DEFINITION
The term toxicity, as it is considered in regional anesthesia and pain medicine, refers generally to the effects of substances that are injurious to tissues and/or the body. In considering this definition further, we need to distinguish between toxicity at the tissue level, that is, in the nervous system as with the contemporary concept of neurotoxicity and at systemic sites (Box 8-1). Yaksh1 highlights concerns about the former by pointing out that the use of untested drugs and drug combinations is rampant in regional anesthesia and pain medicine, and thus our concern is genuine and deserved. Toxicity at the tissue level is clearly distinct from systemic toxicity. An example of the latter is opioid-induced neurotoxicity, as presented by Sweeney and Bruera.2 They declare this to be a “recently recognized syndrome of neuropsychiatric consequences of opioid administration.” The syndrome includes cognitive impairment, severe sedation, hallucinations, delirium, myoclonus, seizures, hyperalgesia, and allodynia. The syndrome seems to be particularly related to the use of high doses of opioids for prolonged periods of time. Another common example of systemic toxicity would be local anesthetic systemic toxicity (LAST) (Chapter 7).3–6 This chapter focuses on tissue neurotoxicity as it relates to the neuraxis or peripheral nerves.
BOX 8-1 Manifestations of Drug-induced Toxicity
Drug-induced toxicity may be caused by:
Common anesthetic agents: local anesthetics, opioids
Adjuvants: epinephrine, clonidine, midazolam
Preservatives: parabens, bisulfites
Toxicity may manifest as:
Systemic toxicity: cardiovascular effects, central nervous system effects
Direct tissue toxicity
Ischemia-related toxicity
SCOPE
Reality dictates that pharmacy, prescription order, and health care provider errors will occur in the provision of clinical care, yielding unexpected dosing or effects from drugs placed in the perispinal or peripheral nerve spaces. When this occurs, focal neurotoxicity might be manifested, as might drug withdrawal, overdose, or systemic toxicity. Given the extensive literature addressing the rediscovery of possible local anesthetic toxicity at the tissue level presenting as transient neurologic symptoms (TNSs) (Chapter 13), it is also evident that drugs of long-standing, traditional benefit might also come under new scrutiny.7,8 As Reichert and Butterworth9 noted, “clinicians must recognize that even local anesthetics labeled as ‘preservative-free’ contain ‘inactive ingredients’.” To be complete, research into single-agent neurotoxicity must isolate the causative agent, separating it from pH, osmolarity, the chemical vehicle of the injected drug, consequences of the use of needles and catheters to place the drugs, and the patient’s preexisting physiologic condition.10 The quest for better adjuvant substances combined with primary drugs to enhance their clinical actions by facilitating additive effects or prolonging the primary drug’s action is in part spurred by the desire to reduce total local anesthetic dose, but also raises the potential of toxicity from the adjuvants themselves (Table 8-1).
TABLE 8.1 Local Anesthetics and Their Additives
Note: Levobupivacaine and ropivacaine contain no additives.
EDTA, ethylenediaminetetraacetic acid.
Table modified from Weinberg GL. Treatment of local anesthetic systemic toxicity (LAST). Reg Anesth Pain Med 2010;35:188–193, with permission.
Indeed, clinicians have been raising more concern about the potential toxicity of everyday drugs in recent years. In a perceptive comment, Sawynok11 noted that “the demonstration of neurotoxic effects following local administration [of amitriptyline] is an important reminder of the need for careful assessment of novel routes of drug administration.” Lavand’homme’s12 editorial from 2006, commenting on the lack of medical science following classic, fundamental steps in the study of intrathecal (IT) midazolam, truly reveals “lessons” that are worth noting. She acknowledges the clinical demand for new agents and their application to, meritoriously, lessen pain. However, “our decision to use spinal analgesics should result from a balance between the risks…and the benefits…. Risks should be our first concern because every spinal drug is potentially neurotoxic and able to produce a local lesion leading to a functional deficit, either temporary or permanent.” She outlines the recommended pathway for drug development in this statement—“Ideally, before widespread use, a spinal drug should undergo specific and complete histologic, physiologic, and behavioral testing in at least two different species (a small-animal model and a large-animal model) followed by safety trials in humans.”
This growing awareness about a rush of research, embodied only in small-scale studies or case reports, that advocates the premature clinical application of drugs before a strict scientific protocol of development was documented, has necessarily resulted in major anesthesiology journals adopting a more rigorous policy for accepting manuscripts that promote the off-label use of old and new investigational drugs.13,14 Because all drugs are, indeed, potentially neurotoxic, a journal could give implicit approval by publishing a study that advocates the off-label use of a drug. The new policies contain specific criteria for authors about the submission of manuscripts addressing the off-label use of drugs. Essentially, the editorial boards can ask for more documentation (than even the author’s institutional review board required), request more information about relevant animal and human neurotoxicity studies, clarify that the study was done under a Federal Drug Administration-approved investigational new drug status, or acknowledge that the proposed clinical application already represents routine clinical practice. This policy action is appropriate as “… the journals … ultimately bear the ethical and professional burden of promulgating only the best science.”13
Medical science has validated models to study toxicity. Westin et al.15 updated the use of the popular implanted lumbar IT catheter technique in 2010. Their report was of a preclinical model for “evaluating dose-dependent efficacy, spinal cord toxicity, and long-term function after IT morphine in the neonatal rat.” The emphasis on the preclinical period appropriately reorders the steps taken in getting a drug ready for market and a manuscript into publication quality form. The model is flexible enough such that other drugs can be tested, a wide range of postnatal ages studied, and different animal species utilized.
PRESERVATIVES AND ADJUVANTS OF HISTORICAL REPUTATION
Parabens
These compounds are added to local anesthetic preparations to provide an antibacterial effect, as in multidose vials. The methyl moieties are more common than the ethyl or propyl varieties. The common concentration is 1 mg/mL. Reichert and Butterworth note that these compounds decrease the microbe load but do not necessarily eliminate it.9 They state that there is no “convincing evidence” in the literature of a neurotoxic effect of these compounds. This is not a universal belief, as reported by Hetherington and Dooley.16 There have been reported systemic toxic effects manifested by the metabolism of parabens to paraaminobenzoic acid, which can trigger an allergic reaction in sensitive individuals.10 This reality is reenforced in a recent case report by Farber et al.,17 in which they discuss the anesthetic management of a patient with an allergy to propylene glycols and parabens. Their introduction clearly states, “multiple pharmaceutical products contain excipients, or additive chemicals, to improve stability, bioavailability, antimicrobial activity, or palatability. Two of the most common excipients are propylene glycol and parabens.” Thus, the potential for a crossreactivity reaction exists; a neurotoxic event is unlikely. Hodgson et al.7 concluded that “parabens are safe when administered spinally in the small doses associated with preservative use.”
