AKIN Criteria
Because of the limitations of the RIFLE criteria just mentioned, revised criteria were introduced by the Acute Kidney Injury Network (AKIN), and these criteria are shown at the bottom of Table 34.1. The AKIN criteria require a smaller change in creatinine (≥0.3 mg/dL) for the diagnosis of AKI, and a time limit of 48 hours is imposed on the change in serum creatinine. Unfortunately, the RIFLE criteria were not abandoned after introduction of the AKIN criteria, so there are two competing systems for the diagnosis and classification of AKI at the present time.
What Now?
So, which criteria should be used to diagnose and stage AKI? The AKIN criteria seem to be favored in published reviews, but comparison studies have not demonstrated a difference between the RIFLE and AKIN criteria for predicting outcomes. This is demonstrated in Figure 34.1, which shows that the two sets of criteria are equivalent for predicting mortality rates (5).
Sources of Confusion
Despite the intent of simplifying the approach to renal failure in critically ill patients, the newly minted condition known as acute kidney injury has created the following sources of confusion:
1. The diagnosis of AKI includes prerenal conditions (e.g., hypovolemia) where there is no “injury” in the kidneys.
2. Oliguria (i.e., urine output <0.5 mL/kg/hr) is required for the diagnosis of AKI, which neglects cases of nonoliguric acute renal failure (e.g., interstitialnephritis, myoglobinuric renal failure).
3. There is a lack of agreement about the minimum increase in serum creatinine required for the diagnosis of AKI.
FIGURE 34.1 Comparison of in-hospital mortality rates for corresponding RIFLE and AKIN criteria in 291 patients with AKI. There is no difference between the classification systems for predicting mortality. Data from Reference 5.
DIAGNOSTIC CONSIDERATIONS
Categories
The clinical disorders that promote AKI can be categorized according to the location of the insult; i.e., prerenal, intrarenal, or postrenal.
Prerenal Disorders
The insult in prerenal disorders is a decrease in renal blood flow. Prerenal disorders are responsible for 30–40% of cases of AKI (6), and most cases are the result of hypovolemia and low-output heart failure. Prerenal AKI typically responds to interventions that augment systemic blood flow (e.g., volume resuscitation), but the response can be lost when the low-flow state is severe (e.g., hypovolemic shock).
Renal Disorders
The intrarenal conditions that produce AKI are acute tubular necrosis (ATN) and acute interstitial nephritis (AIN).
ATN: ATN is responsible for over 50% of cases of AKI (6). This condition was originally considered to be the result of renal hypoperfusion, but there is now convincing evidence that the pathologic process is inflammatory (oxidative) injury in the epithelial cell lining of the renal tubules (7). The damaged cells are sloughed into the lumen of the renal tubules, where they create an obstruction (see Figure 34.2). The luminal obstruction creates a back pressure on the luminal side of the glomerulus, and this decreases the net filtration pressure across the glomerulus, which reduces the glomerular filtration rate (GFR). This process is called tubuloglomerular feedback (8).
ATN is not a primary renal disease, and is typically a manifestation of one of the following disorders: severe sepsis and septic shock, radiocontrast dye, nephrotoxic drugs (e.g., aminoglycoside), or rhabdomyolysis with myoglobinuric renal injury.
AIN: AIN is also the result of inflammatory injury, but the injury is located in the renal interstitium rather than the renal tubules. AIN is de-scribed later in the chapter.
Postrenal Obstruction
Obstruction distal to the renal parenchyma is responsible for only about 10% of cases of AKI (6). The obstruction can involve the most distal portion of the renal collecting ducts (papillary necrosis), the ureters (extraluminal obstruction from a retroperitoneal mass), or the urethra (strictures). Ureteral obstruction from stones does not cause AKI unless there is a solitary functional kidney.
Common Sources of AKI
Most cases of AKI are caused by one of the clinical disorders in Table 34.2. The most common causes of AKI are listed in the left column. Sepsis (i.e., infection plus systemic inflammation) is the most common offender, and accounts for up to 50% of cases of AKI (3,9). AKI has been reported in up to 40% of postoperative patients following major surgery, particularly cardiopulmonary bypass surgery (3). AKI has also been reported in 30% of major trauma victims (3), and rhabdomyolysis is responsible for 30% of these cases (3). Nephrotoxic drugs and radiocontrast dye are implicated in about 20% of cases of AKI (9). Increased abdominal pressure is emerging as a common, and often overlooked, source of AKI. This condition is described later in the chapter.
