Brain adenosine triphosphate is depleted after 4 to 6 minutes of no blood flow. It returns nearly to normal within 6 minutes of starting effective cardiopulmonary resuscitation (CPR).
Through living wills and other instruments, patients have begun placing limitations on medical treatment to include do not resuscitate orders.
The major components of resuscitation from cardiac arrest are airway, breathing, circulation, drugs, and electrical therapy (ABCDE).
Two theories for the mechanism of blood flow during closed-chest compression have been suggested: cardiac pump and thoracic pump.
During CPR, myocardial perfusion is 20% to 50% of normal, whereas cerebral perfusion is maintained at 50% to 90% of normal.
CPR has limited success, with only approximately 40% of victims being admitted to the hospital and 10% surviving to discharge.
End-tidal carbon dioxide has been found to be an excellent noninvasive guide to the adequacy of closed-chest compressions.
Effective uninterrupted chest compressions and defibrillation, if appropriate, should take precedence over medications.
Although there is some evidence of improved early resuscitation in humans, there is no strong evidence that vasopressors improve long-term survival in human cardiac arrest.
After vasopressors, the drugs most likely to be of benefit during CPR are those that help suppress ectopic ventricular rhythms.
Ventricular fibrillation is the most common electrocardiogram pattern found during witnessed sudden cardiac arrest in adults.
Untreated ventricular fibrillation is a time-sensitive model with three phases: electrical, circulatory, and metabolic.
Arrest is less likely to be a sudden event and more likely related to progressive deterioration of respiratory and circulatory function in the pediatric age group.
For optimal outcome, successful restoration of spontaneous circulation must be followed by correction of reversible causes of arrest, including immediate coronary reperfusion and aggressive supportive care.
Multimedia
Adult Bradycardia Algorithm
Tachycardia Algorithm
Treatment of cardiac and respiratory arrest is an integral part of anesthesia practice. The American Board of Anesthesiology indicates in its Booklet of Information that the “clinical management and teaching of cardiac, pulmonary and neurologic resuscitation” are some of the activities that define the specialty of anesthesiology. The cardiopulmonary physiology and pharmacology that form the basis of anesthesia practice are applicable to treating the victim of cardiac arrest. However, there is specialized knowledge relating to blood flow, ventilation, and pharmacology under the conditions of a cardiac arrest that must be understood to maintain leadership of the modern cardiopulmonary resuscitation (CPR) team. This chapter concentrates on those aspects of CPR that are different from the more common circumstances requiring cardiovascular support (e.g., shock, dysrhythmias).
HISTORY
Anesthesiologists have contributed many of the elements of modern CPR and continue to be active investigators and teachers in the field. Discoveries leading to current CPR practice have a long history recorded in many famous works.1,2 The earliest reference may be the Bible story of Elisha breathing life back into the son of a Shunammite woman (2 Kings 4:34). In 1543, Andreas Vesalius3 described tracheotomy and artificial ventilation. William Harvey’s manual manipulation of the heart is well known. Early teaching of resuscitation was organized by the Society for the Recovery of Persons Apparently Drowned, founded in London in 1774. The combined techniques of modern CPR developed primarily from the fortuitous assemblage of innovative clinicians and researchers in Baltimore in the 1950s and early 1960s. Building on the long history of contributions from around the world, these investigators laid the framework for current CPR practice. In the late 1950s, mouth-to-mouth ventilation was established as the only effective means of artificial ventilation.4–7 The internal defibrillator was developed in 1933,8 but it was not applied successfully until 1947.9 It was another decade before general use was made possible by the development of external cross-chest defibrillation.10,11 Despite these advances, widespread resuscitation from cardiac arrest was not possible until Kouwenhoven et al.12 described success with closed-chest cardiac massage in a series of patients. The final major component of modern CPR was added in 1963, when Redding and Pearson13 described the improved success obtained by administering vasopressor drugs.
SCOPE OF THE PROBLEM
Cardiovascular disease remains the most common cause of death in the industrialized world. Although cardiovascular mortality has been declining in the United States since the mid-1960s, >35% of all deaths are due to cardiovascular causes.14 Of the 860,000 annual cardiovascular deaths, approximately half are related to coronary artery disease, the majority are sudden deaths and 70% occur out of the hospital or in hospital emergency departments. Thus, CPR teaching and research tend to focus on myocardial ischemia as the primary cause of cardiac arrest. However, anesthesiologists are more likely than other practitioners to deal with causes other than myocardial infarction. CPR is symptomatic therapy, aimed at sustaining vital organ function until natural cardiac function is restored. The details of effective resuscitation technique are important. However, search for a remediable cause of the arrest must not be lost in excessive attention to mechanics.
Brain adenosine triphosphate (ATP) is depleted after 4 to 6 minutes of no blood flow. It returns nearly to normal within 6 minutes of starting effective CPR. Studies in animals suggest that good neurologic outcome may be possible from 10- to 15-minute periods of normothermic cardiac arrest if good circulation is promptly restored.15,16 In clinical practice, the severity of the underlying cardiac disease is the major determining factor in the success or failure of resuscitation attempts. Of those factors under control of the rescuers, poor outcomes are associated with long arrest times before CPR is begun, prolonged ventricular fibrillation (VF) without definitive therapy, and inadequate coronary and cerebral perfusion during cardiac massage. CPR begun by bystanders can more than double survival.17 However, bystanders provide CPR only 25% to 30% of the time in sudden cardiac arrest. Optimum outcome from VF is obtained only if basic life support is begun within 4 minutes of arrest and defibrillation applied within 8 minutes.18 The importance of early defibrillation has been known for decades and is emphasized in CPR practice.19,20 What is not as well recognized is the tendency to interrupt chest compressions frequently during a resuscitation attempt. Studies of emergency medical systems suggest that chest compressions are performed <50% of the time during a typical out-of-hospital resuscitation, being interrupted for pulse checks, intubations, starting intravenous catheters, defibrillation attempts, and moving the victim.21 Because blood flow falls rapidly with cessation of compressions and resumes slowly with reinstitution of compressions, these interruptions are a major contributor to poor survival rates.