Metabisulfite
Metabisulfite is added to epinephrine-containing local anesthetic solutions as an antioxidant. The intended effect is to increase the stability of the mixture and enhance shelf life.10 Interestingly, these compounds are also included in many emergency drug preparations such as epinephrine, procainamide, phenylephrine, dopamine, dobutamine, and dexamethasone.9 It is noted that there is a lack of sensitive and specific challenge tests, and thus the compound does infrequently provoke a hypersensitive reaction, which is characterized clinically by urticaria, flushing, pruritus, and (potentially) airway obstruction.9
As to neurotoxicity findings, studies are both mildly positive and negative. Concerns were raised about sodium bisulfite in the 1980s when 2-chloroprocaine, given unintentionally in the IT space, resulted in neural deficits.7 The explanation put forward was that the bisulfite in a low-pH (pH <4) environment was converted to sulfurous ions that could cross the dura, resulting in injurious concentrations of sulfuric acid in the cerebrospinal fluid (CSF).7,10 If the pH was >4, inactive sulfonates were formed. The issue of metabisulfite neurotoxicity has returned to the spotlight, as emphasized by Drasner.18 His editorial in 2005 preceded four articles about “new” trials of 2-chloroprocaine as a spinal anesthetic. His main point was that more careful research must precede the broad clinical application of this drug preparation as a replacement for lidocaine, with which there is a high incidence of TNSs. Taniguchi et al.19 raised an interesting point in their rat model research that compared 2-chloroprocaine with and without bisulfite for creating neurotoxicity. They concluded that clinical deficits previously associated with unintentional IT injection of 2-chloroprocaine may actually have been from the local anesthetic itself, and thus an absolute answer to the toxicity of metabisulfite remains elusive (and may indicate how difficult it is to accurately identify the precise injurious etiology, given the interplay of pharmacodynamics and pharmacokinetics of drugs placed in the body). To be most accurate, it is necessary to note that the doses of 2-chloroprocaine that caused neurotoxicity in Taniguchi et al.’s study would be equivalent to using 1 g of the drug in humans. Furthermore, as they note in their discussion, their findings of the toxicity of 2-chloroprocaine with and without bisulfite are similar to those with lidocaine, using the same experimental paradigm. That the concurrent use of bisulfite seemed to induce a neuroprotective effect casts metabisulfite in an entirely different light and certainly begs for further study.
Ethylenediaminetetraacetic Acid and Calcium Chloride
After metabisulfite had been labeled as the offending agent in 2-chloroprocaine neurotoxicity, ethylenediaminetetraacetic acid (EDTA) was substituted as the antioxidant in commercial epidural solutions of 2-chloroprocaine.10 Thus, the spinal nerves could be at potential risk if unintended doses of EDTA reached the subarachnoid space. Reichert and Butterworth9 noted that there are papers documenting a dose and a duration-dependent motor and histopathologic abnormality in animals subjected to IT EDTA (with the effect absent if calcium chloride is administered). This seems to be of only slight import now, in that EDTA is not a common additive. A systemic effect of epidural 2-chloroprocaine containing EDTA was manifested as back muscle spasm, which presented as the epidural block was resolving. It was thought at the time to be caused by the placement of some local anesthetic in the perispinal muscles with the effect that the EDTA chelated calcium, producing the spasm.20 Hung et al.21 noted that elevated extracellular calcium ion levels result in diminished nerve excitability. Using a sciatic nerve block model in rats, they showed that the addition of calcium chloride to lidocaine and bupivacaine did, indeed, produce prolonged neural blockade. However, there were histopathologic changes associated with high concentrations of CaCl2 so the authors predicted little clinical enthusiasm for such combinations in the future.
Phenylephrine
This is an additive to local anesthetics used for the purpose of decreasing the systemic blood levels of the associated local anesthetic and prolonging the duration of action of the local anesthetic.7 In addition to the use of vasoconstrictors such as phenylephrine and epinephrine, other factors that might influence the amount of local anesthetic absorbed include the specific local anesthetic agent used, its volume and concentration, and the anatomic location of its placement.22 To minimize the chance of a systemic toxic reaction, the use of vasoconstrictive agents should be carefully considered in patients with unstable angina, poorly controlled hypertension, arrhythmias, uteroplacental insufficiency, monoamine oxidase (MAO) inhibitor, or tricyclic antidepressant use, as well as in patients having intravenous regional anesthesia. The concern is not gradual systemic uptake of vasoconstrictors but immediate cardiovascular effects consequent to an unintended intravenous bolus injection.
Sakura et al.23 have reported that TNS symptoms were more frequent after tetracaine spinal anesthesia when phenylephrine was also used, but it was not clear that the symptoms were correlated with definite neurotoxicity. This conclusion was influenced by the possibility that the phenylephrine reduced the spinal cord blood flow, as had been demonstrated in animals (Chapter 12). Hodgson et al.7 cited a lack of firm data demonstrating direct neurotoxic effects from phenylephrine, as did Haider22 in his 2004 review. Both authors noted the lack of information about the possible interaction of the drugs lowering the spinal or peripheral nerve blood flow with areas of preexisting “injury” in the targeted nerve(s), leaving open the possibility of synergistic effects causing harm.
Dextrose
Dextrose is commonly used in spinal anesthesia preparations to adjust baricity, which provides some degree of directional control of the local anesthetic and thus the extent and pattern of the subsequent spinal block.9,10 Although there is some concern about the potential injury from the osmolarity,7 “Animal and human studies have largely exonerated glucose as a major contribution to neural injury.” Kalichman et al.’s24 study in diabetic rats maintains a concern that defects in glucose metabolism in the host may be a cofactor in neural injury with local anesthetics. Despite these concerns, dextrose has been used in millions of spinal anesthetics in diabetics without obvious harm.
pH Adjusters: Bicarbonate, Carbon Dioxide, HCl, NaOH
The acidic pH of commercial local anesthetics is intentional, as this maintains the stability of the solution. When clinically applied, the low-pH solution does not contain a high content of unionized local anesthetic molecules, the form of the drug that is required for penetrating the surrounding tissues and membranes prior to reaching the site of action, the sodium channel.6 There is no evidence that pH adjustment with HCl or NaOH, bicarbonate, or carbon dioxide adds to the toxicity of local anesthetic preparations.7 Although the addition of bicarbonate to commercially available local anesthetic solutions (and especially lidocaine) was touted as decreasing the block onset time, most studies have revealed this reality is more of statistical fascination than clinical benefit.25
Epinephrine
Epinephrine is added to local anesthetics for the purpose of extending block duration, limiting systemic uptake, supplementing analgesic effects, and acting as an indicator of intravascular injection. Historically, concerns have been expressed over adverse effects, including hemodynamic alterations, direct neurotoxicity, enhanced toxicity in diabetic patients, and/or vasoconstriction-induced ischemic complications (Box 8-2).7,10,22,26
BOX 8-2 Side Effects of Adjuvant Epinephrine
Hemodynamic Effects
Related to: dose, added local anesthetic, injection site.
High doses, as from unintentional intravascular injection, result in transient tachycardia, hypertension, and increased cardiac output.
Low doses, as from epidural uptake, result in decreased systemic vascular resistance and consequent increased cardiac output.
Neurotoxicity
Epinephrine does not directly cause neurotoxicity, but animal studies show that adjuvant epinephrine may worsen local anesthetic-induced neuraxial or peripheral nerve injury.
Epinephrine-induced ischemia
Animal studies and clinical surveillance studies strongly suggest that epinephrine does not adversely affect spinal cord blood flow.
Animal studies show that local anesthetics and epinephrine significantly reduce peripheral nerve blood flow, whereas patient experience suggests that this effect is clinically insignificant.
