine therapy.
Other Nutritional Anemias—B12 and the Macrocytic Anemias
Vitamin B12 and folic acid deficiencies lead to megaloblastic anemia or large red cells and disordered maturation. Dietary deficiencies of these two vitamins are uncommon in this country. The liver can store large quantities of B12, so prolonged periods of deficiency would be required to deplete these stores before the patient became symptomatic.
The most common form of anemia related to B12 is pernicious anemia. These patients lack a protein, intrinsic factor, which is secreted by the parietal cells of the gastric mucosa. Intrinsic factor binds B12 and allows absorption by the intestinal mucosa. Pernicious anemia is associated with atrophic gastric mucosa and hypochlorhydria. Some types are congenital; others are autoimmune, because autoantibodies to the gastric parietal cell or intrinsic factor are frequently observed, as well as endocrine deficiencies, particularly of the thyroid and adrenal glands. Other conditions that affect B12 include fish tapeworms, blind loop syndrome, intestinal inflammatory diseases, and gastric resections where changes in intestinal flora result.
Folic acid, which is abundant in green leafy vegetables, liver, mushrooms, and yeast, is easily destroyed by overcooking. Because the body cannot store as much folic acid as B12, it is easier to develop folic acid deficiency. However, folic acid deficiency is more commonly associated with alcoholism, malabsorption syndromes, dialysis, and drugs (eg, methotrexate and other folic acid antagonists, some anticonvulsants, and drugs that affect DNA metabolism).
There are increased requirements for folic acid in pregnancy, infancy, malignancy, some hyperhematopoiesis conditions (eg, sickle cell disease and thalassemia), and hypermetabolic conditions (eg, hyperthyroidism). In patients with megaloblastic anemia, it is important to rule out B12 deficiency, because treatment with folic acid will help the anemia but the neurologic defects will progress.
OTHER ANEMIAS CAUSED BY DECREASED PRODUCTION
Endocrine deficiencies, especially hypothyroidism, frequently result in anemia. Pituitary dysfunction, especially when thyroid-stimulating hormone is involved, produces a hypoproliferative anemia. Adrenocortical deficiency (Addison’s disease) is also associated with anemia. Loss of androgen production in males causes a decrease in hematocrit to female levels. Anemia is reversed by hormonal replacement in endocrine diseases.
ANEMIA OF CHRONIC DISEASE
Anemia secondary to infection, inflammation (eg, rheumatoid arthritis), and neoplasm is common, although anemia of chronic disease does not have to be associated with a chronic disease. These normochromic, normocytic anemias are usually mild but difficult to resolve. Besides decreased production of red cells, there is also a slight decrease in red cell survival in this anemia.
Anemia of chronic disease results from an abnormality of iron reuse, causing a failure in erythroid hyperplastic compensation. A decrease in erythropoietin may also be a factor.
Increased Destruction
Table 36-3 lists some of the more common causes of anemia caused by increased destruction or hemolysis. The hemolytic anemias are usually divided into those whose etiology is intrinsic to the red cell (corpuscular) and those that are extrinsic to the red cell (extracorpuscular). Intrinsic red cell defects can be caused by abnormalities in the red cell membrane, the enzyme systems that enable the red cell to function, and hemoglobinopathies. Extrinsic defects are immune or nonimmune.
INTRINSIC RED CELL DEFECTS
Membrane Defects—Hereditary Spherocytosis
Many of the intrinsic red cell defects are genetic in nature and manifest as alterations in structural proteins. The most common is hereditary spherocytosis (Hassoun & Palek, 1996). This disease is autosomal dominant, and the red cells take on a spherocytic
shape instead of the normal biconcave disc, increasing the osmotic fragility of the cell. The abnormal shape impedes red cell flexibility and ability to navigate the microcirculation of the spleen, resulting in increased phagocytization by the macrophages. The spleen enlarges and traps still more spherocytes, resulting in severe anemia and congestive heart failure if untreated. If the hemolysis is brisk, the patient appears jaundiced. Cholelithiasis is common because the rapid destruction of cells results in bilirubin stones in the gallbladder.
shape instead of the normal biconcave disc, increasing the osmotic fragility of the cell. The abnormal shape impedes red cell flexibility and ability to navigate the microcirculation of the spleen, resulting in increased phagocytization by the macrophages. The spleen enlarges and traps still more spherocytes, resulting in severe anemia and congestive heart failure if untreated. If the hemolysis is brisk, the patient appears jaundiced. Cholelithiasis is common because the rapid destruction of cells results in bilirubin stones in the gallbladder.
A family history of multiple members with anemia, jaundice, splenomegaly, or gallstones is common. However, because of incomplete penetrance of the gene, generations may be skipped, or both mild and severe symptoms may appear in the same family.