With an effective rapid-response emergency medical system, initial resuscitation rates of 40% and survival to hospital discharge of 10% to 15% are possible after out-of-hospital arrests,18,20 although the median reported survival to discharge with any first recorded rhythm is 6.4%.22 Rates for survival to discharge from in-hospital arrest are about 18% in adults and 27% in children.23 Within the hospital, the operating room is the location where CPR has the highest rate of success. Cardiac arrest occurs appro-ximately 7 times for every 10,000 anesthetics.24 The cause for the arrest is anesthesia related, approximately 4.5 times for every 10,000 anesthetics, but mortality from these arrests is only 0.4 per 10,000 anesthetics. Thus, resuscitation is successful approximately 90% of the time in anesthesia-related cardiac arrests.
ETHICAL ISSUES: DO NOT RESUSCITATE ORDERS IN THE OPERATING ROOM
Although institution of CPR is standard medical care when an individual is found pulseless, terminally ill patients have become increasingly concerned about inappropriate application of life-sustaining procedures, including CPR. Through living wills and other instruments, patients have begun placing limitations on medical treatment to include do not resuscitate (DNR) orders. Such requests are generally accepted, even welcomed, by health care workers. However, the operating room is one area of the hospital where DNR orders continue to cause ethical conflicts between medical personnel and patients.25,26 There are ethically sound arguments on both sides of the issue as to whether DNR orders should be upheld in the operating room.
The patient’s right to limit medical treatment, including refusing CPR, is firmly established in modern medical practice based on the ethical principle of respect for patient autonomy. A terminally ill patient can reject heroic measures such as resuscitation and still choose palliative therapy. If a surgical intervention will ameliorate symptoms or improve quality of life, there is no reason to withhold this treatment. Operative intervention increases the risk of cardiac arrest, and the patient may not want the burden of surviving in a worse condition than preoperatively. The possibility of death under anesthesia may be viewed as especially peaceful. Thus, the time that the DNR order provides the greatest protection against unwanted intervention is during surgery.
Despite these rather strong arguments for treating a DNR status in the operating room the same way it is treated elsewhere in the hospital, many operating room personnel are at least a little uneasy caring for these patients. Many surgeons require that DNR orders be suspended during the perioperative period or assume consent to surgery includes such suspension. There are multiple reasons for the reluctance to accept DNR status during surgery and anesthesia. Approximately 75% of cardiac arrests in the operating room are related to a surgical or anesthetic complication, and resuscitative attempts are highly successful.24 Ethically, surgeons and anesthesiologists feel responsible for what happens to patients in the operating room: primum non nocere (first, do no harm). Although the physicians are highly diligent in monitoring and managing changes in the patient’s status, complications and arrests do occur. Honoring a DNR order under these circumstances is frequently viewed as failure to treat a reversible process, and hence, tantamount to killing. This is an ethically sound view if the cause of arrest is readily identifiable and easily reversible and if treatment is likely to allow the patient to fulfill the objectives of coming to surgery.25
Institutionally, these ethical conflicts should be addressed by adoption of clear policies by hospitals.27 For the individual patient, conflicts can be resolved by communication among the patient, family, and caregivers. A mutual decision can often be reached to suspend or severely limit a DNR order in the perioperative period if the patient understands the special circumstances of perioperative arrest, that interventions are brief and usually successful, and that the physicians support the patient’s goals in coming to surgery and values in desiring not to prolong death. Many interventions commonly used in the operating room (mechanical ventilation, vasopressors, antidysrhythmics, blood products) may be considered forms of resuscitation in other situations. The only modalities that are not routine anesthetic care are cardiac massage and defibrillation. Therefore, the specific interventions included in a DNR status must be clarified with specific allowance made for methods necessary to perform anesthesia and surgery.
COMPONENTS OF RESUSCITATION
The major components of resuscitation from cardiac arrest are airway, breathing, circulation, drugs, and electrical therapy (ABCDE). Traditionally, these have been divided into basic life support (BLS) for those elements that can be performed without additional equipment—basic airway management, rescue breathing, and manual chest compressions (Fig. 58-1)—and advanced cardiac life support (ACLS), encompassing all the cognitive and technical skills necessary for resuscitation (Fig. 58-2). The lines between BLS and ACLS have tended to blur recently with the introduction of public-access automatic external defibrillators (AEDs) and the recognition that careful attention to uninterrupted, effective chest compressions improves outcome more than any advanced therapy. To stress these important elements, the American Heart Association (AHA) has adopted a simplified adult BLS algorithm as shown in Figure 58-3. In the following sections, each of the components involved in resuscitation will be reviewed separately, followed by a discussion of combining the elements for the best outcome.
FIGURE 58-1. Adult basic life support (BLS) health care provider algorithm. AED, automatic external defibrillator; CPR, cardiopulmonary resuscitation; ALS, advanced life support. (From 2010 American Heart Association Guidelines for cardiopulmonary resuscitation and emergency cardiovascular care. Circulation. 2010; 122(Suppl 3): S689, with permission.)
