Management of the airway is paramount to safe perioperative care. Following a series of evaluation procedures affects outcomes in a favorable way.
The anatomically complex airway undergoes growth and development and significant changes in its size, shape, and relation to the cervical spine between infancy and childhood.
A thorough airway-relevant history and physical examination must be obtained during the preoperative evaluation.
Preoxygenation (also commonly termed denitrogenation) should be practiced in all cases when time permits.
The advent of the laryngeal mask airway (LMA), as well as other supraglottic airways (SGAs), has led some to question the relative safety of tracheal intubation.
Successful laryngoscopy involves the distortion of the normal anatomic planes of the SGA to produce a line of direct visualization from the operator’s eye to the larynx.
The technique of rapid-sequence induction is performed to gain control of the airway in the shortest period of time after the ablation of protective airway reflexes with the induction of anesthesia.
The period of extubation may be far more treacherous than that of induction and intubation.
Awake intubation is usually successful if approached with care and patience.
Awake airway management remains a mainstay of the American Society of Anesthesiologists’ difficult airway algorithm.
An ever-increasing number of airway management devices are commercially available.
When access to the airway from the mouth or nose fails, emergency access via the extrathoracic trachea is a feasible alternative.
Multimedia
Indirect Laryngoscopy
Airway Evaluation
Vocal Chord Polyp
Preoxygenation
Preoxygenation Errors
ETT LMA Pressures
LMA
Laryngoscopy Pitfalls
Laryngoscopy
Right Endotracheal Intubation
Colorimetric CO2 Detection
Airway Trans-Illumination
GlideScope
Channel Scopes—Airtraq
Rapid-Sequence Intubation
Aspiration
Loose Teeth
Endotracheal Extubation Procedure
Tracheostomy
O2 Dissociation Curve
Nasal Intubation
FOB
Esophageal Intubation
Bougie
Jet Ventilation
PERSPECTIVES ON AIRWAY MANAGEMENT
In the more than two decades since the publication of the first edition of this text, the field of airway management has undergone a vigorous revolution. Although the airway manager of today may still employ many of the devices available in 1988, the array of devices, algorithms, and pharmaceuticals in the modern airway armamentarium can be daunting. Fortunately, expertise in a limited, albeit complementary, number of tools as well as careful thought given to planning suffices in most cases. Although the final decade of the last century saw a resolute swing toward the application of supraglottic ventilation, a more recently introduced generation of devices reflects the application of imaging technology in the realm of tracheal intubation. The role of supraglottic airways (SGAs) is firmly established in routine anesthetic care as well as airway rescue, but the widespread adoption of video and optical laryngoscopy promises to remove many of the failings of a technique that has been in use for more than 200 years, that is direct laryngoscopy.
Techniques and practices in airway management have long been an important concern of the American Society of Anesthesiologists (ASA), as illustrated by the publication of original and revised difficult airway guidelines.1 Analysis of the Society’s closed claims database, in the periods before and after the 1993 publication of the ASA difficult airway guidelines, reveals both encouraging as well as disturbing trends.2 A significant decrease in claims related to death/brain death at the induction of anesthesia is not matched with a decrease during emergence and the postoperative period. Although the closed claims data are useful, it has significant limitations, including its retrospective and nonrandom nature, and the lack of a denominator.2
Management of the airway is paramount to safe perioperative care. Difficult and failed airway management account for 2.3% of anesthetic deaths in the United States3 and the following steps become necessary to favorably affect the outcome: (1) a thorough airway history and physical examination; (2) consideration of the ease of rapid tracheal intubation, by direct or
indirect laryngoscopy; (3) formation of management plans for the use of a supraglottic means of ventilation (e.g., face mask, SGA); (4) weighing the risk of aspiration of gastric contents; and (5) estimating the relative risk to the patient of failed airway maneuvers.4 This chapter will reflect the need to consider these five factors when approaching any patient who requires or may require airway control. This text will focus on routine and rescue airway management techniques. Specialty-specific techniques (e.g., elective suspension laryngoscopy, jet ventilation) are not included.
Review of Airway Anatomy
The term airway refers to the upper airway—consisting of the nasal and oral cavities, pharynx, larynx, trachea, and principal bronchi. The airway in humans is primarily a conducting pathway. Because the oroesophageal and nasotracheal passages cross each other, anatomic and functional complexities have evolved for protection of the sublaryngeal airway against aspiration of food that passes through the pharynx. The anatomically complex airway undergoes growth and development and significant changes in its size, shape, and relationship to the cervical spine between infancy and childhood.5
As are other bodily systems, the airway is not immune from the influence of genetic, nutritional, and hormonal factors. Table 27-1 illustrates the anatomic differences in the larynx of infants and adult.
TABLE 27-1. ANATOMIC DIFFERENCES BETWEEN THE PEDIATRIC AND ADULT AIRWAYS
The laryngeal skeleton consists of nine cartilages (three paired and three unpaired); together, these house the vocal folds, which extend in an anterior–posterior plane from the thyroid cartilage to the arytenoid cartilages. The shield-shaped thyroid cartilage acts as the anterior “protective housing” of the vocal mechanism (Fig. 27-1). Movements of the laryngeal structures are controlled by two groups of muscles: The extrinsic muscles, which move the larynx as a whole; and the intrinsic muscles, which move the various cartilages in relation to one another. The larynx is innervated by two branches of each vagus nerve: The superior laryngeal and recurrent laryngeal nerves. Because the recurrent laryngeal nerves supply all of the intrinsic muscles of the larynx (with the exception of cricothyroid muscle), trauma to these nerves can result in vocal cord dysfunction. As a result of unilateral nerve injury, airway function is usually unimpaired, although the protective role of larynx in preventing aspiration may be compromised.
FIGURE 27-1. The major landmarks of the airway mechanism. Note that the cricoid cartilage is <1 cm in height in its anterior aspect, but may be 2 cm in height posteriorly.