Direct Neurotoxicity of Epinephrine
Spinal cord injury does not occur following subarachnoid application of epinephrine. Single injection of up to 500 μg of epinephrine failed to cause histopathologic changes in rabbit and rat models, even if delivered in a repeated fashion.27,28 However, when combined with lidocaine 5% or tetracaine 1% to 2%, epinephrine worsens spinal cord histopathology (see Fig. 11-1).27,28 This most likely reflects decreased clearance of the local anesthetic rather than direct epinephrine-related neurotoxicity. Epinephrine applied directly to peripheral nerves does not result in tissue damage, provided the blood-nerve barrier remains intact.29,30
Epinephrine-induced Ischemia
The vasoconstrictive properties of epinephrine have led to concern that its use could provoke ischemia in the spinal cord. A wealth of clinical experience plus indirect evidence based on human survey studies strongly suggests that epinephrine is not responsible for neuraxial injury in humans (Chapter 12). Indeed, over the past 50 years, published data totaling nearly 27,000 patients document a remarkably low incidence of spinal cord injury subsequent to spinal, epidural, or combined spinal-epidural anesthetic techniques.31–36 Of those patients who did sustain injury, the vast majority did not receive adjuvant epinephrine, which further suggests that epinephrine is not causally linked to spinal cord injury in the general patient population. Whether patients with compromised blood supply, as from diabetes or atherosclerosis, are at increased risk for injury in the setting of altered spinal cord blood flow autoregulation, trauma, or local anesthetic-induced injury is unknown.37 Because such patients are often preferentially administered regional anesthesia, vast clinical experience suggests that this risk, if it exists, is exceedingly low.
Peripheral Nerve Ischemia
There is also legitimate concern that perineural epinephrine would decrease the peripheral nerve blood flow, but the clinical significance is unknown. Clinical experience suggests that the risk of neural toxicity is exceedingly low in healthy patients, whereas theoretically increased in patients at risk for compromised peripheral nerve blood flow, as from diabetes or chemotherapy. Studies suggest that epinephrine alone is not toxic to peripheral nerves in diabetic or nondiabetic rats.30 Furthermore, epinephrine added to lidocaine did not cause more damage in otherwise intact diabetic rat nerves than did lidocaine alone.30 The ischemic risk of locally applied local anesthetics with epinephrine is very low, even in so-called end-organ circulations. Despite the traditional belief that epinephrine-containing local anesthetic digital blocks could result in ischemia, a comprehensive review of reported cases found no solid evidence that this is the case.38 Limited indirect clinical evidence notes that epinephrine may worsen outcome if a peripheral nerve has been compromised by mechanical trauma. In Selander et al.’s29 report of peripheral nerve injury in patients who had also experienced a paresthesia during axillary block, all had received epinephrine. However, most studies of injury following peripheral nerve blocks have not confirmed this association.39
Thus, few specific factors increase the risk of epinephrine-associated injury. Adjuvant epinephrine in concentrations >5 μg/mL is unnecessary for clinical anesthesia applications and may theoretically increase ischemic risk. There are no human experimental studies to quantify the risk of adjuvant epinephrine in populations prone to compromised blood flow, such as diabetics, patients with atherosclerosis, or patients with chemotherapy-induced neuropathy. However, on a theoretical basis alone, it is reasonable to weigh the relative risk of adjuvant epinephrine in these patients.26
MODERN AGENTS WITH NEUROTOXICITY CONCERNS
Local Anesthetic Systemic Toxicity and Myotoxicity
The potential for local anesthetics and/or their adjuvants to cause neuraxial ischemia or peripheral nerve injury is discussed in detail in Chapters 11 and 12, and in Chapter 14, respectively. One of the most monumental advances in our specialty has been the management of LAST3–6 (Chapter 7). Given the prevalence of regional anesthesia and analgesia in current anesthesiology training and everyday practice, having such a simple and effective form of treatment for a dreaded complication is a major factor in the contemporary use of these incredibly valuable clinical techniques, which unmistakably enhance patient outcome and satisfaction.
One clinical circumstance of tissue toxicity related to the use of local anesthetics focuses on the myotoxicity of bupivacaine (Chapter 15).
Opioids, Buprenorphine
The application of neuraxial opioids has become commonplace since the sentinel reports of spinal and epidural use in 1979. The rationale was that smaller doses of these drugs placed closer in the body to the target site of action in the spinal cord would provide more intense analgesia (than systemic dosing) and a lower incidence of side effects because of the lower (than systemic) doses.39–41 That toxicity could be manifested was highlighted early on by DuPen et al.39 They were using an indwelling epidural-opioid delivery system with a preparation of morphine that contained phenol and formaldehyde. Although pain relief did result, so eventually did neuropathic pain and epidural adhesions. These complications were reversed by the use of preservative-free morphine. Sjoberg et al.40 reported on the chronic IT administration of morphine with bisulfite and bupivacaine in cancer patients with pain, associated with improved pain relief and functional status of the patients. No definitive postmortem pathologic changes of the spinal cord that were not related to the primary disease were reported. This clinical result is consistent with the general conclusion reached by Hodgson et al.7 upon a review of the literature of opioid neurotoxicity: “Laboratory studies and extensive clinical experience with morphine, fentanyl, and sufentanil can reasonably assure the safety of limited intrathecal doses of these drugs.” That said, a subsequent report by Kakinohana et al.42 generated reason for concern about a combination of factors involving neuraxial opioids that may result in injury. In a rat model, noninjurious spinal cord ischemia was created. In the presence of IT morphine, CSF glutamate increased, which activated N-methyl-D-aspartate (NMDA) receptors and resulted in the degeneration of α-motor neurons (a manifestation of neurotoxicity). Rathmell et al.43 seemed to have established the safety of IT hydromorphone in a 2005 review. This is important, as this drug is becoming a common component of acute and chronic epidural infusions.
Probably the most frequent manifestation of chronic IT opioid use/toxicity is the formation of catheter-tip granulomas. Allen et al. investigated morphine, hydromorphone, D/L-methadone, L-methadone, D-methadone, and fentanyl, among other substances in dogs, which had had IT catheters implanted.44 They reported that “opiates at equianalgesic doses present different risks for granuloma formation.” Importantly, methadone use resulted in obvious tissue necrosis as well, so the critical demand that research continues to define safe practice is evident.
In a clinical study, Candido et al.45 furthered our options by reporting on the addition of buprenorphine, an opioid agonist-antagonist, to the analgesic injectate in patients receiving an infragluteal sciatic nerve block. Although all patients receiving either perineural or intramuscular buprenorphine had less postoperative pain, it was clear that the block duration was increased only when the drug was mixed with the bupivacaine. There are no references relevant to toxicity studies in their bibliography, but the technique has been published previously.
Limited data and the infrequent application of opioids used for blocking peripheral nerves make it impossible to realistically comment on this potential toxicity.25,46 The systemic consequences of IT opioid use continue to be documented. In 2003, Rathmell et al.41 reported a dose-ranging study for IT morphine in patients after total hip and total knee arthroplasty. They found that an IT dose of 0.2 mg morphine combined with patient-controlled analgesia morphine is effective for most patients with these surgical procedures, although post-total knee replacement pain was more difficult to treat. Common opioid side effects (including pruritus, nausea, and vomiting, and oxygen desaturation events) did occur.