FIGURE 58-2. Adult advanced cardiac life support (ACLS) cardiac arrest algorithm. CPR, cardiopulmonary resuscitation; VF, ventricular fibrillation; VT, ventricular tachycardia; PEA, pulseless electrical activity; IV, intravenous(ly); IO, intraosseous(ly); ROSC, return of spontaneous circulation; PetCO2, partial pressure of end tidal carbon dioxide; J, joules. (From 2010 American Heart Association Guidelines for cardiopulmonary resuscitation and emergency cardiovascular care. Circulation. 2010;122(Suppl 3):S736, with permission.)
FIGURE 58-3. Simplified adult basic life support algorithm. (From 2010 American Heart Association Guidelines for cardiopulmonary resuscitation and emergency cardiovascular care. Circulation. 2010;122(Suppl 3):S687, with permission.)
AIRWAY MANAGEMENT
The problem of airway obstruction caused by the tongue in the unconscious patient is familiar to the anesthesiologist. The techniques used for airway maintenance during anesthesia are applicable to the cardiac arrest victim. The primary method recommended to the public is the same head tilt–chin lift method commonly employed in the operating room.28 The head is extended by pressure applied to the brow while the mandible is pulled forward by pressure on the front of the jaw, lifting the tongue away from the posterior pharynx. The jaw thrust maneuver (applying pressure behind the rami of the mandible) is an effective alternative. Properly inserted oropharyngeal or nasopharyngeal airways can be useful before intubation, recognizing the danger of inducing vomiting or laryngospasm in the semiconscious victim. Tracheal intubation provides the best airway control, preventing aspiration and allowing the most effective ventilation. However, it should not be performed until adequate ventilation (preferably with supplemental oxygen) and chest compressions have been established. A number of alternative airways designed for blind placement have been described and the laryngeal mask airway and the esophageal-tracheal Combitube (see Chapter 27) have been recommended for use during cardiac arrest by individuals who are not skilled laryngoscopists.29 When other methods of establishing an airway are unsuccessful, translaryngeal ventilation or tracheotomy by cricothyroid puncture may be necessary.
Foreign Body Airway Obstruction
In 2004, unintentional choking or suffocation accounted for 5,891 deaths in the United States (approximately 0.2% of all deaths) and 725 of the victims were <1 year old.14 Airway occlusion by a foreign object must be considered in any victim who suddenly stops breathing and becomes cyanotic and unconscious. It occurs most commonly during eating and is usually due to food, especially meat, impacting the laryngeal inlet, at the epiglottis or in the vallecula. Sudden death in restaurants from this cause is frequently mistaken for myocardial infarction, leading to the label cafe coronary. Poorly chewed pieces of food, poor dentition or dentures, and elevated blood alcohol levels are the most common factors contributing to choking. The signs of total airway obstruction are the lack of air movement despite respiratory efforts and the inability of the victim to speak or cough. Cyanosis, unconsciousness, and cardiac arrest follow quickly. Partial airway obstruction will result in rasping or wheezing respirations accompanied by coughing. If the victim has good air movement and is able to cough forcefully, no intervention is indicated. However, if the cough weakens or cyanosis develops, the patient must be treated as if there were complete obstruction.
Mothers and friends have been pounding on the backs of choking victims for centuries. In 1974, Heimlich30 proposed abdominal thrusts as a better method of relieving airway obstruction and, in 1976, Guildner et al.31 reported that sternal thrusts were just as effective. Subsequently, there were multiple studies of these maneuvers. In clinical practice, Redding32 observed that no maneuver was always successful and that each occasionally was successful when another had failed. To minimize confusion from teaching multiple techniques, the AHA has elected to emphasize the abdominal thrust maneuver (with chest thrusts as an alternative for the pregnant and massively obese).29 This recommendation is made on the twofold premise that the abdominal thrust is at least as effective as other techniques and that teaching one method simplifies education.
For the awake victim, abdominal thrusts are applied in the erect position (sitting or standing). The rescuer reaches around the victim from behind, placing the fist of one hand in the epigastrium between the xiphoid and umbilicus. The fist is grasped with the other hand and pressed into the epigastrium with a quick upward thrust. In the unconscious, thrusts are applied by kneeling astride the victim, placing the heel of one hand in the epigastrium and the other on top of the first hand. Care must be taken to ensure the xiphoid is not pushed into the abdominal contents and that the thrust is in the midline. Sternal thrusts are valuable in the massively obese or in women in advanced pregnancy. In the erect victim, the chest is encircled from behind as in the abdominal maneuver but the fist is placed in the midsternum. For the unconscious, thrusts are applied from the side of the supine victim with a hand position the same as for external cardiac compression. Back blows are applied directly over the thoracic spine between the scapulae. They must be delivered with force. Placing the victim in a head-down position (e.g., leaning over a chair) may help move the obstruction into the pharynx.
Whatever technique is used, each individual maneuver must be delivered as if it will relieve the obstruction. If the first attempt is unsuccessful, repeated attempts should be made because hypoxia-related muscular relaxation may eventually allow success. Complications of thrust maneuvers include laceration of the liver and spleen, gastric rupture, fractured ribs, and regurgitation.