An important, externally identifiable structure is the membrane joining the superior aspect of the cricoid cartilage and the inferior edge of the thyroid cartilage. The cricothyroid membrane (CTM) is typically 8 to 12 mm in width and 10.4 to 13.7 mm in height in the adult, and it is composed of a yellow elastic tissue that lies directly beneath the skin and a fascial layer. It can be identified 1 to 1.5 fingerbreadths below the laryngeal prominence (thyroid notch).6 The membrane has a central portion known as the conus elasticus and two lateral thinner portions. Directly beneath the membrane is the laryngeal mucosa. Because of anatomic variability in the course of veins and arteries and the membrane’s proximity to the vocal folds (which may be 0.9 cm above the ligaments’ upper border), it is suggested that any incisions or needle punctures to the CTM be made in its inferior third and be directed posteriorly (a posterior probing needle will strike the back side of the ring-shaped cricoid cartilage). Identification of an appropriate incision or puncture site may be difficult. Elliot et al. 7 found that only 30% of anesthesiologists were able to correctly identify the skin over the CTM, in a controlled situation. It should be noted that the ASA Difficult Airway Guidelines suggest that every patient be evaluated for difficult surgical airway—this should prompt the clinician to include examination of the laryngeal structures in every patient.1
At the base of the larynx, suspended by the underside of the CTM, is the signet ring–shaped cricoid cartilage. This cartilage is approximately 1 cm in height anteriorly, but almost 2 cm in height in its posterior aspect as it extends in a cephalad direction (Fig. 27-1). Inferiorly, the trachea is suspended from the cricoid cartilage by the cricotracheal ligament. The trachea measures approximately 15 cm in adults and is circumferentially supported by 17 to 18 C-shaped cartilages, with a membranous posterior aspect overlying the esophagus.
In the adult, the first tracheal ring is anterior to the sixth cervical vertebrae. The tracheal cartilages are interconnected by fibroelastic tissue, which allows for expansion of the trachea both in length and in diameter with inspiration/expiration and flexion/extension of the thoracocervical spine. The trachea ends at the carina (opposite the fifth thoracic vertebra), where it bifurcates into the principal bronchi. The right principal bronchus is larger in diameter than the left and deviates from the plane of the trachea at a less acute angle. Aspirated materials, as well as a deeply inserted endotracheal tube (ETT), tend to gain entry into the right principal bronchus, although left-sided positioning cannot be excluded. Cartilaginous rings support the first seven generations of the bronchi.
History of Airway Management
Obstruction of the airway was a poorly understood phenomenon prior to 1874. Opening the mouth with a wooden screw and drawing the tongue forward with a forceps or a steel-gloved finger was the height of airway management.8 Recognition that the base of the tongue falling against the posterior pharyngeal wall accounted for most airway obstruction did not occur until 1880. Credit for the first use of a true SGA is given to Joseph Thomas Clover (1825–1882),9 although it is possible that devices were used toward the end of the first millennium. Clover used a nasopharyngeal tube for the delivery of chloroform anesthesia. The O’Dwyer tube was introduced in 1884. This device consisted of a curved metal conduit, with a conical end that could seal the laryngeal inlet when placed into the oropharynx. Although it was designed for the treatment of narcotic overdose, it was later modified to be used with volatile anesthetics. Over the next 50 years, several modifications of the basic oropharyngeal airway were described. In the 1930s, Ralph Waters introduced the now-familiar flattened tube oral airway. Guedel modified Waters’ concept by fitting his airway within a stiff rubber envelope in an attempt to reduce mucosal trauma.
Tracheal intubation was first described in 1788 as a means of resuscitation of the “apparently dead”,10 but it was not used for the delivery of anesthesia until almost 100 years later. The forerunner of the modern ETT was designed by the German otolaryngologist, Dr. Franz Kuhn (1866–1929). Kuhn developed a flexo-metallic tube that resisted kinking and could be shaped to the patient’s upper airway anatomy. It was inserted using a rigid stylet, and the hypopharynx was sealed with oiled gauze packing. Sir Ivan Magill and Stanley Rowbotham are credited with the initial development of modern tracheal intubation. Performing anesthesia for reconstructive facial surgery (during World War I), they developed a two-tube nasal system. One narrow tube (gum elastic design) was passed through the nares and guided into the larynx using a surgical laryngoscope. The other tube was blindly passed into the pharynx to provide for the escape of gases. During use of this “Magill” tube, the exhaust lumen would occasionally pass blindly into the larynx, leading Sir Ivan to describe “blind nasal intubation.”11
Cuffed SGAs were initially described in the early part of the 20th century. Three factors led to the development of these devices: (1) the introduction of cyclopropane (which was explosive and required an airtight circuit for appropriate gas containment), (2) the fact that blind and laryngoscopic-guided tracheal intubation remained a difficult task, and (3) a recognized need for protection of the lower airway from blood and surgical debris in the upper airway.9 The Primrose cuffed oropharyngeal tube, the Shipway airway (a Guedel oropharyngeal airway fitted with a cuff, and a circuit connector designed by Sir Ivan Magill), and the Lessinger airway were predecessors of the modern supraglottic devices. In 1937, Leech introduced a “pharyngeal bulb gasway” with a noninflatable cuff that fits snuggly into the hypopharynx.
The use of SGAs remained dominant until the introduction of curare in 1942, and the mass training of anesthesiologists in tracheal intubation in anticipation of casualties during World War II. The description by Mendelson12 of gastric-content aspiration in obstetric cases (66 of 44,016 patients, with 2 deaths) furthered the shift toward tracheal intubation in most surgical procedures. Within a few years, proficiency in direct laryngoscopy and tracheal intubation became a mark of professionalism. The advent of succinylcholine (1951) furthered the dominance of tracheal intubation by providing rapid and profound muscle relaxation.
By 1981, two types of airway management prevailed: Tracheal intubation or the anesthesia face mask/Guedel airway. Although both were time-tested, each had its failings (apart from airway failure in a small number of patients). Tracheal intubation was associated with dental and soft tissue injury as well as cardiovascular stimulation, and mask ventilation often required a hands-on-the-airway technique. These difficulties led to the reconsideration of SGAs.
In 1981, Dr. Archie Brain conceived the idea of fitting a mask-like structure over the larynx. The first prototypes of the laryngeal mask airway (LMA) were built from a Goldman dental mask, fitted with a tracheal tube. The motivation behind his early concepts and the evolution of his designs was the belief that airway control could be achieved less traumatically than tracheal intubation and more reliably than face mask ventilation. The LMA Classic was introduced into practice in the United Kingdom in 1989, and approved by the U.S. Food and Drug Administration in 1991. A variety of advanced models followed (e.g., intubation capable, gastric drainage), as will be discussed later in this chapter. With the expiration of several patents, as well as the introduction of other design concepts (e.g., noninflated masks), several devices applying the supraglottic ventilation concept were subsequently introduced.
Parallel to the development of SGA devices, the concept of indirect laryngoscopy was being increasingly considered. Although fiberoptic devices were applied to the problem of difficult tracheal intubation in the late 1960s, this technology was expensive, required a unique skill set, and was not readily available. It was not until the late 1980s that technical skill in flexible fiberoptic airway management was considered a critical anesthetic skill.13 Bullard introduced the use of a fiberoptic bundle to the rigid laryngoscope. The Bullard laryngoscope incorporated optical and light-transmitting fiberoptic bundles into an anatomically shaped blade. A detachable stylet held the tracheal tube near the optic objective, which could be placed proximal to the larynx with minimal movement of the head and neck. Though less commonly used today, the Bullard laryngoscope can be considered the vanguard of the modern video laryngoscopes and optical and video stylets.14 Fiberoptic elements were also incorporated into standard laryngoscope blades such as the Storz Endovision Video-Macintosh blade.