In the epidural application of opioids, a new product that slowly released morphine (for up to 48 hours) from a novel liposomal element represented a clinical advance. This mode of drug delivery is to be used for long-acting and controlled-release drug administration. Although not commenting on this specific liposomal vehicle, Rosenberg47 has some cautionary comments about the neuronal tissue toxicity of some of the lipids previously used in creative liposomal formulations. Rose et al.48 have also expressed concern regarding the relative lack of neurotoxicity and systemic toxicity data on extended-duration analgesic preparations intended for perineural or neuraxial administration.
Benzyl Alcohol and Polyethylene Glycol
In pain medicine practice, it has been routine that epidural steroid injections are provided as therapy for patients with radicular back pain. Traditionally, preparations of corticosteroids have been chemically engineered to behave as depot-steroids to provide benefit over an extended period of time. The preservatives benzyl alcohol and polyethylene glycol (PEG) have been part of the drugs commonly used (triamincinolone and methylprednisolone). Animal investigations and millions of therapeutic injections in humans have failed to suggest that PEG is toxic at concentrations <3%.49 The most feared concern was that arachnoiditis would result if such epidural injectates were unexpectedly deposited in the CSF. Although there is little evidence as to the harm of benzyl alcohol, Hodgson et al.7 offer the caution that all alcohols are neurotoxic at certain concentrations. This concern is also repeated by Hetherington and Dooley.16
Benzon et al.50 assessed the particle size of different steroids used in clinical practice in 2007, as a surrogate to explaining the systemic/neurotoxicity of the preparations. Using a confocal microscope, the particulate content of methylprednisolone acetate, triamcinolone acetonide, dexamethasone sodium phosphate, betamethasone sodium phosphate/betamethasone acetate, betamethasone repository (compounded), and betamethasone sodium phosphate was tabulated. The dexamethasone and betamethasone were absent of particles as they are pure liquids. “The proportion of larger particles was significantly greater in the methylprednisolone and compounded betamethasone preparations compared to the commercial betamethasone.” No direct correlation with neurotoxicity was drawn by the authors. There is a proposed relationship between particle size and vascular injury to the spinal cord in the clinical application of these preparations in transforaminal injection techniques (Chapter 28).
In a recent report in the regional anesthesia realm, Parrington et al.51 reported their findings that the addition of dexamethasone to mepivacaine prolonged the duration of analgesia after supraclavicular blocks. The complication rate did not differ in their study of only 45 adult patients, but it is hard to draw specific conclusions about the neurotoxicity of this combination from such a small study. Williams et al.26,52 advise caution in considering such use of a steroid preparation in diabetic patients, given the lack of specific studies addressing the safety of the practice and knowing that hyperglycemia can result when steroids are given to diabetics.
Clonidine and Dexmedetomidine
Clonidine is an α-2-adrenergic receptor agonist that binds to such receptors in the substantia gelatinosa and the intermediolateral cell column. The neurophysiologic consequence is to inhibit the release of substance P and the firing of wide dynamic range neurons in the spinal cord dorsal horn.53 Eisenach et al.54 demonstrated no toxicity with IT doses of clonidine up to 300 μg, and clinical analgesic effects demonstrated as a decrease in the secondary hyperalgesia of wounds by interaction with DL-alpha-amino-3-hydroxyl-5-methyl-4-isoxazole proprionic acid (AMPA) (excitatory) and gamma-amino-butyric-acid (GABA) (inhibitory) receptors. Yaksh1 also confirmed a lack of neurotoxic effects (manifested as no change in CSF or histopathology findings) in rats that had been implanted with IT catheters and received 28 days of continuous infusion of clonidine. Hodgson et al.7 found no evidence of harm from clonidine as reported in their 1999 summary article. A recent review of the clinical analgesic effects of clonidine being added to spinal local anesthetics for surgery was provided by Elia et al.55 They examined 22 randomized trials including 1,445 patients receiving clonidine mixed with bupivacaine, mepivacaine, prilocaine, or tetracaine. They reported a longer duration of block, less intraoperative pain, and more episodes of hypotension when clonidine was used with the spinal anesthetic. They noted that the optimal dose of added clonidine is still not known and do not comment on there being any evidence of tissue toxicity. Fairbanks et al.56 amplified the options for choosing an α-2 agonist additive in reporting on the solo or combination use of clonidine and dexmedetomidine in a rat model. The use of both agents produced evidence of synergistic, and not competitive, analgesia, suggesting that the two drugs result in analgesia by two distinct mechanisms of action. There was no report of obvious neurotoxicity.
Peripherally, clonidine appears to act more as a local anesthetic than as a vasoconstrictor. The addition of clonidine has more effect on the duration than the onset of local anesthetic blockade. Systemic side effects such as hypotension, sedation, and dry mouth can occur as with IT dosing.10 A recent meta-analysis of 20 randomized trials (1,054 patients) of clonidine added to lidocaine for peripheral, single-injection nerve or plexus blocks by Popping et al.57 highlights the current clinical status of this drug application. With reported doses between 30 and 300 μg, it is evident that clonidine prolongs the duration of sensory and motor block, but also increases the likelihood of hypotension and orthostatic hypotension, fainting, and sedation. The authors noted a lack of evidence of dose responsiveness for beneficial or harmful effects.
It was to be expected that studies about the combination of dexmedetomidine with local anesthetics for peripheral nerve blocks would soon appear. Brummett et al.58 studied the use of “high-dose” (meaning not clinically relevant in humans) dexmedetomidine added to bupivacaine in a rat sciatic nerve block model. Dexmedetomidine significantly increased the duration of the sensory and motor block, but only when combined with bupivacaine, and did so with no evidence of axonal or myelin injury. These results were duplicated with ropivacaine as the local anesthetic agent in a subsequent study.59
Nonsteroidal Anti-inflammatory Agents
Trauma to the body results in the release of inflammatory mediators that activate nociceptors. Prostaglandins from the breakdown of arachidonic acid are part of this cascade.53 Blockade of prostaglandins should have a potent analgesic effect, and the use of nonsteroidal anti-inflammatory agents is universal and prevalent. Furthermore, Zhu et al.60 demonstrated that cyclooxygenase-1 in the spinal cord has an important capability to modulate nociceptive input, such as that associated with postoperative pain. Korkmaz et al.61 utilized the chronic intrathecally implanted rat to study the neurotoxic effects of ketorolac given over 20 days and failed to report any significant evidence of toxicity. Eisenach et al.62 provided the logical next step in reporting the same (negative) findings in a phase I assessment of IT ketorolac in humans. This same lead investigator broadened the technique in a recent, three-part study of IT ketorolac in humans.63 The symptoms of five patients already receiving IT morphine were not helped or harmed by the addition of 0.5 to 2 mg of IT ketorolac. Twelve similar patients did not manifest a change in their pain intensity with IT saline compared to 2 mg IT ketorolac. Lastly, 30 patients undergoing vaginal hysterectomy were not distinguished by the receipt of IT saline or 2 mg IT ketorolac when measuring the time to their first request for morphine postoperatively.