Patients with concurrent infections may go into aplastic crises. Severe hemolytic anemia accompanied by decreased red cell production can be life-threatening. Splenectomy is the only treatment that resolves the anemia. The spherocytosis is unaffected by splenectomy, but the rate of red cell production in the bone marrow is able to compensate for the loss by hemolysis once the major site of destruction has been removed. However, the immunologic risks of splenectomy include increased susceptibility to pneumococci and other encapsulated bacteria, particularly in children.
Red Cell Enzyme Defects—Glucose-6-Phosphate Dehydrogenase
As the red cell matures, it loses its nucleus, mitochondria, and microsomes, leaving very limited metabolic capacity. To maintain the energy necessary to function and transport oxygen, the primary source of energy for red cells is glucose. Glucose is metabolized via one of two pathways: the Embden-Meyerhof (glycolytic) or the hexose monophosphate shunt. Numerous enzymes can be affected by hereditary defects in these two pathways, resulting in clinical disorders, including hemolytic anemias. The most common of these enzyme defects involves an enzyme in the hexose monophosphate shunt pathway, glucose-6-phosphate dehydrogenase (G6PD). The gene for this enzyme is present on the X chromosome. More than 350 variants of this protein have been identified. Although some are capable of causing disease, others are protective. For instance, many variants of red cell enzymes and hemoglobins have evolved because they impart a partial resistance to Plasmodium falciparum malaria. Malaria, one of the most lethal infectious diseases in the world, reproduces in red cells. Severe G6PD deficiency impedes the survival of erythrocytes to these parasites.
The predominant form of this enzyme is GdB, G6PD B, or G-6-PDB. The most common variant is GdA, which functions 80% to 90% as well as GdB. GdB differs from GdA by a single amino acid, the substitution of aspartic acid for asparagine. The variant GdA−, which is frequently seen in African Americans, has markedly decreased enzyme activity and can cause hemolysis. Males with this variant and females homozygous for this gene usually have no symptoms but can develop a severe hemolysis when their red cells are stressed (eg, infection, certain drugs, diabetic acidosis). A reaction to primaquine, an antimalarial, first identified this enzyme deficiency.
Another variant, GdMediterranean, has even less enzyme activity than GdA−. Caucasians, especially Italians, Greeks, Arabs, and Sephardic Jews, are affected with this gene. This disease results in an even more severe hemolysis than GdA−.
Because the gene for G6PD is on the X chromosome, all males who inherit this gene are affected. Women with one affected gene are usually normal, but abnormal G6PD genes are so common that homozygous females are often seen. In females, only one of the two X chromosomes is active in any cell (Lyon hypothesis). Therefore, males and females with normal variants of G6PD have equal enzyme levels. If a woman is heterozygous for one of the deficient genes, she will have approximately half the normal level of enzyme. However, women with severely deficient genes may have normal enzyme levels. This is because red cells that inactivate X chromosomes containing affected genes are quickly destroyed. The only red cells that survive have the normal gene.
In patients with the most severe deficiency of G6PD, jaundice, hemoglobinuria (with resulting dark urine), renal failure, and severe, symptomatic anemia usually result within 1 to 3 days of the causative stress. In patients with less severe hemolysis, there is a moderate decline in hemoglobin. These episodes can easily be missed unless the diagnosis is considered.
Screening tests for this disease can detect the abnormality only if sufficient red cells are affected. Unfortunately, after a hemolytic episode, the oldest cells, with the lowest enzyme activity, are destroyed. The remaining younger cells have greater G6PD levels, and because these are the only ones that can be measured, false-negative results may occur. Because younger red cells contain more G6PD, the GdA- variant is usually self-limiting once the older cells are destroyed.
Drugs that cause hemolytic episodes include antimalarials (primaquine, quinacrine), antibiotics (sulfonamides, nitrofurantoin), and analgesics (acetylsalicylic acid). A G6PD-deficient patient treated with certain antibiotics and analgesics becomes a prime candidate for a hemolytic episode. Drugs that cause hemolysis in G6PD-deficient patients are listed in Table 36-4.
Hemoglobinopathies
Other common causes of hemolysis are abnormalities in hemoglobin (hemoglobinopathies) or in hemoglobin synthesis (thalassemias). For oxygen to be bound in the lung and released in tissue, a very defined structure of hemoglobin is required. Variations can cause increased or decreased oxygen affinity, resulting in hemoglobin that either does not bind or does not release oxygen. Other variations may affect red cell shape and cause rapid destruction of cells and anemia.
Sickle Cell Disease
Sickle hemoglobin, the most common hemoglobinopathy, is caused by the replacement of a glutamic acid by valine at position 6 of β chain and is written Hb S or α2β2S or α2β26Glu→val. The α chains are normal in Hb S. There is a one-in-four chance that two persons with sickle trait will have a child with sickle cell disease.