In the unconscious victim, manual dislodgement of the obstruction should be tried only if solid material can be seen obstructing the airway. Grasping the object under direct visualization with a Magill forceps or ordinary instrument (e.g., ice tongs) may be used. Care must be taken not to push the foreign body deeper into the larynx. Blind finger sweeps and blind grasping with instruments are rarely successful and may cause damage to tonsils or other tissue. Finally, if the object cannot be dislodged, a cricothyroidotomy can be lifesaving.
VENTILATION
The standard approach to the unresponsive victim is to follow opening the airway with ventilation. When ventilation is provided in the rescue setting, mouth-to-mouth or mouth-to-nose ventilation is the most expeditious and effective method immediately available. Although inspired gas with this method will contain approximately 4% carbon dioxide and only approximately 17% oxygen (composition of exhaled air), it is sufficient to maintain viability.
Physiology of Ventilation During Cardiopulmonary Resuscitation
In the absence of an endotracheal tube, the distribution of gas between the lungs and stomach during positive pressure ventilation will be determined by the relative impedance to flow into each (i.e., the opening pressure of the esophagus and the lung–thorax compliance). It is likely that esophageal opening pressure during cardiac arrest is no more than that found in anesthetized individuals (approximately 20 cm water [H2O]), and lung–thorax compliance is likely reduced. To avoid gastric insufflation, inspiratory airway pressures must be kept low.
Insufflation of air into the stomach during CPR leads to gastric distention, impeding ventilation and increasing the risk of regurgitation and gastric rupture. Avoiding gastric insufflation requires that peak inspiratory airway pressures stay below esophageal opening pressure. Partial airway obstruction by the tongue and pharyngeal tissues is a major cause of increased airway pressure contributing to gastric insufflation during CPR. Meticulous attention to airway management is necessary during rescue breathing. Recommended tidal volumes to cause a noticeable rise in the chest wall in most adults is 0.5 to 0.6 L. Each rescue breath should be given over 1 second during a pause in chest compressions.
Techniques of Rescue Breathing
While maintaining an open airway with the head tilt–jaw lift technique, the hand on the forehead pinches the nose, the rescuer takes a normal breath and seals the victim’s mouth with the lips and exhales, watching for the chest to rise, indicating effective ventilation. For exhalation, the rescuer’s mouth is removed from the victim, and the rescuer listens for escaping air while taking a breath. When both hands are being used in the jaw thrust maneuver of opening the airway, the cheek is used to seal the nose. For mouth-to-nose ventilation, the rescuer’s lips surround the nose and the victim’s lips are held closed. In some patients, the mouth must be allowed to open for exhalation with this technique. Give one breath over 1 second, take a normal breath, and give a second breath over 1 second. During CPR in adults and one-rescuer CPR in children, a pause for two breaths should be made after each 30 chest compressions. When there are two rescuers with a child victim, a pause for two breaths should be made after each 15 compressions.29
Several adjuncts to ventilation are available. Perhaps the most useful adjunct is a common mask, such as that used for anesthesia. The mask can be applied to the face and held in place with the thumbs and index fingers while the other fingers are used to apply jaw thrust. Breathing into the connector port of the mask provides ventilation. Mouth-to-mask ventilation may be more aesthetic than mouth-to-mouth ventilation and can be just as effective in trained hands. Masks are also available with one-way valves that direct the victim’s exhaled gas away from the rescuer. Masks with integral nipple adapters are useful for providing supplemental oxygen. An oxygen flow of 10 L/min can raise the inspired concentration to 50%.
The self-inflating resuscitation bag and mask are the most common adjuncts used in rescue vehicles and hospitals. Although these devices have the advantages of noncontact and an ability to use supplemental oxygen, they have been shown to be difficult for a single rescuer to apply properly, preventing substantial gas leak while maintaining a patent airway.33 Tidal volumes with mouth-to-mouth and mouth-to-mask ventilation are often greater than those with the resuscitation bag. It is now recommended that if this device is to be used, two individuals manage the airway: one to hold the mask and maintain head position and one to squeeze the bag, using both hands.34 The self-inflating resuscitation bag can also be used with the laryngeal mask airway and the esophageal-tracheal Combitube.
Finally, tracheal intubation provides the best control of ventilation. With an endotracheal tube in place, breathing can proceed without concern for gastric distention or synchronizing ventilation with chest compressions. Blood flow during CPR slows rapidly when chest compressions are stopped and recovers slowly when they are resumed. Consequently, intubation should be accomplished without stopping chest compressions, if possible. Following intubation, no pause should be made for ventilation, and ventilation should be delivered without regard for the compression cycle.
CIRCULATION
Physiology of Circulation During Closed-chest Compression
Two theories of the mechanism of blood flow during closed-chest compression have been suggested.12,35 They are not mutually exclusive, and which mechanism predominates in humans continues to be debated.
Cardiac Pump Mechanism
The cardiac pump mechanism was originally proposed by Kouwenhoven et al.12 and Jude et al.36 According to this theory, pressure on the chest compresses the heart between the sternum and the spine. Compression raises the pressure in the ventricular chambers, closing the atrioventricular valves and ejecting blood into the lungs and aorta. During the relaxation phase of closed-chest compression, expansion of the thoracic cage causes a subatmospheric intrathoracic pressure, facilitating blood return. The mitral and tricuspid valves open, allowing blood to fill the ventricles. Pressure in the aorta causes aortic valve closure and coronary artery perfusion.