FIGURE 34.2 Photomicrograph of acute tubular necrosis (ATN) showing a proximal tubule (outlined by the dotted line) filled with exfoliated renal tubular cells.
Table 34.2 Common Causes of Acute Kidney Injury
Diagnostic Evaluation
The evaluation of AKI begins with a bedside ultrasound evaluation of the kidneys for evidence of postrenal obstruction. If there is no obstruction, the remaining evaluation is aimed at determining if the problem is a prerenal disorder (e.g., hypovolemia or a reduced cardiac output) or an intrinsic renal disorder (e.g., ATN or AIN). The measurements in Table 34.3 can help to distinguish prerenal from renal disorders, but only in patients with oliguria.
Spot Urine Sodium
In prerenal disorders, the renal hypoperfusion is accompanied by an increase in sodium reabsorption in the renal tubules and a subsequent decrease in urine sodium concentration. In contrast, renal “tubulo-pathies” like ATN are characterized by impaired sodium reabsorption and increased urinary sodium losses. Therefore, when a random urine sample (spot urine) is obtained in a patient with AKI, a urine sodium <20 mEq/L is used as evidence of a prerenal disorder, while a urine sodium >40 mEq/L is used as evidence of an intrinsic renal disorder (10).
EXCEPTIONS: A prerenal disorder can be associated with a high urine sodium (>40 mEq/L) if there is ongoing diuretic therapy, or the patient has chronic renal disease (where there is “obligatory” sodium loss in the urine).
Table 34.3 Urinary Measurements for the Evaluation of AKI
Fractional Excretion of Sodium
The fractional excretion of sodium (FENa) is considered a more accurate measure of renal tubular function than the spot urine sodium concentration. The FENa is equivalent to the fractional sodium clearance divided by the fractional creatinine clearance, as expressed by the following equation:
(34.1)
(U/P is the urine-to-plasma ratio for sodium and creatinine concentrations.) In euvolemic patients with normal renal function, the FENa is 1% (i.e., only 1% of the filtered sodium is excreted in the urine). In prerenal disorders like hypovolemia, the FENa is <1% (reflecting sodium conservation), and in intrinsic renal disorders like ATN, the FENa is typically >2% (reflecting an increase in urinary sodium excretion) (11).
EXCEPTIONS: Like the spot urine sodium, the FENa can be falsely elevated (>1%) by diuretic therapy and chronic renal insufficiency (11). In addition, the FENa can be falsely low (<1%) in patients with renal failure due to sepsis (12), radiocontrast dyes (13), and hemoglobinuria or myoglobinuria (14).
Fractional Excretion of Urea
The fractional excretion of urea (FEU) is conceptually similar to the FENa, and is equivalent to the fractional urea clearance divided by the fractional creatinine clearance, as expressed by the following equation:
(34.2)
(U/P is the urine-to-plasma ratio for urea and creatinine concentrations.) The FEU is low (<35%) in prerenal disorders like hypovolemia, and high (>50%) in renal disorders like ATN. However, the FEU is not influenced by diuretics (15), which is the major advantage of the FEU over FENa.
Uncertainty
Distinguishing between prerenal and intrarenal causes of AKI can be difficult, and often a fluid challenge is necessary to distinguish between these two conditions (see next section).
INITIAL MANAGEMENT
The early management of AKI should include the following: (a) volume infusion to promote renal blood flow, (b) discontinuing any nephrotoxic drugs, and (c) treating any conditions that predispose to AKI (e.g., sepsis).
Fluid Challenges
If a prerenal source of AKI has not been ruled out, prompt volume infusion is warranted. Delays in correcting renal hypoperfusion can lead to intrarenal damage, so prompt attention to volume resuscitation is mandatory. Fluid challenges can be given in 500 ml to 1,000 mL aliquots for crystalloid fluids and 300 mL to 500 mL aliquots for colloid fluids, infused over 30 minutes (16). The fluid challenges are continued until there is a response (i.e., an increase in urine output), or until you are concerned about volume overload. (Remember that only 20–25% of infused crystalloid fluids remain in the intravascular space, so 500 mL of infused crystalloid fluid will increase the plasma volume by only 100–125 mL. Therefore, fluid challenges with crystalloid fluids should not stop if a volume of 500 ml does not produce a favorable response.) Diuretics should never be used to increase urine output until the possibility of a prerenal condition has been eliminated.