Patients with sickle cell disease produce mostly sickle hemoglobin, variable amounts of Hb F, and normal but small amounts of Hb A2. The morphology of the red cells in sickle cell trait is normal. In sickle cell disease, however, the peripheral smear is markedly abnormal, with many bizarre-looking cells, especially the hallmark of the disease: red cells in the shape of sickles. The sickle cells are rigid, occlude small blood vessels, and damage tissue. The abnormally shaped cells quickly hemolyze, leading to the severe anemia that is classic in this disease.
The predominant form of hemoglobin in the newborn is Hb F, which contains no β chains. As a result, patients with sickle cell disease are rarely symptomatic as newborns. When β-chain production increases, sickle cells begin to appear and patients begin to have myriad symptoms. Almost every body system is eventually involved. Morbidity is caused by severe anemia and vaso-occlusive problems.
Patients with sickle cell disease usually have hematocrits between the high teens and low 30s. The anemia can be exaggerated by marrow failure caused by infection (particularly parvovirus B19), folic acid deficiency caused by increased erythrocyte production as a response to the anemia, and splenomegaly from increased entrapment and destruction of red cells.
Symptoms begin in infancy, often with acute and painful swelling of the hands and feet. Growth and maturation are hindered. Anemia develops quickly and can progress to congestive heart failure. The vaso-occlusive nature of the disease causes severe painful crises, often triggered by infection. Although these painful episodes can occur almost anywhere, they frequently occur in the abdomen, chest, and joints. It is often difficult to distinguish painful crises from other pathology because of the numerous systems that can be involved in this disease.
The clinical manifestations of sickle cell disease are listed in Table 36-5. Many symptoms can present a diagnostic dilemma. The development of gallstones and cholecystitis is often difficult to differentiate from abdominal pain crises. Bony changes mimic osteomyelitis on x-ray.
Other manifestations cause treatment difficulties as well. The kidneys lose their ability to concentrate the urine, so patients can dehydrate easily. Recurring strokes may require chronic transfusion to prevent further vaso-occlusive episodes, but transfusions add to the possibility of further complications.
The spleen infarcts early in life and the patient suffers all the immunologic risks of asplenia. Autosplenectomy is usually complete by age 8. Because of the increased risk of infection with Pneumococcus, Haemophilus, and salmonella, patients should be seen quickly if infection is a concern. Pneumococcal sepsis can progress from an asymptomatic state to death within a few hours. Therefore, pneumococcal vaccine is routinely given to these patients. Neurologic symptoms are common and recurring strokes usually require chronic transfusions to prevent further vaso-occlusive episodes.
In general, persons with sickle cell trait are completely asymptomatic. However, on occasion they may experience hematuria, splenic infarcts when exposed to severe hypoxia, problems concentrating urine, and crises that may proceed to death with extreme exercise, especially at high altitudes. Anesthesiologists must be particularly careful to avoid hypoxia in any patient with sickle hemoglobin.
Hemoglobin C Disease
The second most common hemoglobinopathy caused by a single amino acid substitution is hemoglobin C. Hemoglobin C consists of two normal α chains and β chains with a replacement of a glutamic acid by lysine at position 6, and is written Hb C or α2β2C or α2β26Glu→lys. Persons who inherit the Hb C gene from only one parent and Hb A from the other have Hb C trait, with 60% to 75% Hb A and 25% to 40% Hb C. The gene occurs in approximately 2% of African Americans.
Heterozygous persons are asymptomatic, but their peripheral blood smears contain numerous target cells, which look like little bull’s-eyes. Persons with homozygous Hb C have a mild anemia and splenomegaly. The peripheral smear demonstrates numerous target cells and intracellular crystals of hemoglobin. Recurrent arthralgias and abdominal pain are the predominant symptoms. Jaundice may be present.
Thalassemia
The thalassemias are inherited disorders with absence or diminished synthesis of one or more of the globin chains. Any chain can be involved. Like many of the abnormal hemoglobins, thalassemia parallels the malaria belt. The severity of the thalassemia depends on the chain involved and the degree to which synthesis is decreased. For example, because δ chains represent only a small proportion of hemoglobin chain synthesis, complete absence of δ-chain synthesis exists but is not a clinically significant entity.
The lack of balanced synthesis among hemoglobin chains in thalassemia leads to deficient production of one chain, with decreased hemoglobin and hypochromic, microcytic cells. There is an abundance of a second chain, which precipitates in the red cell, causing cell membrane damage. Thalassemia is a mixture of ineffective erythropoiesis and hemolysis.
Most populations have two genes for α chains on each chromosome for a total of four genes. If all four genes fail to produce α chains, a condition termed hydrops fetalis results. The patient becomes severely anemic and edematous and dies in utero or shortly after birth. If only one α gene is functional, the patient has Hb H disease, named because the excess β chains form a tetramer β4 called Hb H. These patients suffer a moderate anemia (thalassemia intermedia).