Thoracic Pump Mechanism
In 1976, Criley et al.37 reported a patient undergoing cardiac catheterization who simultaneously developed VF and an episode of cough-hiccups. With every cough-hiccup, a significant arterial pressure was noted. This observation of self-administered “cough CPR” prompted further investigations on the mechanism of blood flow, and these studies produced the theory of a thoracic pump mechanism for blood flow during closed-chest compressions.35 According to this theory, blood flows into the thorax during the relaxation phase of chest compressions in the same manner as that described for the cardiac pump mechanism. During the compression phase, all intrathoracic structures are compressed equally by the rise in intrathoracic pressure caused by sternal depression, forcing blood out of the chest. Backward flow through the venous system is prevented by valves in the subclavian and internal jugular veins and by dynamic compression of the veins at the thoracic outlet by the increased intrathoracic pressure. Thicker, less compressible vessel walls prevent collapse on the arterial side, although arterial collapse will occur if intrathoracic pressure is raised enough.38 The heart is a passive conduit with the atrioventricular valves remaining open during chest compression. Because there is a significant pressure difference between the carotid artery and jugular vein, blood flow to the head is favored. The lack of valves in the inferior vena cava results in less resistance to backward flow, and pressures in the arteries and veins below the diaphragm are nearly equal. This is consistent with the fact that there is little blood flow to organs below the diaphragm.39,40
It seems clear that fluctuations in intrathoracic pressure play a significant role in blood flow during CPR. It is also likely that compression of the heart occurs under some circumstances. Factors that influence the mechanism probably include the compliance and configuration of the chest wall, size of the heart, force of the sternal compressions, duration of cardiac arrest, and other undiscovered factors. Which mechanism predominates varies from victim to victim and even during the resuscitation of the same victim.
Distribution of Blood Flow During Cardiopulmonary Resuscitation
Whatever the predominant mechanism, total body blood flow (cardiac output) is reduced to 10% to 33% of normal during experimental closed-chest cardiac massage. Similar severe reductions in flow are likely during clinical CPR in humans. Nearly all the blood flow is directed to organs above the diaphragm.39,40 Myocardial perfusion is 20% to 50% of normal, whereas cerebral perfusion is maintained at 50% to 90% of normal. Abdominal visceral and lower extremity flow is reduced to 5% of normal. Total flow tends to decrease with time during CPR, but the relative distribution is not altered. Changes in CPR technique and the use of epinephrine may help sustain cardiac output over time.40 Epinephrine improves flow to the brain and heart, whereas flow to organs below the diaphragm is unchanged or further reduced.
Gas Transport During Cardiopulmonary Resuscitation
During the low flow state of CPR, excretion of carbon dioxide (CO2) (milliliters of CO2 per minute in exhaled gas) is decreased from prearrest levels to approximately the same extent that cardiac output is reduced. This reduced CO2 excretion is due primarily to shunting of blood flow away from the lower half of the body. The exhaled CO2 reflects only the metabolism of the part of the body that is being perfused. In the nonperfused areas, CO2 accumulates during CPR. When normal circulation is restored, the accumulated CO2 is washed out, and a temporary increase in CO2 excretion is seen.
Although CO2 excretion is reduced during CPR, measurement of blood gases reveals an arterial respiratory alkalosis and a venous respiratory acidosis with a markedly elevated arteriovenous CO2 difference.41 The primary cause of these changes is the severely reduced cardiac output. Two factors account for the elevation of the venous partial pressure of CO2 (PvCO2). Buffering acid causes a reduction in serum bicarbonate, so the same blood CO2 content results in a higher PvCO2. In addition, the mixed venous CO2 content is elevated. When flow to a tissue is reduced, all the CO2 produced fails to be removed and CO2 accumulates, raising the tissue partial pressure of CO2. This allows more CO2 to be carried in each aliquot of blood, and mixed venous CO2 content increases. If flow remains constant, a new equilibrium is established in which all CO2 produced in the tissue is removed but at a higher venous CO2 content and partial pressure. In contrast to the venous blood, arterial CO2 content and partial pressure (PaCO2) are usually reduced during CPR. This reduction accounts for most of the observed increase in arteriovenous CO2 content difference. Although venous blood may have an increased CO2, the marked reduction in cardiac output with maintained ventilation results in efficient CO2 removal.
Decreased pulmonary blood flow during CPR causes a lack of perfusion to many nondependent alveoli. The alveolar gas of these lung units has no CO2. Consequently, mixed alveolar CO2 (i.e., end-tidal CO2) will be low and correlate poorly with arterial CO2. However, end-tidal CO2 does correlate well with cardiac output during CPR. As flow increases, more alveoli become perfused, there is less alveolar dead space, and end-tidal CO2 measurements rise.
Technique of Closed-chest Compression
Cardiac arrest should be assumed in an unresponsive individual with abnormal or absent breathing. The community or institution emergency response system should immediately be activated and chest compressions begun. In emergency circumstances, it is difficult to detect a pulse, even in a major artery (carotid, femoral, axillary). No more than 10 seconds should be taken to check for a pulse and, if a pulse is not definitely felt, chest compressions should be started. Witnessed sudden collapse with unresponsiveness in an adult in the absence of seizure activity is nearly always dysrhythmic cardiac arrest, and chest compressions should be started immediately.
Important considerations in performing closed-chest compressions are the position of the rescuer relative to the victim, the position of the rescuer’s hands, and the rate and force of compression. The victim must be supine, the head level with the heart, for adequate brain perfusion. The victim must be on a firm surface. The rescuer should stand or kneel next to the victim’s side. Compressions are performed most effectively if the rescuer’s hips are on the same level, or slightly above the level of, the victim’s chest.