Neostigmine
This acetylcholine esterase inhibitor prevents the breakdown of acetylcholine in the spinal cord, which has effects at the muscarinic receptors in the substantia gelatinosa, and results in analgesia.53 Studies cited in Thannikary and Enneking’s53 review from 2004 failed to demonstrate neurotoxicity with IT administration over 14 days. This theme is repeated in Hodgson et al.’s7 review from 5 years earlier. Kaya et al.64 have demonstrated that IT neostigmine has analgesic characteristics, but that nausea is a limiting factor clinically (as can be sedation), even with epidural administration. Joseph and McDonald25 reported that neostigmine added to peripheral nerve block mixtures offered no beneficial effects to block onset, but only a possible benefit as to block duration, yet consistent, ongoing concerns about common adverse and systemic side effects.
Ketamine
Ketamine is a phencyclidine-related analgesic that binds to gated calcium channels of the NMDA receptor, producing a noncompetitive blockade. It also binds at opioid and monoaminergic receptors and voltage-sensitive calcium channels, and thus its effects and side effects are widespread and variable.53 Preservative-free solutions of ketamine have proven to be safe for IT injection in a variety of animal models,7,53 but Errando et al.65 have shown pathologic findings after repeated dosing with IT injections of commercially available ketamine that contains benzethonium chloride. This concern is only enhanced by the recent study of Braun et al.66 They specifically investigated the adverse effects of benzethonium, the most widely used preservative in ketamine, in hematopoietic, neuronal, and glial cells. There was definite evidence of additive toxicity for ketamine, benzemethonium, and the combination of the two substances. Severe toxic damage to the spinal cord of rabbits from clinically relevant concentrations and doses of preservative-free S(+) ketamine has also been documented in a study by Vranken et al.67 A strong word of caution to clinicians about the real, adverse, and potential hazards of “ordinary anesthetic and sedative-hypnotic” drugs, especially on the developing nervous system, is aptly voiced by Drasner68 in an editorial that precedes an animal study by Walker et al.69 addressing the detrimental consequences of IT ketamine in neonatal rats. The conjoint concerns relate to apoptosis and neural degenerative changes induced by the drug.
Midazolam
In the modern era, few drugs other than local anesthetics have provoked as much commentary about toxicity as has midazolam.12,70,71 This is a water-soluble benzodiazepine, and thus it could have therapeutic potential with perineural application. In Hodgson et al.’s7 review of the neurotoxicity of IT drugs, they concluded that animal data were conflicting (as of their 1999 article), and although there were a few, small studies in humans, the data were genuinely insufficient to draw conclusions about the neural safety of the drug. The issue of neurotoxicity remained largely unsolved until Johansen et al.72 published a sufficiently sized animal toxicity study that showed a lack of classic neurotoxic effects with the drug given intrathecally. Thus, some of the angst about the conflicting studies in animals was alleviated. This study also set the scene for further discussions about the evidence of toxicity with IT midazolam in humans. Tucker et al.73 followed up 1,100 patients who had had IT midazolam. They reported no evident neurotoxicity. Yaksh and Allen71 reviewed this issue and reported in an erudite fashion the entire process by which questions about the neurotoxicity of midazolam were raised and how they should be addressed to arrive at a firm and scientific solution. The theme of more careful, scientific investigation being needed was expressed by Lavand’homme12 in an editorial that preceded the clinical report of Boussofara et al.74 about the addition of IT midazolam to a bupivacaine-clonidine mixture in 110 patients having elective lower extremity surgery. Only an increase in the duration of the motor block was a discriminating result. No neurotoxicity or data relevant to lingering clinical consequences were reported.
Amitriptyline
This classic tricyclic antidepressant has raised interest because of the local anesthetic properties attributed to its sodium channel blockade capabilities and that of voltage-gated potassium and calcium channels.75 Although it was touted as having potential as a long-acting local anesthetic, the dose-related neurotoxic effects manifested with topical application in a rat sciatic nerve model as reported by Estebe and Myers75 will likely discourage enthusiasm for the IT use of this drug. Sawynok11 identified “a significant error in the calculation of the total dose [of amitriptyline] administered” in this study in a subsequent letter to the editor. Nonetheless, the opportunity to broadcast caution about the casual use of an obviously neurotoxic drug, albeit dose related, was gained. Fukushima et al.76 investigated the potential clinical value of low doses of amitriptyline in a dog model of IT administration, but identified worrisome “intense meningeal adhesive arachnoiditis,” making it unlikely that this drug will be a spinal adjuvant. Yaksh et al.77 assessed the toxicity profiles of a number of NMDA antagonists given intrathecally, including amitriptyline, in a canine model. It was evident that IT infusions of amitriptyline, ketamine, MK801, and memantine all contributed to pathological lesions of the neural structures.
Magnesium
This ion is a noncompetitive antagonist at NMDA receptors, which are intimately involved in the CNS processing of nociceptive input. Animal studies of reasonable quality have demonstrated the tissue safety of IT applications of magnesium, referenced by Buvanendran et al.78 in 2002 in a randomized controlled trial of 52 patients who requested labor analgesia. As in animal studies, fentanyl analgesia was prolonged and no apparent signs or symptoms of neurotoxicity were manifested with the addition of magnesium to the IT injectate. There is little subsequent data upon which to alter this impression of the apparently benign effects of IT magnesium sulfate. As a matter of fact, in an induced spinal cord ischemia model, Jellish et al.79 showed that concurrent administration of magnesium sulfate in a rabbit model significantly diminished spinal cord motor neuron loss and delayed neuronal degeneration, suggesting perhaps a protective benefit of the drug.
Miscellaneous Agents
Hyaluronidase was used to facilitate the spread of local anesthetics in the past. Because this is now used only in ophthalmologic regional anesthesia, it is not reviewed here.47 Verapamil and other calcium channel blockers inhibit calcium entry into cells, thereby manifesting the ability to block nociceptive processing in the nervous system. There are too few data to comment on the neurotoxicity of these agents, just as there are for the weak opioid agonist tramadol.53 Chiari and Eisenach,80 in addressing the issue of the safety of spinally administered drugs (including somatostatin and calcitonin), stated that as of 1998 there were not enough data to qualify the safety of these agents. Eisenach et al.81 have also specifically addressed the potential application of IT adenosine. This drug produces receptor-specific analgesia, especially so at the high density of adenosine receptors in the dorsal horn. Phase I studies failed to identify toxicity with this drug.
The growing application of numerous IT agents in the practice of chronic pain medicine was highlighted by Dougherty and Staats82 in their 1999 review of this topic. One traditional drug, baclofen (used to treat spasticity), seems to be safe for IT administration given years of such use.7 Murphy et al.83 have recently reported the occurrence of a catheter-tip granuloma in a patient receiving only IT baclofen. As might be expected, a clinical investigation was initiated because this previously well-controlled patient developed a significant increase in her spasticity symptoms despite marked increases in her baclofen dosing via an IT pump. Another recent addition to the armamentarium of pain management physicians is ziconotide.84 This is a conopeptide that is used intrathecally in selected patients with chronic pain. Although its effectiveness was reviewed extensively by a study group, no specific issues about associated neurotoxicity were raised. In a view to the future, Kissin85 elaborated on the use of vanilloid agonists as adjuvants for peripheral nerve blocks in a rat sciatic nerve block model. It is exciting that initial results failed to demonstrate high levels of toxicity, so such agents may find a clinical application in regional anesthesia and analgesia.