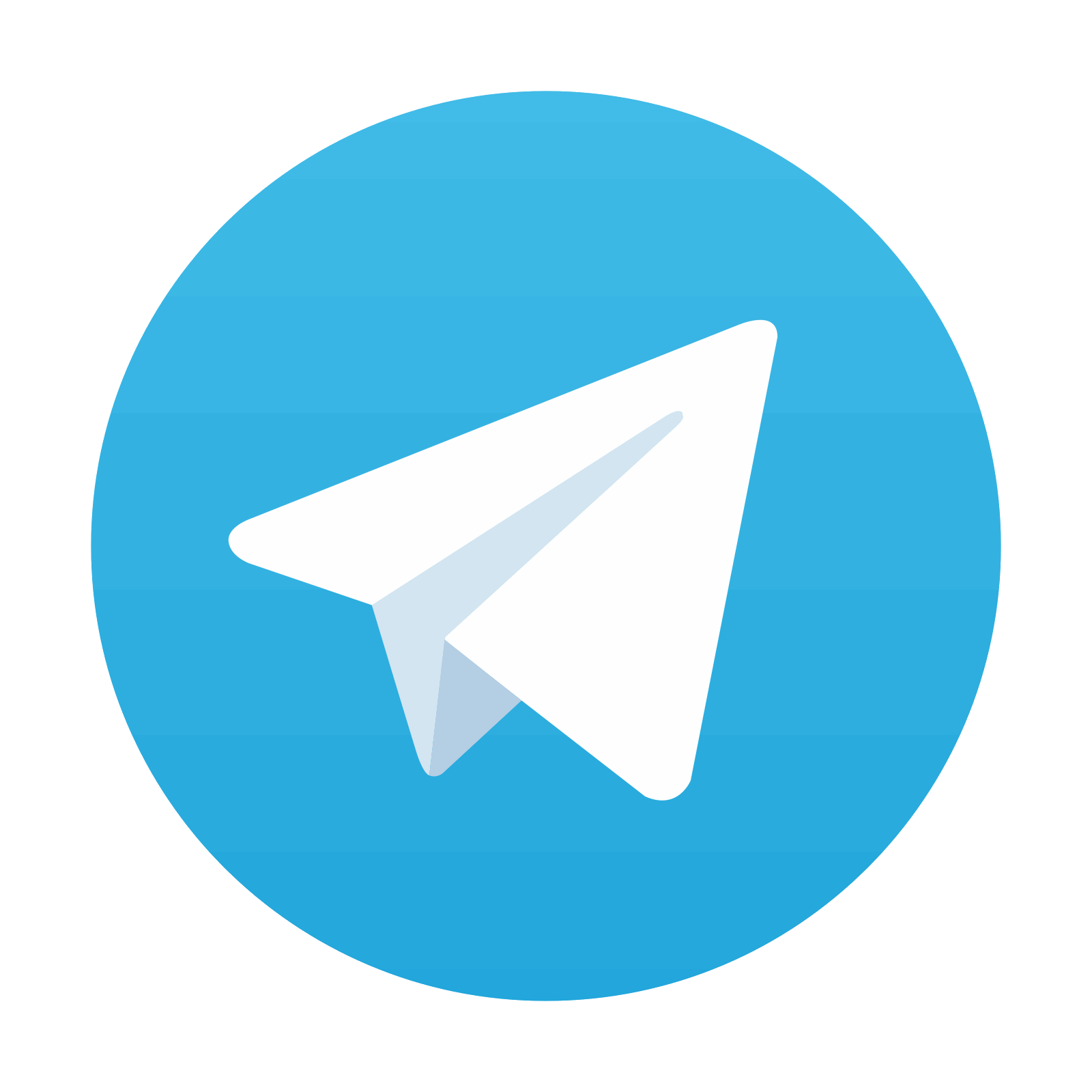
Stay updated, free articles. Join our Telegram channel

Full access? Get Clinical Tree
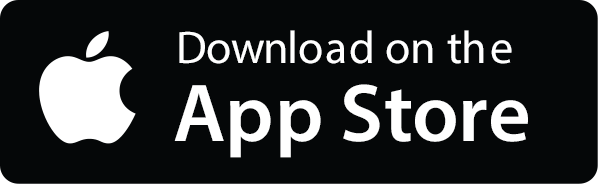
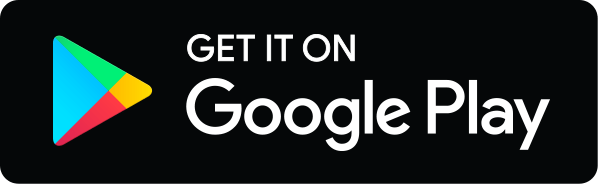