With the advent of inexpensive and miniaturized light-sensitive computer chip devices (i.e., CMOS), the era of video laryngoscopy was born in the first years of the 21st century. The GlideScope was the first of a generation of laryngoscopic devices to use CMOS technology to indirectly visualize the larynx. The GlideScope’s acutely angled blade placed the clinician’s point of vision around the base of the tongue without the need to establish a direct line of site from the operator’s eye to the larynx. Difficulty in placement of the ETT led to the introduction of both specialized stylets and the channeled-type laryngoscope in the late 2000s. Each of the advents will be discussed in the following text.
Limitations of Patient History and
Physical Examination
Airway management always begins with evaluation and planning. A thorough airway-relevant history must be obtained whenever possible including a search for documentation to confirm or dismiss manifest problems. It is becoming common practice for a dedicated difficult airway “note” to be incorporated into electronic medical records and for a “difficult airway letter” to be given to patients describing critical and nonanticipated events. In the absence of such documentation, the clinician should seek the anesthetic records of past surgical visits. In particularly difficult cases, this may involve contacting other institutions. In cases where this information is not available, adopting a low threshold for using the safest approach to airway management (e.g., awake intubation) will mitigate risk. This assumes that a clinician skilled in this technique is available. It is not unusual for a patient to be referred to a different facility or practitioner because of airway management issues. Signs and symptoms related to the airway should be sought (Table 27-2). Many congenital and acquired syndromes are associated with difficult airway management (Table 27-3).
TABLE 27-2. SIGNS, SYMPTOMS, AND DISORDERS WITH AIRWAY MANAGEMENT IMPLICATIONS
TABLE 27-3. SYNDROMES ASSOCIATED WITH DIFFICULT AIRWAY MANAGEMENT
Over the last two decades, several physical evaluation measures have become popularized, although their reproducibility and predictability have been disputed. The difficulty in developing the perfect airway evaluation tool lies in two interrelated areas: Simplicity and interdependency. Simple bedside evaluation tools are useful, but adequate evaluation may require endoscopic, radiologic, or other currently uncommon examinations.15,26,17 Interdependency refers to the predictive value of one airway examination measure based on the findings of another. This is discussed later in “Direct Laryngoscopy,” under the topic of functional airway assessment (FAA). Details of the various examinations and their interdependency are discussed in that section.
Historically, airway assessment has been synonymous with evaluation for the ease of direct laryngoscopy (DL), the end point being the anticipated degree of visualization of the larynx. The changing landscape of laryngoscopy (i.e., the rapid proliferation of indirect techniques including video laryngoscopy [VL]) may make many evaluation indexes obsolete or irrelevant. Efforts to define attributes that identify the difficult DL patient have been only modestly successful. Shiga et al.18 performed a meta-analysis of studies of the physical predictors of difficult DL. They concluded that when interpreted as individual test, currently used techniques of evaluation have only modest discriminative power (Tables 27-4 and 27-5).
TABLE 27-5. TECHNIQUES OF COMMON AIRWAY INDEXES MEASUREMENT
TABLE 27-4. SUMMARY OF POOLED SENSITIVITY AND SPECIFICITY OF COMMONLY USED METHODS OF AIRWAY EVALUATION
Despite the disappointing usefulness of these individual indexes in identifying the difficult to intubate patient (by DL), other authors have recognized that combinations of tests can provide improved predictability. El-Ganzouri et al.19 designed a statistical model for stratifying risk of difficult DL in a large population. This multivariate index assigned relative weights to each physical examination or historical finding based on the odds of a high-grade laryngeal view being achieved on DL. The authors noted that with increasing multivariate index scores, positive predictive value increased, but sensitivity decreased (i.e., higher multivariate index scores occur when there are more positive physical findings, but not all difficult laryngoscopy patients will manifest multiple findings). Compared with the Mallampati classification alone, the multivariate composite index had improved positive predictive and specificity values at equal sensitivity. Of course, some pathology will only present with the induction of anesthesia and/or attempts at laryngoscopy.20,21 Other groups have used similar regimens to increase the predictability of multivariate indexes by incorporating imaging technologies.22 In a small population of patients, Naguib et al. 22 were able to achieve high predictive accuracy (90% or higher) when physical examination and imaging scores were weighted. A simplified scoring system, studied on a multi-institution population of 3,763 patients, recognized both the difficulty of constructing a complex analysis in the preoperative holding area and the failure of any model to be completely predictive.23 Accepting a discriminating power of 70%, this model found five attributes that could be used to predict difficult laryngoscopy (Table 27-6).
TABLE 27-6. SIMPLIFIED RISK SCORE FOR DIFFICULT INTUABTION
Because it is a relatively new area, specific information is not yet complete on the external airway findings that may indicate failure of indirect laryngoscopy. Studies comparing DL with a Macintosh laryngoscope and video laryngoscopy with the GlideScope (Verathon, Bothell, WA) indicate that though no single examination finding may distinguish the success or failure with each device, the failure to visualize the larynx with the GlideScope was characterized by higher multivariate risk scores of the same clinical finding.24 Others have found that the following preoperative findings contribute to failure of VL: Scarring, radiation, masses or thickness of the neck, thyromental distance of <6 cm, limited cervical motion, and operator experience.25
In general, tracheal intubation should be considered nonroutine under the following conditions: (1) The presence of equally important priorities to the management of the airway (such as a full stomach or open globe), (2) abnormal airway anatomy, (3) an emergency, or (4) direct injury to the upper airway and larynx and/or trachea. Although the finding of abnormal anatomy is not necessarily synonymous with the difficult airway, it should kindle a heightened level of suspicion. Several investigators have identified anatomic features as having unfavorable influences on the mechanics of DL; these are explainable on the basis of inability to create a line of site from the operator’s eye to the aperture of the larynx.
Few studies have objectively determined those findings that identify the difficult-to-mask ventilate patient. This basic airway maneuver was examined in a control study by Langeron et al.26 Of 1,502 patients (excluding planned rapid-sequence induction or emergency cases), 5% of patients were characterized as difficult to ventilate. Only one patient in the series was unable to be ventilated by a face mask. Table 27-7 describes the criteria for defining difficult mask ventilation and the five independent clinical predictors found by Langeron et al.26 The presence of two predictors indicated a high likelihood of difficult mask ventilation. Kheterpal et al.27 investigating 53,041 patients and, using different criteria, found an impossible-to-mask ventilate incidence of 0.15%. In addition to the criteria used by Langeron et al., these authors found that a finding of a high Mallampati score, poor mandibular protrusion, a history of radiation therapy to the neck, and male gender improved the prediction of difficult mask ventilation.