PATHOPHYSIOLOGY
The exact mechanism of injury of adjuvant chemicals, substances, and preservative drugs is not always obvious. If we learn from the local anesthetic experience, even revered drugs of everyday use can provoke toxicity for poorly defined reasons when our ability to survey for such events becomes sophisticated enough. Obviously, a drug can have direct toxic effects on tissue, as might an acid spill on the skin. This is not the general finding with the agents discussed in this chapter. Toxicity is more likely a result of a combination of factors involving some characteristics of the primary drug, which interplay with the physical conditions present such as low pH and osmolarity, and the host’s particular preexisting pathologic conditions.
RISK FACTORS
The caution voiced by Yaksh1 about the multiplicity of untested drugs and drug combinations, all of various concentrations, that are being utilized to obtain analgesia is worth repeating. That medical science got off the usual course of orderly experimental progress and scientific rigor in the evolution of analgesic agents, adjuncts, and preservatives is evident, as highlighted in the intellectual editorials by Drasner,18 Yaksh and Allen,71 Chiari and Eisenach,80 and Lavand’homme.12 Thus, the necessary preliminary step of tissue testing for safety with a potential analgesic or adjuvant drug must include tests in a variety of animal species before human studies are initiated. We must more fully understand the toxicity of drugs so that the mistakes of the past are not repeated. It is evident that the editorial boards of our journals will hold investigators to this crucial element of scientific method.13,14
DIAGNOSIS, TREATMENT, AND PREVENTION
The diagnosis, treatment, and prevention of neuraxial and peripheral nerve injury are discussed in detail in Chapters 10, 11, 12, and 14.
SUMMARY
The pharmacology and physiology of the neurologic response continues to be investigated, and our knowledge expands day by day. When we understand the chemistry and processes involved, drugs tailored to affect specific components of that response system can be created to the benefit of patients. The process by which the neurotoxicity and systemic toxicity of drugs is determined must not be compromised in the rush to clinical application, lest patients be unexpectedly placed in harm’s way. There is little direct evidence that most of the classic adjuvants and preservatives have neurotoxic effects. Newer agents must be rigorously tested so that an appropriate balance between risk and benefit is achieved. We are witnessing the creation of reliable models for the scientific study of these matters. There are even signs that protective strategies will be forthcoming. Overall, the sophistication of our approach to neurotoxicity is progressing.
References
- Yaksh T. Preclinical models for analgesic drug study. In: Godberg AM, Zutphen LFM, eds. Alternate Methods in Toxicology and the Life Sciences. New York, NY: Mary Ann Liebert., Inc, 1995: 629–636.
- Sweeney C, Bruera E. Opioids. In: Melzack R, Wall PD, eds. Handbook of Pain Medicine. Philadelphia, PA: Churchill Livingstone, 2003:377–396.
- Drasner K. Local anesthetic systemic toxicity. A historical perspective. Reg Anesth Pain Med 2010;35:162–166.
- Di Gregorio G, Neal JM, Rosenquist RW, et al. Clinical presentation of local anesthetic systemic toxicity. A review of published cases, 1979 to 2009. Reg Anesth Pain Med 2010;35:181–187.
- Weinberg GL. Treatment of local anesthetic systemic toxicity (LAST). Reg Anesth Pain Med 2010;35:188–193.
- Morau D, Ahern S. Management of local anesthetic toxicity. Int Anesthesiol Clin 2010;48:117–140.
- Hodgson PS, Neal JM, Pollock JE, et al. The neurotoxicity of drugs given intrathecally (spinal). Anesth Analg 1999;88:797–809.
- Rowlingson JC. To avoid “transient neurologic symptoms”: the search continues (editorial). Reg Anesth Pain Med 2000;25:215–217.
- Reichert M, Butterworth J. Local anesthetic additives to increase stability and prevent organism growth. Tech Reg Anesth Pain Mang 2004;8:106–109.
- Rowlingson JC. Toxicity of local anesthetic additives. Reg Anesth 1993;18:453–460.
- Sawynok J. Amitriptyline neurotoxicity. Anesthesiology 2005;102: 240–241.
- Lavand’homme P. Lessons from spinal midazolam: when misuse of messages from preclinical models exposes patients to unnecessary risks. Reg Anesth Pain Med 2006;31:489–491.
- Neal JM, Rathmell JP, Rowlingson JC. Publishing studies that involve “off-label” use of drugs. Formalizing Regional Anesthesia and Pain Medicine’s policy. Reg Anesth Pain Med 2009;34:391–392.
- Anesthesiology information for authors. Anesthesia and Analgesia guide for authors. Anesth Analg 2009;109:217–231.
- Westin BD, Walker SM, Deumens R, et al. Validation of a preclinical spinal safety model. Effects of intrathecal morphine in the neonatal rat. Anesthesiology 2010;113:183–199.
- Hetherington NJ, Dooley MJ. Potential for patient harm from intrathecal administration of preserved solutions. Med J Aust 2000;173:141–143.
- Farber MK, Angelo TE, Castells M, et al. Anesthetic management of a patient with an allergy to propylene glycol and parabens. Anesth Analg 2010;110:839–842.
- Drasner K. Chloroprocaine spinal anesthesia: back to the future? (editorial). Anesth Analg 2005;100:549–552.
- Taniguchi M, Bollen AW, Drasner K. Sodium bisulfite: scapegoat for chloroprocaine neurotoxicity? Anesthesiology 2004;100:85–91.
- Stevens RA, Urmey WF, Urquhart BL, et al. Back pain after epidural anesthesia with chloroprocaine. Anesthesiology 1993;78:492–497.
- Hung YC, Suzuki S, Chen CC, et al. Calcium chloride prolongs the effects of lidocaine and bupivacaine in rat sciatic nerve. Reg Anesth Pain Med 2009;34:333–339.
- Haider N. Additives used to limit systemic absorption. Tech Reg Anesth Pain Mang 2004;8:119–122.
- Sakura S, Sumi M, Sakaguchi Y, et al. The addition of phenylephrine contributes to the development of transient neurologic symptoms after spinal anesthesia with 0.5% tetracaine. Anesthesiology 1997;87:771–778.
- Kalichman MW, Calcutt NA. Local anesthetic-induced conduction block and nerve fiber injury in streptozotocin-diabetic rats. Anesthesiology 1992;77:941–947.
- Joseph RS, McDonald SB. Facilitating the onset of regional anesthetic blocks. Tech Reg Anesth Pain Mang 2004;8:110–113.
- Williams BA, Murinson BB, Grable BR, et al. Future considerations for pharmacologic adjuvants in single-injection peripheral nerve blocks for patients with diabetes mellitus. Reg Anesth Pain Med 2009;34:445–457.
- Oka S, Matsumoto M, Ohtake K, et al. The addition of epinephrine to tetracaine injected intrathecally sustains an increase in glutamate concentrations in the cerebrospinal fluid and worsens neuronal injury. Anesth Analg 2001;93:1050–1057.
- Hashimoto K, Hampl KF, Nakamura Y, et al. Epinephrine increases the neurotoxic potential of intrathecally administered lidocaine in the rat. Anesthesiology 2001;94:876–881.
- Selander D, Edshage S, Wolff T. Paresthesiae or no paresthesiae? Nerve lesions after axillary blocks. Acta Anaesthesiol Scand 1979;23:27–33.
- Kroin JS, Buvanendran A, Williams DK, et al. Local anesthetic sciatic nerve block and nerve fiber damage in diabetic rats. Reg Anesth Pain Med 2010;35:343–350.