Hydroxyethyl Starch:
Several studies have shown an association between hydroxyethyl starch solutions and AKI (see page 231). Therefore, it is wise to avoid starch solutions for fluid challenges in AKI.
Intrarenal Disorders
The following considerations are relevant in patients with AKI due to intrarenal disorders (i.e., ATN and AIN). Unfortunately, the only early option available for halting or reversing the course of AKI is to discontinue possible offending drugs.
Furosemide
Despite its popularity in AKI, intravenous furosemide does not improve renal function in AKI, and does not convert oliguric to non-oliguric renal failure (3,17). Furosemide can increase urine output during the recovery phase of AKI (18), and a trial of furosemide is reasonable during this period to relieve fluid accumulation.
Low-Dose Dopamine
Low-dose dopamine (2 ∝g/kg/min) can act as a renal vasodilator, but it does not improve renal function in patients with AKI (19,20). Furthe-rmore, low-dose dopamine can have deleterious effects on hemodynamics (decreased splanchnic blood flow) immune function (inhibition of T-cell lymphocyte function) and endocrine function (inhibition of thyroid-stimulating hormone release from the pituitary) (20). Because of the lack of benefit combined with the risk of harm, the use of low-dose dopamine in patients with AKI is considered bad medicine (borrowing the title from Reference 20).
Nephrotoxic Agents
As mentioned, discontinuing possible offending drugs is the most effective early measure for halting or reversing the course of AKI. A variety of drugs can be responsible for AKI, as indicated in Table 34.4.
SPECIFIC CONDITIONS
Contrast-Induced Renal Injury
Iodinated contrast agents can damage the kidneys in several ways, including direct renal tubular injury, renal vasoconstriction, and the generation of toxic oxygen metabolites (21). Using the AKIN criteria for the diagnosis of AKI, the incidence of AKI after contrast studies is 8–9% (22). The AKI usually appears within 72 hours after the contrast study. The incidence is greater in patients with multiorgan failure, chronic renal insufficiency, or during therapy with other nephrotoxic agents (23). Most cases resolve within two weeks, and few require renal replacement therapy (24).
Prevention
INTRAVENOUS HYDRATION: The most effective preventive measure for contrast-induced nephropathy in high-risk patients is intravenous hydration (if permitted). The recommended regimen is isotonic saline at 100–150 mL/hr started 3 to 12 hours before the procedure and continued for 6–24 hours after the procedure (23). For emergency procedures, at least 300–500 mL isotonic saline should be infused just prior to the procedure.
Table 34.4 Drugs Most Often Implicated in Acute Kidney Injury
N-ACETYLCYSTEINE: N-acetylcysteine (NAC) is a glutathione surrogate with antioxidant actions that has had mixed results as a protective agent for contrast-induced nephropathy (3). However, an analysis of 16 studies using high-dose NAC (exceeding 1,200 mg daily) showed a 50% risk-reduction for contrast-induced nephropathy (24). The high-dose NAC regimen is 1,200 mg orally twice daily for 48 hours, beginning the night before the contrast procedure. For emergency procedures, the first 1,200 mg dose should be given just prior to the procedure. Despite continuing debate, NAC is a popular preventive agent because of its low cost and safety.
Acute Interstitial Nephritis (AIN)
AIN is an inflammatory condition that involves the renal interstitium and presents as acute renal failure. However, oliguria is not always a feature of AIN (25), which means that AIN does not always qualify for the diagnosis of AKI. Most cases of AIN are the result of a hypersensitivity drug reaction, but infections (usually viral or atypical pathogens) can also be involved. The drugs most often implicated in AIN are listed in Table 34.4 (26). Antibiotics are the most common offenders, particularly the penicillins.
Drug-induced AIN is often (but not always) accompanied by signs of a hypersensitivity reaction; i.e., fever, rash, and eosinophilia. The onset of renal injury usually appears several weeks after the first exposure (26), but can appear within a few days after a second exposure. Sterile pyuria and eosinophiluria are common manifestations (26). A renal biopsy can secure the diagnosis, but these are rarely performed. AIN usually re-solves spontaneously after the offending agent is discontinued, but recovery can take months
Myoglobinuric Renal Failure
Acute renal failure develops in about one-third of patients with diffuse muscle injury (rhabdomyolysis) (27,28). The culprit is myoglobin, which is released by the injured muscle and is capable of damaging the renal tubular epithelial cells. The source of cell injury may be the iron moiety in heme (29), which is capable of oxidative cell injury via the production of hydroxyl radicals (see Figure 22.6 on page 437). This would explain why hemoglobin is also capable of producing renal tubular injury.