Ultrasonography (US) currently has limited application in airway evaluation. Ezri et al.28,29 showed that obese patients who were difficult to intubate by direct laryngoscopy had increased pretracheal adipose (28(±2.7) mm vs. 17(±1.8) mm), mirroring Brodsky’s finding regarding neck circumference in this population. Bedside US has proven useful in other areas of airway management, including confirmation of tracheal intubation, exclusion of esophageal intubation, lung excursion (and assuring bilateral ventilation), verifying ventilation in the absence of CO2 detection, and identifying the cricoid membrane. The availability of US technology is widespread, portable to the bedside, and provides rapid, real-time, and dynamic images. These characteristics make it desirable to use in the operating room, and the promise of airway evaluation is enticing. Linear high-frequency transducers image within 2 to 3 cm of the skin surface and are the most useful probe for airway examination. Although it may be possible to examine the upper airway for changes such as hypertrophic lingual tonsils, its clinical relevancy (e.g., impact on laryngoscopy) has not been studied.29 Subglottic hemangiomas, laryngeal stenosis and cysts, and papillomas have been identified by US. Correct tracheal tube size including double-lumen tubes can be estimated based on the US image.
Predicting difficulty in DL remains, in a large part, an enigma. As previously illustrated, the commonly used indexes may not only be less predictive than originally thought, but they may also be misleading. The advent of video laryngoscopy may make these deficits irrelevant; new criteria will need to be explored.
CLINICAL MANAGEMENT OF THE AIRWAY
Preoxygenation
Preoxygenation (also commonly termed denitrogenation) should be practiced in all cases when time allows.30 This procedure entails the replacement of the nitrogen volume of the lung (as much as 95% of the functional residual capacity) with oxygen in order to provide a reservoir for diffusion into the alveolar capillary blood after the onset of apnea. Preoxygenation with 100% O2 via a tight-fitting face mask for several minutes in a spontaneously breathing patient can furnish up to 8 or more minutes of oxygen reserve following apnea (in a patient without significant cardiopulmonary disease and with a normal oxygen consumption). In one study of healthy, nonobese patients who were allowed to breathe 100% O2 preoperatively, subjects sustained an oxygen saturation of >90% for 6 ± 0.5 minutes, whereas obese patients experienced oxyhemoglobin desaturation to <90% in 2.7 ± 0.25 minutes.30 Under ideal conditions, the patient breathing room air (FIO2 = 0.21) will experience oxyhemoglobin desaturation to a level of <90% after approximately 1 to 2 minutes of apnea. Patients with pulmonary disease, or with conditions affecting metabolism or lung volumes, frequently evidence desaturation sooner, owing to increased O2 extraction, decreased functional residual capacity, or right-to-left transpulmonary shunting. The most common reason for not achieving a maximum alveolar oxygen store during preoxygenation is a loose-fitting mask, allowing the entrainment of room air.30
Time-sparing methods of preoxygenation have also been described. Using a series of four vital capacity breaths of 100% O2 over a 30-second period, a high arterial PaO2 (339 mm Hg) can be achieved, but the time to desaturation is consistently shorter as compared with traditional techniques.30 A modified vital capacity technique, wherein the patient is asked to take eight deep breaths in a 60-second period, shows promise in terms of prolonging the time to desaturation.30 The authors of the current chapter prefer the technique of applying a tight-fitting mask for 5 minutes or more of tidal volume breathing; the mask is placed immediately after the patient has been made comfortable on the operating room table, and it remains in place during intravenous catheter insertion and the application of monitors. Fresh gas flow into the mask should be a minimum of 10 to 12 L/min. Pharyngeal insufflation of oxygen has been described to delay the onset of oxyhemoglobin desaturation (<90%) during apnea.30 In this technique, oxygen is insufflated at a rate of 3 L/min via a catheter passed through the nares. This technique relies on the phenomenon of apneic oxygenation, a process by which gases are entrained into the alveolar space during apnea, in the presence of a patent airway. In the obese patient, bilevel positive airway pressure as well as the head-up position (approximately 25 degrees) have been advocated to both reach maximal preinduction arterial oxygenation and to delay oxyhemoglobin desaturation.30 Surprisingly, the head-up position may not improve the efficacy of preoxygenation in the pregnant patient.
Some circumstances can serve to decrease the effectiveness of preoxygenation . For example, the patient who experiences claustrophobia with the anesthesia face mask (which can almost always be overcome by patients holding the mask themselves) or the use of self-inflating breathing bags (which do not deliver an FIO2 of 100% during spontaneous breathing) can decrease effectiveness of preoxygenation. Likewise, leaks between the face mask and patient’s facial contours allow entrainment of air, thereby reducing the FIO2. Leaks as small as 4 mm (cross-sectional) can cause significant reductions in the inspired oxygen content.31
Support of the Airway with the Induction of Anesthesia
With the induction of anesthesia and the onset of apnea, ventilation and oxygenation must be supported by the anesthesiologist. Traditional methods include the anesthesia face mask and the ETT. During the last two decades, several SGA devices have been introduced into worldwide clinical practice. Although older data suggest that 35% or more of general anesthetics in the United States are performed with these devices, more recent data from the United Kingdom imply a 56% incidence.32,33
The Anesthesia Face Mask
The anesthesia face mask is the device most commonly used to deliver anesthetic gases and oxygen as well as to ventilate the patient who has been made apneic. The skillful use of a face mask may be challenging and, despite the many advances in airway management, remains a mainstay in the delivery of anesthesia and in resuscitation. When the induction of anesthesia is initiated, the patient’s level of consciousness changes from the awake state, with a competent and protected airway, to the unconscious state, with an unprotected and potentially obstructed airway. This drug-induced central ventilatory depression and relaxation of the musculature of the upper airway can rapidly lead to hypercapnia and hypoxia. Face mask ventilation is minimally invasive, virtually universal, and requires the least sophisticated equipment, thus making it critical to management of the airway.
The mask is gently held on the patient’s face with the left hand, leaving the right hand free for other tasks (Fig. 27-2). Air leak around the edges of the mask is prevented by downward pressure. Most modern masks can be distorted by the operator’s fingers in order to seal around the facial contours. Elastic “mask straps” may be used to help secure the mask in the awake or anesthetized patient who is breathing spontaneously and without obstruction, or to complement the left-hand grip. The mask straps can be particularly helpful for the clinician with short fingers. During a preoxygenation phase of anesthetic induction, gas leaks should be avoided; during inspiration, the patient may entrain air, limiting the efficacy of the preoxygenation maneuver.