- Dripps RD, Vandam LD. Long term follow-up of patients who received 10,098 spinal anesthetics: failure to discover major neurological sequelae. JAMA 1954;156:1486–1491.
- Horlocker TT, McGregor DG, Matsushige DK, et al. Neurologic complications of 603 consecutive continuous spinal anesthetics using macrocatheter and microcatheter techniques. Anesth Analg 1997;84:1063–1070.
- Horlocker TT, McGregor DG, Matsushige DK, et al. A retrospective review of 4767 consecutive spinal anesthetics: central nervous system complications. Anesth Analg 1997;84:578–584.
- Moore DC, Bridenbaugh LD. Spinal (subarachnoid) block: a review of 11,574 cases. JAMA 1966;195:907–912.
- Vandam LD, Dripps RD. Long-term follow-up of patients who received 10,098 spinal anesthetics. IV. Neurological disease incident to traumatic lumbar puncture during spinal anesthesia. JAMA 1960;172:1483–1487.
- Hebl JR, Kopp SL, Schroeder DR, et al. Neurologic complications after neuraxial anesthesia or analgesia in patients with preexisting peripheral sensorimotor neuropathy or diabetic polyneuropathy. Anesth Analg 2006;103:1294–1299.
- Denkler K. A comprehensive review of epinephrine in the finger: to do or not to do. Plast Reconstr Surg 2001;108:114–124.
- Neal JM. Effects of epinephrine in local anesthetics on the central and peripheral nervous systems: neurotoxicity and neural blood flow. Reg Anesth Pain Med 2003;28:124–134.
- DuPen SL, Ramsey D, Chin S. Chronic epidural morphine and preservative-induced injury. Anesthesiology 1987;67:987–988.
- Sjoberg M, Karlsson PA, Nordborg C, et al. Neuropathologic findings after long-term intrathecal infusion of morphine and bupivacaine for pain treatment in cancer patients. Anesthesiology 1992;76:173–186.
- Rathmell JP, Pino CA, Taylor R, et al. Intrathecal morphine for postoperative analgesia: a randomized, controlled, dose-ranging study after hip and knee arthroplasty. Anesth Analg 2003;97:1452–1457.
- Kakinohana M, Kakinohana O, Jun JH, et al. The activation of spinal N-methyl-D-aspartate receptors may contribute to degeneration of spinal motor neurons induced by neuraxial morphine after a noninjurious interval of spinal cord ischemia. Anesth Analg 2005;100:327–334.
- Rathmell JP, Lair TR, Nauman B. The role of intrathecal drugs in the treatment of acute pain. Anesth Analg 2005;101(5 suppl): S30–S43.
- Allen JW, Horais KA, Tozier NA, et al. Opiate pharmacology of intrathecal granulomas. Anesthesiology 2006;105:590–598.
- Candido KD, Hennes J, Gonzales S, et al. Buprenorphine enhances and prolongs the postoperative analgesic effect of bupivacaine in patients receiving infragluteal sciatic nerve block. Anesthesiology 2010;113:1419–1426.
- Weller R, Butterworth J. Opioids as local anesthetic adjuvants for peripheral nerve block. Tech Reg Anesth Pain Mang 2004;8: 123–128.
- Rosenberg PH. Additives to increase tissue spread of local anesthetics. Tech Reg Anesth Pain Mang 2004;8:114–118.
- Rose JS, Neal JM, Kopacz DJ. Extended-duration analgesia: update on microspheres and liposomes. Reg Anesth Pain Med 2005;30:275–285.
- McQuillan PM, Kafiludali R, Hahn M. Interventional techniques. In: Raj PP, ed. Pain Medicine: A Comprehensive Review. 2nd ed. St. Louis, MO: Mosby, 2003:286–287.
- Benzon HT, Chew TL, McCarthy RJ, et al. Comparison of the particle sizes of different steroids and the effect of dilution. A review of the relative neurotoxicities of the steroids. Anesthesiology 2007;106:331–338.
- Parrington SJ, O’Donnell D, Chan VWS, et al. Dexamethasone added to mepivacaine prolongs the duration of analgesia after supraclavicular brachial plexus block. Reg Anesth Pain Med 2010;35:422–426.
- Williams BA. Toward a potential paradigm shift for the clinical care of diabetic patients requiring perineural analgesia; strategies for using the diabetic rodent model. Reg Anesth Pain Med 2010;35:229–232.
- Thannikary LJ, Enneking FK. Non-opioid additives to local anesthetics. Tech Reg Anesth Pain Mang 2004;8:129–140.
- Eisenach JC, De Kock M, Klimscha W. Alpha 2-adrenergic agonists for regional anesthesia: a clinical review of clonidine (1984–1995). Anesthesiology 1996;85:655–674.
- Elia N, Culebras X, Mazza C, et al. Clonidine as an adjuvant to intrathecal local anesthetics for surgery: systemic review of randomized trials. Reg Anesth Pain Med 2008;33:159–167.
- Fairbanks CA, Kitto KF, Nguyen O, et al. Clonidine and dexmedetomidine produce antinociceptive synergy in mouse spinal cord. Anesthesiology 2009;110:638–647.
- Popping DM, Elia N, Marret E, et al. Clonidine as an adjuvant to local anesthetics for peripheral nerve and plexus blocks. Anesthesiology 2009;111:406–415.
- Brummett CM, Norat MA, Palmisano JM, et al. Perineural administration of dexmedetomidine in combination with bupivacaine enhances sensory and motor blockade in sciatic nerve block without inducing neurotoxicity in rat. Anesthesiology 2008;109:502–511.
- Brummett CM, Padda AK, Amodeo FS, et al. Perineural dexmedetomidine added to ropivacaine causes a dose-dependent increase in the duration of thermal antinociception in sciatic nerve block in rat. Anesthesiology 2009;111:1111–1119.
- Zhu X, Conklin D, Eisenach JC. Cyclooxygenase-1 in the spinal cord plays an important role in postoperative pain. Pain 2003;104:15–23.
- Korkmaz HA, Maltepe F, Erbayraktar S, et al. Antinociceptive and neurotoxicologic screening of chronic intrathecal administration of ketorolac tromethamine in the rat. Anesth Analg 2004;98: 148–152.
- Eisenach JC, Curry R, Hood DD, et al. Phase I safety assessment of intrathecal ketorolac. Pain 2002;99:599–604.
- Eisenach JC, Curry R, Rauck R, et al. Role of spinal cyclooxygenase in human postoperative and chronic pain. Anesthesiology 2010;112:1225–1233.
- Kaya FN, Sahin S, Owen MD, et al. Epidural neostigmine produces analgesia but also sedation in women after cesarean delivery. Anesthesiology 2004;100:381–385.
- Errando CL, Sifre C, Moliner S, et al. Subarachnoid ketamine in swine, pathological findings after repeated doses: acute toxicity study. Reg Anesth Pain Med 1999;24:146–152.
- Braun S, Werdehausen R, Gaza N, et al. Benzethonium increases the cytotoxicity of S(+)-ketamine in lymphoma, neuronal and glial cells. Anesth Analg 2010;111:1389–1393.
- Vranken JH, Troost D, de Haan P, et al. Severe toxic damage to the rabbit spinal cord after intrathecal administration of preservative-free S(+)-ketamine. Anesthesiology 2006;105:813–818.