The diagnosis of AKI can be difficult in the setting of rhabdomyolysis because the injured muscle releases creatine, which is measured as creatinine, and falsely elevates the serum creatinine concentration (29).
Myoglobin in Urine
Myoglobin can be detected in urine with the orthotoluidine dipstick reaction (Hemastix), which is used to detect occult blood in urine. If the test is positive, the urine should be centrifuged (to separate erythrocytes) and the supernatant should be passed through a micropore filter (to remove hemoglobin). A persistently positive test after these measures is evidence of myoglobin in urine. An alternative approach is to inspect the urine sediment for red blood cells; i.e., a positive dipstick test for blood without red blood cells in the urine sediment can be used as evidence of myoglobinuria.
The presence of myoglobin in urine does not ensure the diagnosis of AKI, but the absence of myoglobin in urine can be used to exclude the diagnosis of myoglobinuric renal injury (28).
Management
Aggressive volume resuscitation to promote renal blood flow is the most effective measure for preventing or limiting the renal injury in rhabdomyolysis. Alkalinizing the urine can also help to limit the renal injury, but this is difficult to accomplish, and is often not necessary. The potassium and phosphate levels in plasma must be monitored closely in rhabdomyolysis because these electrolytes are released by injured skeletal muscle, and their concentrations in plasma can increase dramatically, especially when renal function is impaired. About 30% of patients who develop myoglobinuric renal injury will require dialysis (28).
Abdominal Compartment Syndrome
Abdominal compartment syndrome (ACS) is the condition where an increase in abdominal pressure leads to dysfunction in one or more vital organs (30,31). This organ dysfunction usually involves the bowel (splanchnic ischemia), the kidneys (AKI), and cardiovascular system (reduced cardiac output).
Definitions
The pertinent definitions related to ACS are shown in Table 34.5 (30). The intraabdominal pressure (IAP) is normally 5–7 mm Hg in the supine position (the IAP measurement is described later), and intraabdominal hypertension (IAH) is defined as a sustained increase in IAP to ≥12 mm Hg. ACS occurs when the IAP rises above 20 mm Hg and there is evidence of a newly-developed organ dysfunction.
Table 34.5 Definitions Related to Intraabdominal Pressure
Predisposing Conditions
ACS is traditionally associated with abdominal trauma, but several conditions can raise the IAP and predispose to ACS, including gastric distension, bowel obstruction, ileus, peritoneal hemorrhage, ascites, bowel wall edema, hepatomegaly, positive-pressure breathing, upright body position, and obesity (31). Several of these factors can co-exist in critically ill patients, which explains why IAH is discovered in as many as 60% of patients in medical and surgical ICUs (32).
LARGE VOLUME RESUSCITATION: One of the more common and unrecognized causes of IAH is large volume resuscitation, which can raise the IAP by promoting edema in the abdominal organs (particularly the bowel). In one report of ICU patients with a net positive fluid balance >5 liters over 24 hours, ICH was discovered in 85% of the patients, and ACS was diagnosed in 25% of the patients (33). This observation adds to the growing consensus that avoiding a positive fluid balance will reduce morbidity and mortality in ICU patients (see page 457).
Renal Dysfunction
An increase in IAP can affect virtually every organ (by decreasing venous return and subsequently decreasing cardiac output), but the kidneys are most frequently affected. The influence of IAP on renal function can be explained by the two variables described next.
ABDOMINAL PERFUSION PRESSURE: The driving pressure for renal blood flow is the difference between the mean arterial pressure (MAP) and the mean pressure in the renal veins. When the IAP exceeds the renal venous pressure, the driving pressure for renal blood flow is the difference between MAP and IAP. This pressure difference is called the abdominal perfusion pressure (APP):
(34.3)
In patients with IAH, the APP is equivalent to the renal perfusion pressure, and thus an increase in IAP will reduce renal blood flow by decreasing the APP. The APP needed to preserve renal blood flow is not known, but in studies of IAH and ACS, maintaining an APP >60 mm Hg is associated with improved survival (30).