FIGURE 27-2. Holding the anesthesia mask on the face. The thumb and the first finger grip the mask in such a fashion that the anesthesia circuit (or Ambu bag) connection abuts the web between these digits. This allows the palm of the hand to apply pressure to the left side of the mask, while the tips of these two digits apply pressure over the right. The third finger helps to secure under the mentum, and the fourth finger is under the angle of the mandible or along the lower mandibular ridge. Mask straps (on pillow) may be used to complement the hand grip by securing the right side of the mask.
In preparation for using the face mask for positive-pressure ventilation (once apnea is induced), appropriate positioning of the patient is paramount. With the patient in the supine position, the head and neck are placed in the sniffing position, which is discussed extensively later. This position improves mask ventilation by anteriorizing the base of the tongue and the epiglottis. This has been demonstrated in endoscopic studies in anesthetized patients.34
After induction of anesthesia, a tight fit of the face mask is achieved by downward displacement of the mask between the thumb and the first finger with concurrent upward displacement of the mandible with the remaining fingers. This latter maneuver, commonly known as a jaw thrust, raises the soft tissues of the anterior airway off the pharyngeal wall and allows for improved ventilation. In patients who are obese, edentulous, or bearded, two hands or a mask strap may be required to ensure a tight-fitting mask seal. When two hands are required for holding the face mask, a second operator obviously will be required in order to squeeze the reservoir bag. If necessary, the second operator can lend a third hand to the mask fitting, providing for both jaw-thrust and chin lift.
One useful, albeit poorly characterized, maneuver that can aid in face mask ventilation is the expiratory chin drop. When positive-pressure inspiration is successful, but is not followed by passive gas escape during expiration, allowing phasic head flexion and reducing chin/jaw lifting will often improve gas egress.
When a patient has presented with removable dentures, leaving the prosthetics in place can aid face mask ventilation.35
The patient with normal lung compliance should require no more than 20 to 25 cm H2O pressure to inflate the lungs. If more pressure than this is required, the clinician should re-evaluate the adequacy of the airway, adjust the mask fit, seek the aid of a second operator in order to perform two- or three-handed mask holds, and/or consider other devices that aid in the creation of an open passage for air flow through the upper airway (e.g., oral airway, SGA). Both rigid oral airways and soft nasal airways create an artificial passage to the hypopharynx. A variety of oral and nasal airways are available, but they will not be discussed in detail in this text. Nasal airways are less likely to stimulate cough, gag, or vomiting in the lightly anesthetized patient, but the risk of epistaxis must always be weighed. Nasal manipulation, including use of a nasal airway is avoided in patients at risk for epistaxis (e.g., iatrogenic or pathologic coagulopathy, pregnancy, Hereditary Hemorrhagic Telangiectasia, uncontrolled hypertension). The nasal airway is inserted along the floor of the nose, in a directly anterior–posterior direction, and should always be lubricated to reduce trauma to the nasal turbinates. Resistance to insertion should prompt repositioning of the airway bevel, reassessment of the direction of insertion forces, or change to the contralateral nare. The airway should be long enough to reach from the nare to the thyroid notch when placed alongside the face of the patient. Oral airways should likewise reach from the teeth (or alveolar ridge) to the mandibular angle. The typical rounded oral airway is placed with its longitudinal concavity rotated in a rostrad direction. Once the distal end of the airway has been inserted to the level of the oropharynx, the device is rotated 180 degrees as insertion continues, to reach its ultimate position. This maneuver avoids displacement of the tongue into the hypopharynx. A small oral aperture, an intrapharyngeal mass or foreign body, intact gag reflex or otherwise light anesthesia may prevent its placement. As will be discussed later, some intubating oral airways are large and have a rectangular cross section. These tend to be too large for intraoral rotation. They are inserted with the concavity facing caudad while the tongue is stabilized by a tongue depressor or held by the operator.
Obstruction to mask ventilation may be caused by laryngospasm, a reflex closure of the vocal folds. Laryngospasm occurs from local stimulation by a foreign body (e.g., oral or nasal airway), saliva, blood, or vomitus touching the glottis, or even a light plane of anesthesia. Hypoxia as well as noncardiogenic pulmonary edema can result if there is continued spontaneous ventilation against closed vocal cords (or other obstruction). Treatment of laryngospasm includes removal of an offending stimulus (if it can be identified), removal of secretions, continuous positive airway pressure, deepening of the anesthetic state, and the use of a rapid-acting muscle relaxant.
If there are no contraindications (e.g., a full stomach or other aspiration risk), mask ventilation can be the technique employed for the duration of anesthesia maintenance. Otherwise, it is commonly used to administer anesthetic gases until the anesthetic state is adequate for use of another means of airway support (e.g., SGA, ETT). This decision is made after careful consideration of the patient’s coexisting diseases and surgical requirements.
Supraglottic Airways
The LMA ushered in the first major use of SGAs in the United States. However, by the time of its initial introduction in 1989 and approval by the U.S. Food and Drug Administration in 1991, it was being used in more than 500 hospitals in the United Kingdom. Although initially approved for use as a substitute for face mask ventilation, and when tracheal intubation was not achievable, it soon enjoyed wide use in surgical cases traditionally managed with tracheal intubation.32 SGAs are associated with lower incidence of sore throat, coughing and laryngospasm on emergence as compared to tracheal intubation.36
The US patents on the original LMA design expired in 2002 ushering in a proliferation of similar devices. A wealth of information exists on the LMA and its subsequent iterations (all by the original inventor, Dr. Archie Brain). Much of this knowledge may be applied to newer devices. This chapter devotes a considerable amount of text to the family of LMAs. This is not meant to infer preference, but rather the availability of information. Although similar data are not available in the United States, the LMA accounts for 87% of SGA use in the United Kingdom.37
The advent of the LMA as well as other SGAs has led some to question the relative safety of tracheal intubation.38 This, along with the ASA closed claims database information, lends support to the search for safe alternatives to tracheal intubation whenever possible.39 Similarly, pharyngeal mucosal (traumatic) changes, as a result of SGA use, appear to be markedly delayed when compared with the effects of the ETT in the trachea. In one animal study, mucosal injury from the LMA ProSeal (The Laryngeal Mask Company, Jersey, UK) did not occur until more than 9 hours of continuous use.40
The LMA Classic. The LMA is composed of a small “mask” designed to sit in the hypopharynx, with an anterior surface aperture overlying the laryngeal inlet (Fig. 27-3). The rim of the mask is composed of an inflatable cuff that fills the hypopharyngeal space, creating a seal that allows positive-pressure ventilation with up to 20 cm H2O pressure. The adequacy of the seal depends on correct placement and appropriate size. It is less dependent on the cuff filling pressure or volume. Attached to the posterior surface of the mask is a barrel (airway tube) that extends from the mask’s central aperture through the mouth and can be connected to a self-inflating resuscitation bag or anesthesia circuit. Although the original mask was constructed of silicone, the single-use model, the LMA Unique (Laryngeal Mask Airway-North America, San Diego, CA), is more ubiquitous today.