- Drasner K. Anesthetic effects on the developing nervous system. If you aren’t concerned, you haven’t been paying attention. Anesthesiology 2010;113:10–12.
- Walker SM, Westin D, Deumens R, et al. Effects of intrathecal ketamine in the neonatal rat. Evaluation of apoptosis and long-term functional outcome. Anesthesiology 2010;113:147–159.
- Cousins MJ, Miller RD. Intrathecal midazolam: an ethical editorial dilemma (editorial). Anesth Analg 2004;98:1507–1508.
- Yaksh TL, Allen JW. Preclinical insights into the implementation of intrathecal midazolam: a cautionary tale (editorial). Anesth Analg 2004;98:1509–1511.
- Johansen MJ, Gradert TL, Satterfield WC, et al. Safety of continuous intrathecal midazolam infusion in the sheep model. Anesth Analg 2004;98:1528–1535.
- Tucker AP, Lai C, Nadeson R, et al. Intrathecal midazolam I: a cohort study investigating safety. Anesth Analg 2004;98:1512–1520.
- Boussofara M, Carles M, Raucoules-Aime M, et al. Effects of intrathecal midazolam on postoperative analgesia when added to a bupivacaine-clonidine mixture. Reg Anesth Pain Med 2006;31: 501–505.
- Estebe JP, Myers RR. Amitriptyline neurotoxicity: dose-related pathology after topical application to rat sciatic nerve. Anesthesiology 2004;100:1519–1525.
- Fukushima FB, Barros GAM, Marques MEA, et al. The neuraxial effects of intraspinal amitriptyline at low concentrations. Anesth Analg 2009;109:965–971.
- Yaksh TL, Tozier N, Horais KA, et al. Toxicology profile of N-methyl-D-aspartate antagonists delivered by intrathecal infusion in the canine model. Anesthesiology 2008;108:938–949.
- Buvanendran A, McCarthy RJ, Kroin JS, et al. Intrathecal magnesium prolongs fentanyl analgesia: a prospective, randomized, controlled trial. Anesth Analg 2002;95:661–666.
- Jellish WS, Zhang X, Langen KE, et al. Intrathecal magnesium sulfate administration at the time of experimental ischemia improves neurological functioning by reducing acute and delayed loss of motor neurons in the spinal cord. Anesthesiology 2008;108:78–86.
- Chiari A, Eisenach JC. Spinal anesthesia: mechanisms, agents, methods, and safety. Reg Anesth Pain Med 1998;23:357–362, 384–387.
- Eisenach JC, Hood DD, Curry R. Phase I safety assessment of intrathecal injection of an American formulation of adenosine in humans. Anesthesiology 2002;96:24–28.
- Dougherty PM, Staats PS. Intrathecal drug therapy for chronic pain: from basic science to clinical practice. Anesthesiology 1999;91:1891–1918.
- Murphy PM, Skouvaklis DE, Amadeo RJJ, et al. Intrathecal catheter granuloma associated with isolated baclofen infusion. Anesth Analg 2006;102:848–852.
- Wallace MS, Rauck R, Fisher R, et al. Intrathecal ziconotide for severe chronic pain: safety and tolerability results of an open-label, long-term trial. Anesth Analg 2008;106:628–637.
- Kissin I. Vanilloid-induced conduction analgesia: selective, dose-dependent, long-lasting, with a low level of potential neurotoxicity. Anesth Analg 2008;107:271–281.
< div class='tao-gold-member'>
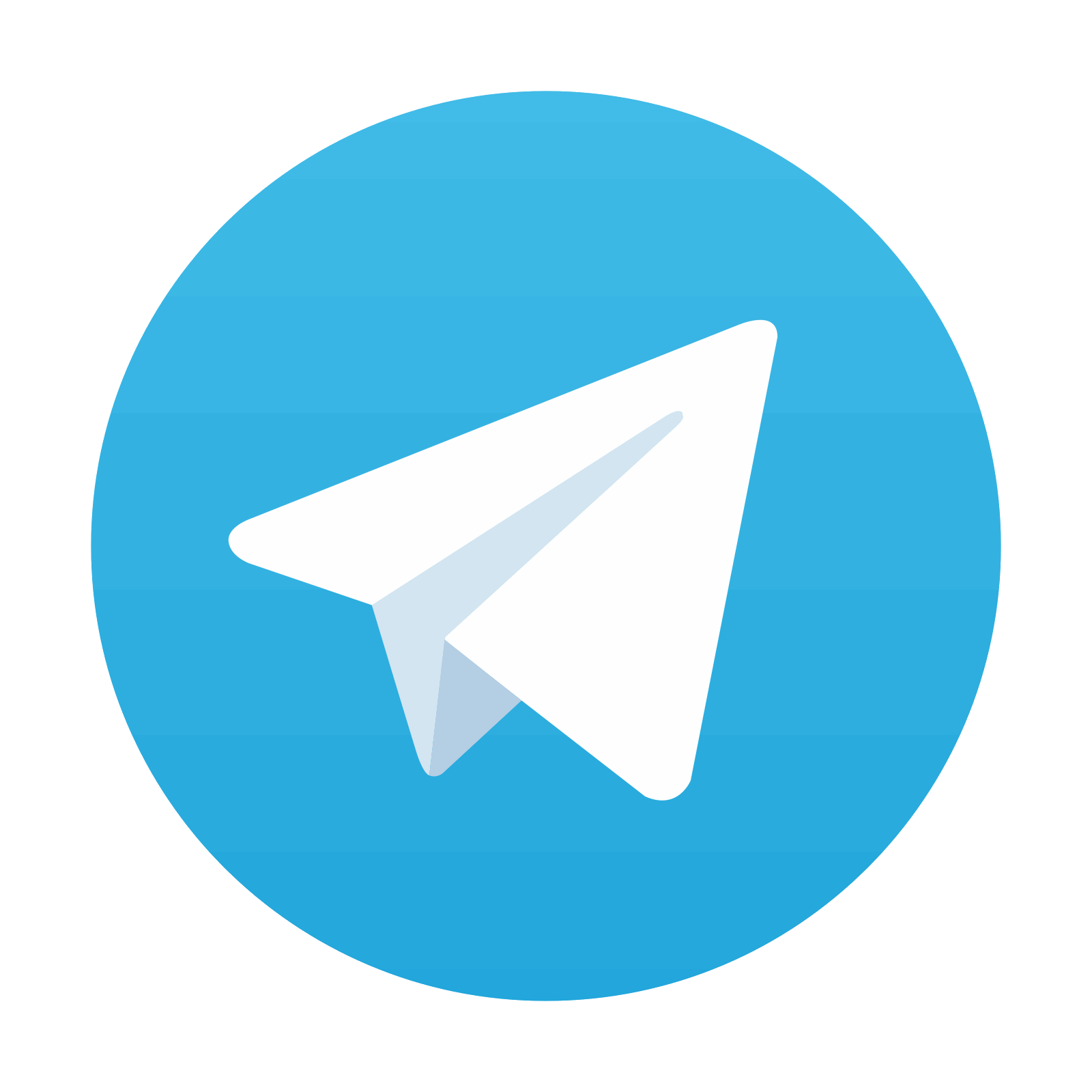