FILTRATION GRADIENT: The filtration gradient (FG) is the pressure gradient across the glomerulus, and is equivalent to the difference between the glomerular filtration pressure (GFP) and the proximal tubular pressure (PTP) (30):
(34.4)
In patients with IAH, GFP is considered equivalent to MAP – IAP, and PTP is considered equivalent to IAP, so equation 34.4 can be rewritten as:
(34.5)
According to this relationship, an increase in IAP will have a greater impact on glomerular filtration (and urine flow) than an equivalent decrease in MAP. This might explain why oliguria is one of the first signs of IAH (30).
Measuring Intraabdominal Pressure
Patients with AKI and a predisposing condition for ACS (which includes most ICU patients) should have a measurement of IAP. The physical examination is insensitive for detecting an increase in IAP (34), so the IAP must be measured. The standard measure of IAP is the pressure in a decompressed urinary bladder (intravesicular method). Specialized bladder drainage catheters are available for measuring IAP (e.g., from Bard Medical, Covington, GA). The following conditions are required for each measurement (30): (a) the patient must be in the supine position, with the pressure transducer zeroed along the mid-axillary line, (b) a small volume (25 mL) of isotonic saline in injected into the bladder 30–60 seconds prior to each measurement, and (c) the IAP is measured only at the end of expiration, and only when there is no evidence of abdominal muscle contractions. The IAP is measured in mm Hg, not cm H2O (1 mm Hg = 1.36 cm H2O).
Management
General measures for reducing IAP include sedation (to reduce abdominal muscle contractions), avoiding elevation of the head more than 20º above the horizontal plane (35), and avoiding a positive fluid balance. Specific measures are dictated by the source of the elevated IAP, and can include decompression of the stomach, small bowel, or colon, percutaneous drainage of peritoneal fluid, or surgery (e.g., for abdominal injuries or bowel obstruction). As mentioned earlier, efforts to maintain an APP >60 mm Hg (with vasopressors to increase MAP, if necessary) is associated with improved outcomes in ACS.
Surgical decompression is recommended for patients with ACS when the IAP cannot be reduced by conventional measures (35). However, this procedure has considerable risks (e.g., the abdomen is often left open for continued drainage), and these risks must be weighed against the risks of not performing the procedure.
RENAL REPLACEMENT THERAPY
About 70% of patients with acute renal failure will require some form of renal replacement therapy (RRT). The usual indications for RRT in acute renal failure include (a) volume overload, (b) life-threatening hyperkalemia or metabolic acidosis that is refractory to conventional measures, and (c) removal of toxins (e.g., ethylene glycol). Otherwise, the optimal timing for RRT in acute renal failure is unclear (36).
There is a growing body of RRT techniques, which includes not only hemodialysis and hemofiltration, but also hemodiafiltration, high flux dialysis, and plasmafiltration. The descriptions that follow are limited to hemodialysis and hemofiltration. The mechanisms of fluid and solute removal by each of these techniques is shown in Figure 34.3.
Hemodialysis
Hemodialysis removes solutes by diffusion, which is driven by the concentration gradient of solutes across a semipermeable membrane. To maintain this concentration gradient, a technique called countercurrent exchange is used, where blood and dialysis fluid are driven in opposite directions across the dialysis membrane. A blood pump is used to move blood in one direction across the dialysis membrane at a rate of 200–300 mL/min. The dialysis fluid on the other side of the membrane moves about twice as fast, at a rate of 500 to 800 mL/min (37). Large-bore, double lumen catheters are required for acute hemodialysis, and these catheters are described in Chapter 1 (see Table 1.5 on page 13, and Figure 1.6 on page 14).
Advantages and Disadvantages
The principal benefit of hemodialysis is rapid clearance of small solutes. Only a few hours of hemodialysis is needed to clear life-threatening accumulations of potassium or organic acids, or to remove a day’s worth of accumulated nitrogenous waste. The disadvantages of hemodialysis include (a) limited removal of large molecules (e.g., inflammatory cytokines), and (b) the need to maintain a blood flow of 200–300 mL/min through the dialysis chamber. This latter requirement creates a risk of hypotension, which occurs in about one-third of hemodialysis treatments (37).
Hemofiltration
Hemofiltration removes solutes by convection, where a hydrostatic pressure gradient is used to move a solute-containing fluid across a semipermeable membrane. Since the bulk movement of fluid “drags” the solute across the membrane, this method of solute removal is also known as solvent drag (37).