FIGURE 27-3. The family of laryngeal mask airways (from top): (A) Unique, (B) flexible, (C) supreme, (D) fastrach.
LMA size selection is critical to its successful use and to the avoidance of minor as well as more significant complications. Neonatal to large adult sizes are available. The manufacturer recommends that the clinician choose the largest size that will fit comfortably in the oral cavity, which is then inflated to the minimum pressure that allows ventilation to 20 cm H2O without an air leak. The intracuff pressure should never exceed 60 cm H2O (and should be periodically monitored if nitrous oxide is used as part of the anesthetic). When an adequate seal cannot be obtained with 60 cm H2O cuff pressure, the LMA may be malpositioned and/or sizing should be re-evaluated. Light anesthesia may also contribute to poor seal or partial or complete laryngospasm.
The insertion of the LMA as described by its inventor, Dr. Archie J. I. Brain, has been modified by a number of writers. Discussion of these various alternatives is beyond the scope of this text. To understand the insertion technique, we review the processes of deglutination, which the procedure mimics: Lubrication with saliva; formation of a flat oval food bolus by the tongue; initiation of the swallowing reflex by stimulation of the palate; upward pressure by the tongue flattening the food bolus against the palate; directing of the food bolus toward the posterior pharyngeal wall and into the hypopharynx by the shape of the palate and pharyngeal wall; head extension and neck flexion, which enlarges the space behind the tongue to allow passage of the food bolus into the hypopharynx; and finally, opening of the upper esophageal sphincter to allow esophageal entry of the food bolus. These functions allow the food bolus to reach its mark blindly while avoiding the anterior pharyngeal structures and avoiding reflex responses meant to protect the airway.
The currently recommended insertion technique is illustrated in Figure 27-4, and has 98% success rate. The principles of LMA insertion can be applied to all other SGAs, though the manufacturer’s recommendations should be sought. In this technique the palatal surface of the mask is lubricated with a nonlocal anesthetic–containing lubricant (simulating the saliva), and is fully deflated to form a thin, flat wedge shape (masticated food bolus). The operator’s nondominant hand is placed under the occiput to flex the neck on the thorax and extend the head at the atlanto-occipital joint (creating a space behind the larynx; this action also tends to open the mouth).41 The index finger of the dominant hand is placed in the cleft between the mask and barrel. The hard palate is visualized and the superior (nonaperture) surface of the mask is placed against it. Force is applied by the index finger in an upward direction toward the top of the patient’s head. This will cause the mask to flatten out against the palate and follow the shape of the palate as it slides into the pharynx and hypopharynx. The index finger continues along this arc, continually applying an outward pressure until the resistance of the upper esophageal sphincter is met. The most common error made by clinicians is applying pressure with a posterior vector. This tends to catch the tip of the LMA on the posterior pharyngeal wall, causing folding with resultant misplacement and trauma.
FIGURE 27-4. Insertion of the laryngeal mask airway (LMA). The LMA is inserted with the index finger of the dominant hand pressing with a force vector against the hard palate (A and B). The outward force vector is continued from the hard palate to the pharynx and hypopharynx (C) until the index finger meets resistance against the upper esophageal sphincter and is removed (D).
Once insertion is complete, removal of the inserting hand is facilitated by gentle stabilization of the LMA barrel with the nondominant hand. Prior to attachment of the anesthesia circuit, the LMA is inflated with the minimum amount of gas to form an effective seal. Sixty centimeters of H2O pressure is the maximum suggested pilot valve pressure. Accompanying the inflation, one should be able to observe a rising of the cricoid and thyroid cartilages and lifting of the barrel out of the mouth by approximately 1 cm as the mask is lifted off the upper esophageal sphincter. If a midline position is not possible owing to the nature of the patient position or surgical procedure, a flexible LMA (discussed later) should be considered. A bite block is recommended to prevent biting and occlusion of the LMA barrel.
Although the distal tip of the LMA mask sits in the esophageal inlet, it does not reliably seal it. The LMA was not designed to protect against the aspiration of gastric contents. Despite this, when used in patients at low risk for regurgitation, the rate of aspiration during LMA use is similar to that in all non-LMA general anesthetics (approximately 2 in 10,000 cases), although the incidence of gastroesophageal reflux may be increased when compared with use of the face mask.42
If regurgitated gastric contents are noted in the LMA barrel, maneuvers similar to those applied when using an ETT should be instituted: Patient in Trendelenburg position, administer 100% oxygen, leave the LMA in place and use a flexible suction device down the barrel, and if necessary, deepen the anesthetic.
When populations of patients considered to have a full stomach are studied (in controlled trials, prospective series, or anecdotally), there is a low incidence of aspiration noted with elective or emergency LMA use. Reports have included patients who are morbidly obese or experience frequent gastroesophageal reflux, those undergoing elective cesarean section or airway rescue during labor, and those presenting to emergency departments or paramedic crews.43,44 During cardiopulmonary resuscitation, the incidence of gastroesophageal regurgitation is four times greater with a bag-valve mask than with the LMA.45
Although first introduced for use with spontaneous ventilation, the LMA has proved useful for cases in which positive-pressure ventilation is either desired or preferred.46 Contrary to initial impression, positive-pressure ventilation can be safely accomplished with the LMA.47 There is no difference found in gastric inflation with positive pressure (<17 cm H2O) when comparing the LMA and the ETT.48 When using the LMA Classic, one should limit tidal volumes to 8 mL/kg and airway pressure to 20 cm H2O. LMA use has been described with supine, prone, lateral, oblique, Trendelenburg, and lithotomy positions. Although the manufacturer recommends use for a maximum of 2 to 3 hours, reports of use for more than 24 hours can be found.49 Although no studies have examined histologic changes in the mucosa with prolonged LMA use, Goldmann et al. 50 saw no evidence of mucosal changes with up to 9 hours of ProSeal LMA use, though damage was consistently evident after 12 hours.