Standard technique consists of the rhythmic application of pressure over the lower half of the sternum. The heel of one hand is placed on the lower sternum, and the other hand is placed on top of the first one. Great care must be taken to avoid pressing the xiphoid into the abdomen, which can lacerate the liver. Even with properly performed CPR, costochondral separation and rib fractures are common. Applying pressure on the ribs by improper hand placement increases these complications and risks puncturing the lung. Pressure on the sternum should be applied through the heel of the hand only, keeping the fingers free of the chest wall. The direction of force must be straight down on the sternum, with the arms straight and the elbows locked into position so the entire weight of the upper body is used to apply force. Inadequate chest recoil due to leaning on the chest during the relaxation phase has been demonstrated to be both common and deleterious to effective chest compressions. During relaxation, care must be taken to remove all pressure from the chest wall, but the hands should not lose contact with the chest wall.
The sternum must be depressed at least 2 inches (5 cm) in the average adult. Occasionally, deeper compressions are necessary to generate a palpable pulse. The duration of compression should be equal to that of relaxation, and the compression rate should be at least 100 times per minute. Push hard and push fast, minimizing interruptions in chest compressions. Allow a brief pause for two 1-second breaths after every 30 compressions. With an advanced airway in place, ventilations at a rate of 8 to 10 breaths per minute should be interposed between compressions without a pause.
Alternative Methods of Circulatory Support
As currently practiced, CPR has limited success, with only approximately 40% of victims being admitted to the hospital and 10% surviving to discharge. Despite the occasional success of prolonged resuscitation, standard CPR will sustain most patients for only 15 to 30 minutes. If return of spontaneous circulation has not been achieved in that time, the outcome is dismal. Recognition of these limits and improved understanding of circulatory physiology during CPR have led to several proposals for alternatives to the standard techniques of closed-chest compression. Most, but not all, are based on the thoracic pump mechanism of blood flow. The goals of the new methods are to provide better hemodynamics during CPR and thus improve survival and/or to extend the duration during which CPR can successfully support viability. Unfortunately, none of the alternatives has proved reliably superior to the standard technique.
Simultaneous Ventilation–Compression Cardiopulmonary Resuscitation and Abdominal Binding
According to the thoracic pump theory, elevation of intrathoracic pressure during chest compression should improve blood flow and pressure.38 Studies with techniques that raise intrathoracic pressure (abdominal binding, simultaneous ventilation–compression) demonstrate that the elevated aortic pressure is offset by similar elevations in right atrial and intracranial pressures, so no improvement in myocardial or cerebral blood flow is found. Most important, survival from cardiac arrest is not improved when these techniques are compared with standard CPR in experimental animals or limited human trials.42–44
Interposed Abdominal Compression Cardiopulmonary Resuscitation
Interposed abdominal compression (IAC) is fundamentally different from abdominal binding. With this technique, an additional rescuer applies abdominal compressions manually during the relaxation phase of chest compression.45 Abdominal pressure is released when chest compression begins. One large randomized trial of out-of-hospital cardiac arrest with IAC CPR found no improvement in survival compared with standard CPR,46 but a subsequent in-hospital study demonstrated improved outcome.47 The safety of IAC CPR has been established and is recommended as an alternative to standard CPR for in-hospital resuscitation. Further studies will be needed to establish out-of-hospital efficacy.
Load-distributing Band Cardiopulmonary Resuscitation or Pneumatic Vest Cardiopulmonary Resuscitation
Following the description of “cough CPR” and the development of the thoracic pump theory, a pneumatic vest device was developed that would simulate the events of vigorous coughing.48 The technique continues to be investigated with a number of modifications from the original method. Most active investigations use a circumferential chest-compression device composed of a pneumatically or electronically actuated constricting band and backboard. In a preliminary clinical study, aortic and coronary perfusion pressure was better with load-distributing band CPR than with standard CPR.49 Additional studies are ongoing.
Active Compression–Decompression Cardiopulmonary Resuscitation
The newest proposed alternative technique developed from the anecdotal report of CPR is performed with a plumber’s helper applied to the anterior chest wall.50 This suggested that active decompression of the chest wall might reduce intrathoracic pressure during the relaxation phase of chest compressions, leading to improved venous return, increased stroke volume with compression, and better blood flow. A suction device that can be applied to the chest wall to enable active compression and decompression was developed.51 Hemodynamic studies in animals and humans with this technique have shown that coronary and cerebral perfusion may be somewhat improved with this method compared with standard CPR, although when epinephrine is used there is no difference between techniques.51,52 Clinical trials of this technique have had mixed results, with four studies showing improved outcome and five showing no positive or negative effects. No survival benefit of active compression–decompression CPR over standard CPR was found in a meta-analysis of 10 trials involving 4,162 patients in the out-of-hospital setting and in a meta-analysis of two trials involving 826 patients in the in-hospital setting.53
Impedance Threshold Device
The impedance threshold device (ITD) is a valve that impedes air entry into the lungs during chest recoil of the relaxation phase of chest compressions, thus reducing intrathoracic pressure and increasing venous return to the thorax. Originally designed to be used with a cuffed endotracheal tube and active compression–decompression CPR (during which it would act to further increase the venous return of active decompression),54 it has recently been used with conventional CPR and a tight-fitting face mask.55 Two randomized trials of out-of-hospital cardiac arrest comparing conventional CPR and the ITD with active compression–decompression CPR have shown improvement in short-term resuscitation.54,56 One randomized trial of 8,718 out-of-hospital cardiac arrest victims undergoing standard CPR with an active versus sham ITD found no difference in short-term or long-term outcomes.57 Although improved long-term survival has not been demonstrated, the ITD may be a useful adjunct for professionals trained in its use.