The LMA Flexible. The introduction of the LMA Flexible (The Laryngeal Mask Company, Jersey, UK) (Fig. 27-3) has permitted extension of LMA use to a variety of cases in which the airway is shared with the surgical team (e.g., otolaryngologic surgery) or within the surgical field (e.g., ophthalmologic surgery). The LMA Flexible differs from the original design by virtue of a thin-walled, small-diameter, wire-reinforced (kink-resistant) barrel, which can be positioned out of the midline without affecting the hypopharyngeal position of the mask. It was designed to be used with a tonsillar mouth gag employed in surgery on the mouth and pharynx.51 The LMA Flexible has also proved useful when heavy drapes are placed over the head and airway (e.g., mastoidectomy, ophthalmic procedures), when there is movement of the head position during surgery (e.g., tympanostomy tubes), or when the LMA barrel cannot be secured in the midline (e.g., mid or lateral facial surgery). The use of this mask in surgery above the level of the hypopharynx, including tonsillectomy, affords a number of clinically important advantages over tracheal intubation (Table 27-8). When correctly placed, the LMA mask serves to better block the airway from blood, secretions, and surgical debris above the level of the mask, as compared with the tracheal tube, which is known to not protect the trachea from liquids instilled into the pharynx.47
TABLE 27-8. ADVANTAGES OF THE LARYNGEAL MASK AIRWAY IN SUPRAGLOTTIC SURGERY
The SGA and Bronchospasm. SGAs appear to be well suited to the patient with a history of bronchospasm. SGAs present a unique opportunity for the clinician to conveniently and effectively control the airway without having to introduce a foreign body into the trachea. Thus, it may be an ideal airway tool in the asthmatic patient who is not at risk for reflux and aspiration.52 Because the halogenated inhaled anesthetics are potent bronchodilators, it is at the time of emergence, when the anesthetic is discontinued, that the patient at risk for bronchospasm is most likely to wheeze. In the patient managed with an SGA, there is no foreign body in the sensitive bronchorespiratory tree and the patient can be fully emerged prior to removal of the device. In the event that uncontrollable bronchospasm does occur intraoperatively (e.g., from vagal stimuli such as traction on the peritoneum), intubation can be performed through many SGAs or after removal. When tracheal intubation is mandatory (for the surgical procedure) yet concerns regarding bronchospasm exist, the Bailey maneuver is employed.53 In this maneuver, the deflated LMA is placed behind the in situ ETT. The ETT is removed and the LMA is inflated. The patient is then emerged on the LMA.
SGA Removal. Timing of the removal of SGAs at the end of surgery is critical.54 SGAs should be removed either when the patient is deeply anesthetized or after protective reflexes have returned and the patient is able to open the mouth on command. Removal during excitation stages of emergence can be accompanied by coughing and/or laryngospasm. Many clinicians remove the LMA fully inflated; thus, it acts as a “scoop” for secretions above the mask, bringing them out of the airway.55 This has been particularly useful in otolaryngologic surgery.
Contraindications to SGA Use. The primary contraindication to elective use of the SGA is a risk of gastric-content aspiration (e.g., full stomach, hiatus hernia with significant gastroesophageal reflux, intestinal obstruction, delayed gastric emptying, poor history). Other contraindications include poor lung compliance or high airway resistance, glottic or subglottic airway obstruction, and limited mouth opening (<1.5 cm).56
SGA Use Complications. Apart from gastroesophageal reflux and aspiration, reported complications have included laryngospasm, coughing, gagging, retching, bronchospasm, and other events characteristic of airway manipulation. The incidence of SGA-induced postoperative sore throat varies from 4% to 50% and is highly dependent on the study methods. No single device shows a consistently lower rate of dysphagia, though all appear to be better than tracheal intubation in this regard with expected rates of 30% to 70%.57 SGAs may cause transient changes in vocal cord function. This is possibly related to cuff overinflation during prolonged procedures.
There have been rare reports of nerve injury associated with SGA use, including recurrent hypoglossal, lingual, and inferior alveolar nerves. The injuries are typically manifest from emergence to 48 hours after surgery and the majority resolved spontaneously in 1 hour to 18 months. Predisposing factors include the use of small masks, nitrous oxide, lidocaine lubrication, cuff overinflation, difficult or alternate insertion techniques, and cervical bone or joint disease.58 Pressure neuropraxia from the tube or cuff is the most common cause.
The Laryngeal Tube (VBM Medizintechnik, GmbH, Sulz, Germany) consists of a single-lumen tube with an approximately 130-degree midshaft angle and two (distal and proximal) low-pressure cuffs (Fig. 27-5A). An oval aperture between the cuffs serves as a ventilation orifice. The distal cuff encloses the distal end of the tube. When inserted correctly, the proximal cuff seals the oral and nasal pharynx, whereas the distal cuff sits within the upper esophageal sphincter. Ventilation (spontaneous or positive pressure) occurs via an anterior surface orifice midway between the cuffs. The cuffs are inflated via a common pilot valve. The original Laryngeal Tube is available in single-use or reusable models, requires a mouth opening of at least 2.3 cm, and is inserted either blindly or with the aid of a laryngoscope. The Laryngeal Tube Suction is a modification of the Laryngeal Tube, with the addition of a second lumen for suction and gastric drainage (the orifice of which is at the distal aspect of the esophageal cuff). Six sizes (0 to 5) are suitable for neonates to large adults. Using the Laryngeal Tube in children under 10 years old is less effective than the LMA during spontaneous or assisted ventilation and for fiberoptic evaluation of the airway.59 The Laryngeal Tube is not recommended for children weighing <10 kg because of technical difficulties and inadequate ventilation. A successful use of the Laryngeal Tube Suction was reported in an emergency airway situation in a pregnant woman with history of gastroesophageal reflux who underwent cesarean section but could not be intubated. The device improved oxygen saturation and drained gastric contents during the patient’s emergence from rapid-sequence intubation.60
FIGURE 27-5. (A) The laryngeal tube, (B) I-Gel, (C) Air-Q, (D) Aura-I.