Invasive Techniques
In contrast to the closed-chest techniques, two invasive methods have been able to maintain cardiac and cerebral viability during long periods of cardiac arrest. In animal models, open-chest cardiac massage and cardiopulmonary bypass (through the femoral artery and vein using a membrane oxygenator) can provide better hemodynamics, as well as better myocardial and cerebral perfusion, than closed-chest techniques.58 Prompt restoration of blood flow and perfusion pressure with cardiopulmonary bypass can provide resuscitation with minimal neurologic deficit after 20 minutes of fibrillatory cardiac arrest in canines.15 However, these techniques must be instituted relatively early (probably within 20 to 30 minutes of arrest) to be effective.16,59 If open-chest massage is begun after 30 minutes of ineffective closed-chest compressions, survival is no better, although hemodynamics are improved.60 The need to apply these maneuvers early in an arrest obviously limits the application. Before invasive procedures play a greater role in modern CPR, a method must be developed to predict, early in resuscitation, which patients will and will not respond to closed-chest compressions.
Assessing the Adequacy of Circulation During Cardiopulmonary Resuscitation
The adequacy of closed-chest compression is frequently judged by palpation of a pulse in the carotid or femoral vessels. The palpable pulse primarily reflects systolic pressure. Cardiac output correlates better with mean pressure and coronary perfusion with diastolic pressure. In the femoral area, the palpable pulse is as likely to be venous as arterial. Whenever possible, more accurate means of monitoring the efficacy of chest compressions should be used. The importance of monitoring CPR quality is stressed in the new AHA ACLS circular algorithm (Fig. 58-4).
FIGURE 58-4. Advanced cardiac life support cardiac arrest circular algorithm. CPR, cardiopulmonary resuscitation; VF, ventricular fibrillation; VT, ventricular tachycardia; IV, intravenous(ly); IO, intraosseous(ly); PetCO2, partial pressure of end tidal carbon dioxide; J, joules. (From 2010 American Heart Association Guidelines for cardiopulmonary resuscitation and emergency cardiovascular care. Circulation. 2010;122(Suppl 3):S737, with permission.)
Return of spontaneous circulation with an arrested heart greatly depends on restoring oxygenated blood flow to the myocardium. In experimental models, a minimum blood flow of 15 to 20 mL/min/100 g of myocardium has been shown to be necessary for successful resuscitation.61 Obtaining such flow depends on closed-chest compressions developing adequate cardiac output and coronary perfusion pressure. Similar to the beating heart, coronary perfusion during CPR occurs primarily in the relaxation phase (diastole) of chest compressions. In 1906, Crile and Dolley62 suggested that a critical coronary perfusion pressure was necessary for successful resuscitation. This concept has been confirmed in numerous other reports.40,61–71 During standard CPR, critical myocardial blood flow is associated with aortic diastolic pressure exceeding 40 mm Hg. Because right atrial pressure can be elevated with some techniques, the aortic diastolic pressure minus the right atrial diastolic pressure is a more accurate reflection of coronary perfusion pressure. The critical coronary perfusion pressure is 15 to 25 mm Hg. When invasive monitoring is available during CPR, adjustments in chest compression technique and epinephrine should be used to ensure critical perfusion pressures are exceeded. Damage to the myocardium from underlying disease may preclude survival no matter how effective the CPR efforts. However, vascular pressures below critical levels are associated with poor results even in patients who may be salvageable (Table 58-1).
TABLE 58-1. CRITICAL VARIABLES ASSOCIATED WITH SUCCESSFUL RESUSCITATION
Although invasive pressure monitoring may be ideal, it is rarely available during CPR. End-tidal CO2 also has been found to be an excellent noninvasive guide to the adequacy of closed-chest compressions.72 CO2 excretion during CPR with an endotracheal tube in place is flow dependent rather than ventilation dependent. Because alveolar dead space is large in low-flow states, end-tidal CO2 is very low (frequently <10 mm Hg). If blood flow improves with better CPR technique, more alveoli are perfused and end-tidal CO2 rises (usually to >20 mm Hg with successful CPR). The earliest sign of return of spontaneous circulation is frequently a sudden increase in end-tidal CO2 to >40 mm Hg. Within a wide range of cardiac outputs during CPR, end-tidal CO2 correlates well with cardiac output,73 coronary perfusion pressure,74 and initial resuscitation.75 End-tidal CO2 correlates with survival in human CPR and can predict a poor outcome.76,77 Patients with end-tidal CO2 <10 mm Hg will not be resuscitated successfully. In the absence of invasive monitoring, end-tidal CO2 using quantitative waveform capnography should be used to judge the effectiveness of chest compressions, whenever possible.78 Attempts should be made to maximize the measured end-tidal CO2 by alterations in technique or drug therapy. It should be remembered that sodium bicarbonate administration liberates CO2 into the blood and causes a temporary increase in end-tidal CO2. The elevation returns to baseline within 3 to 5 minutes of drug administration and end-tidal CO2 monitoring can again be used for monitoring effectiveness of closed-chest compressions.