A study in 15 fresh cadavers showed that mucosal pressures in the lateral pharynx, the base of tongue, and the posterior pharynx were similar between Laryngeal Tube and LMA ProSeal, but pressure on the posterior hypopharynx was always higher with the Laryngeal Tube. The investigators expressed concern that pressure from the Laryngeal Tube might impede pharyngeal perfusion more than LMA ProSeal.61 A case of acute tongue and uvula ulceration after using the Laryngeal Tube for hysteroscopy has been reported.62
Cookgas Air-Q Airway. Developed by Dr. Daniel Cook (Cookgas LLC, St Louis, MO), the Air-Q perilaryngeal airway functions as an elective SGA, or as a conduit for blind or fiberoptic-aided intubation of the trachea (Fig. 27-5C). The barrel of the airway is precurved and of wide diameter, and will accept a tracheal tube from 5- to 8-mm internal diameter (ID). The keyhole-shaped airway outlet is designed to steer the ETT toward the larynx. A cuff, grossly the shape of the LMA cuff, seals the perilaryngeal space. The device is inserted (cuff deflated) by a technique similar to that recommended for the LMA (see the previous discussion). If, after insertion, the airway is obstructed, an up–down motion of the barrel will often realign the epiglottis. Blind tracheal intubation should be undertaken only if the airway is clear and the patient is muscle relaxed and/or sufficiently anesthetized. Airway seal pressures are similar to that of the ProSeal LMA.63 The ETT cuff is completely deflated and lubricated. It is inserted 12 to 15 cm into the device barrel. Advancement past this point will be into the larynx. If resistance is met, the device can be repositioned. Once tracheal intubation is assured, the device can be removed with the help of a specialized stylet marketed by the manufacturer. In a study comparing like devices, the Fastrach LMA had a higher blind intubation rate as compared to the Air-Q, although all patients could be intubated with the addition of fiberoptic guidance.64
The Double-lumen SGAs. The LMA Proseal was developed by Dr. Brain as the first double-lumen SGA. The original intent of the LMA Proseal’s gastric drain was to aid the clinician in the diagnosis of SGA malposition—if not placed correctly with the device’s distal tip within the opening of the esophagus, an air leak would be detected. Several tests were developed to verify positioning with the LMA Proseal (Table 27-9).65–68 The LMA ProSeal also increases the maximum airway seal during positive-pressure ventilation as compared with other LMA devices (≥40 cm H2O), and allows passive (regurgitation) and active (gastric tube insertion) emptying of the stomach.66–68 The advanced capabilities of the LMA Proseal allowed its use in the care of obese patients, patients undergoing intra-abdominal procedures, and in airway resuscitation.41,69,70 A single-use version of the double-lumen LMA, the LMA-Supreme also incorporated a fixed curvature replicating the insertion ease of the LMA Fastrach (Fig. 27-3C). This device also allowed inspiratory pressures of >35 cm H2O, and has been used for intra-abdominal procedures.71
TABLE 27-9. FEATURES OF THE LARYNGEAL MASK AIRWAY PROSEAL
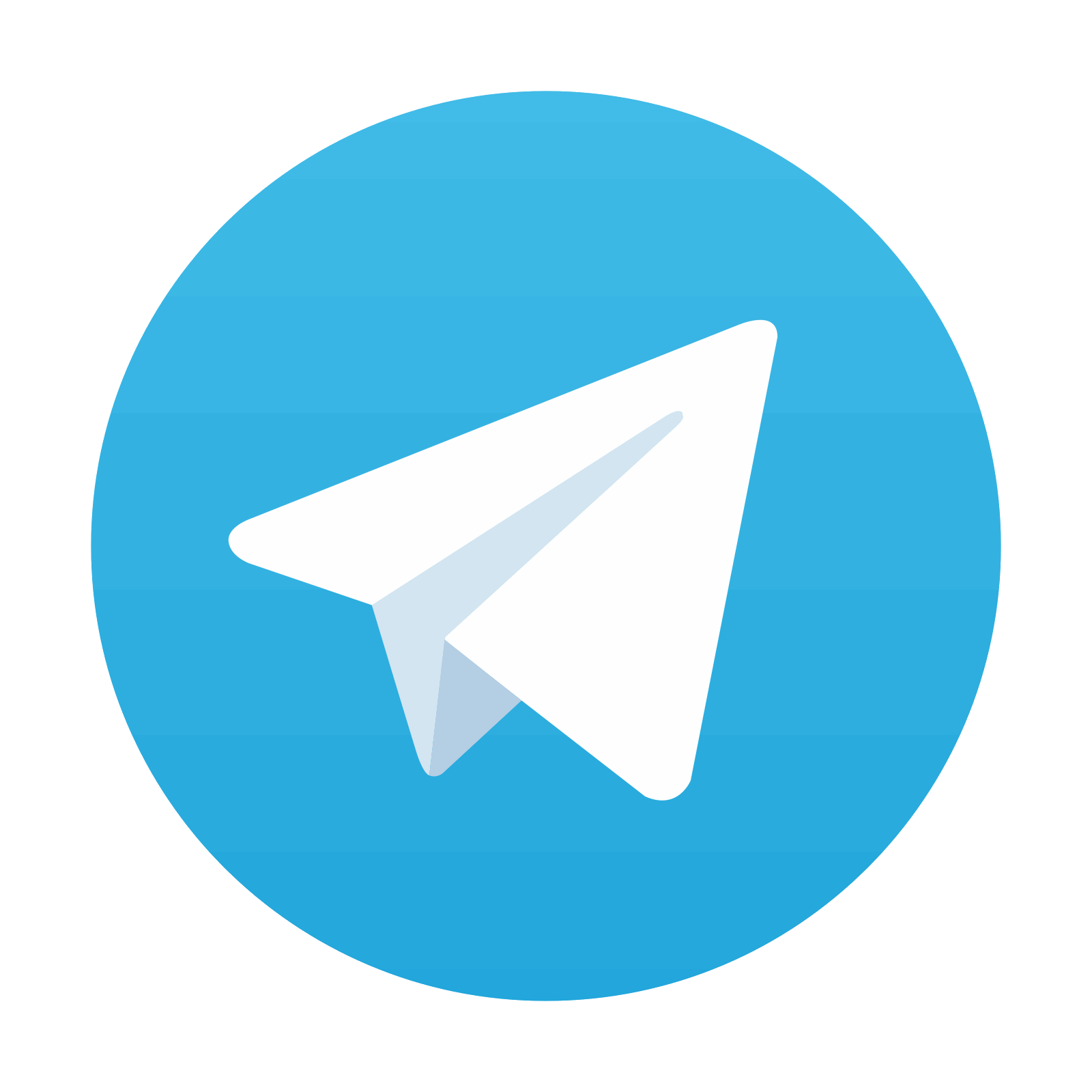
Stay updated, free articles. Join our Telegram channel

Full access? Get Clinical Tree
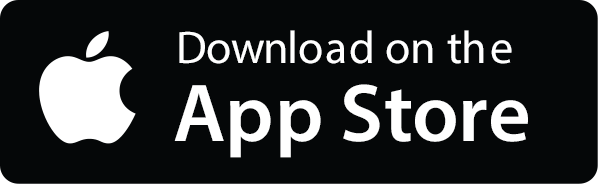
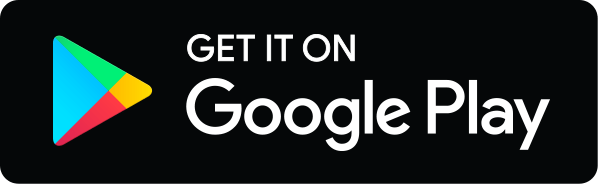