PHARMACOLOGIC THERAPY
This discussion of drug therapy is confined to the use of drugs during CPR attempts to restore spontaneous circulation. The use of drugs to support the circulation when there is mechanical cardiac function is discussed elsewhere (see Chapters 15 and 39). During cardiac arrest, drug therapy is secondary to other interventions. Effective uninterrupted chest compressions and defibrillation, if appropriate, should take precedence over medications. Establishing intravenous access and pharmacologic therapy should come as soon as possible but after these critical interventions are established. Although vasopressors are firmly established as improving survival in animal models and there is some evidence of improved early resuscitation in humans, there is no strong evidence that they improve long-term survival in human cardiac arrest
.79,80 The most common drugs and the appropriate adult doses are shown in Table 58-2. In addition, pharmacologic and other therapeutic approaches for the treatment of bradycardia and tachycardia are in Figures 58-5 and 58-6.
TABLE 58-2. ADULT ADVANCED CARDIAC LIFE SUPPORT DRUGS AND DOSES (INTRAVENOUS)
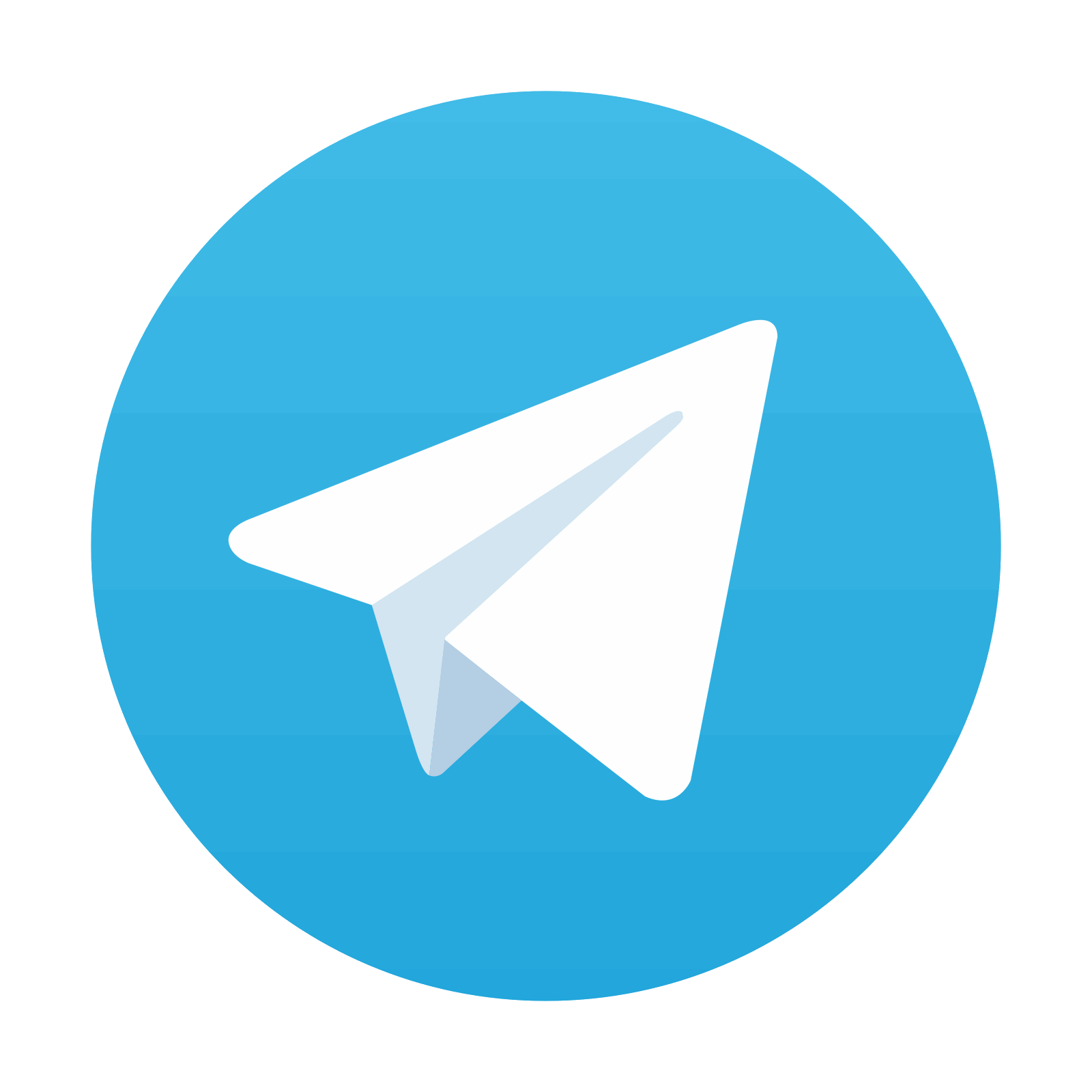
Stay updated, free articles. Join our Telegram channel

Full access? Get Clinical Tree
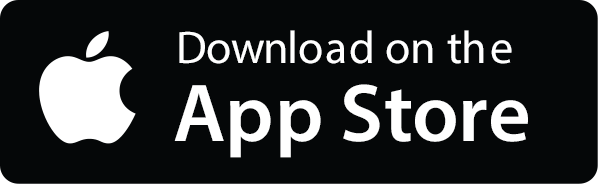
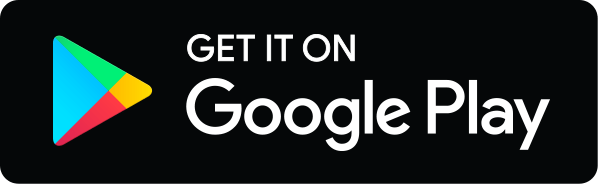