Hemofiltration can remove large volumes of fluid (up to 3 liters per hour), but the rate of solute clearance is much slower than during hemodialysis. Therefore, hemofiltration must be performed continuously to provide effective solute clearance. Because solutes are cleared with water, the plasma concentration of these solutes (e.g., urea) does not decrease during hemofiltration unless a solute-free intravenous fluid is infused to replace some of the ultrafiltrate that is lost (this is often necessary because of the large volumes removed during hemofiltration).
Methods
Hemofiltration was originally performed by cannulating an artery (radial, brachial, or femoral) and a large vein (internal jugular or femoral). This method of continuous arteriovenous hemofiltration (CAVH) uses the mean arterial pressure as the filtration pressure, and does not require a pump in the circuit. However, it is not well suited for patients with labile blood pressures.
FIGURE 34.3 Mechanisms of solute clearance by hemodialysis and hemofiltration. The smaller particles represent small solutes (e.g., urea), which can be cleared by both techniques, while the larger particles represent larger molecules (e.g., inflammatory cyto-kines) that can be cleared by hemofiltration, but not by hemodialysis.
The more popular method at present is continuous venovenous hemofiltration (CVVH), where venous blood is removed and returned through large-bore, double-lumen catheters like the ones used for hemodialysis. This method does not require cannulation of an artery, but a pump in the circuit is required to create an effective filtration pressure.
Advantages and Disadvantages
There are two major advantages with hemofiltration. First, hemofiltration allows more gradual fluid removal than hemodialysis, and thus is less likely to produce hemodynamic compromise. Secondly, hemofiltration removes larger molecules than hemodialysis, which makes it the preferred method for removing toxins such as ethylene glycol. This feature also allows for the removal of inflammatory mediators, which can provide a therapeutic advantage in patients with systemic inflammation and multiorgan failure (38).
The major disadvantage of hemofiltration is the slow solute removal, which is not well suited when rapid removal of solutes is necessary (e.g., for life-threatening hyperkalemia or acidosis). A newer method of RRT known as hemodiafiltration (which combines the features of dialysis and hemofiltration) is more suitable than hemofiltration for patients who require rapid solute removal as well as volume removal.
A FINAL WORD
The Dirac Equation & Acute Kidney Injury
The author of the introductory quote, Paul Dirac, was a prominent (and eccentric) theoretical physicist who introduced the concept of antimatter (39). His quote refers to his equation (the Dirac equation) for describing the behavior of electrons, which was later shown to have some limitations. Like the Dirac equation, the concept of acute kidney injury has not achieved its intent to describe the spectrum of renal failure experienced by critically ill patients. The sources of confusion created by the concept of acute kidney injury are described on page 635.
The concept of acute kidney injury has one feature that is unlike the Dirac equation; i.e., the Dirac equation added considerably to the understanding of how electrons behave, while the concept of acute kidney injury adds little to the understanding of how or why renal failure occurs in critically ill patients.
REFERENCES
Clinical Practice Guidelines
1. Fliser D, Laville M, Covic A, et al. A European Renal Best Practice (ERBP) Position Statement on Kidney Disease Improving Global Outcomes (KDIGO) clinical practice guidelines on acute kidney injury. Nephrol Dial Transplant 2012; 27:4263–4272.
2. Brochard L, Abroug F, Brenner M, et al. An official ATS/ERS/ESICM/SCCM/ SRLF statement: Prevention and management of acute renal failure in the ICU patient. Am J Respir Crit Care Med 2010; 1126–1155.
Diagnostic Criteria
3. Dennen P, Douglas IS, Anderson R. Acute kidney injury in the intensive care unit: an update and primer for the intensivist. Crit Care Med 2010; 38:261–275.
4. Ympa YP, Sakr Y, Reinhart K, et al. Has mortality from acute renal failure decreased? A systematic review of the literature. Am J Med 2005; 118:827–832.
5. Chang C-H, Lin C-Y, Tian Y-C, et al. Acute kidney injury classification: comparison of AKIN and RIFLE criteria. Shock 2010; 33:247–252.
Diagnostic Evaluation
6. Abernathy VE, Lieberthal W. Acute renal failure in the critically ill patient. Crit Care Clin 2002; 18:203–222.
7. Wang Z, Holthoff JH, Seely KA, et al. Development of oxidative stress in the peritubular capillary microenvironment mediates sepsis-induced renal microcirculatory failure and acute kidney injury. Am J Pathol 2012; 180:505–516.
8. Blantz RC, Pelayo JC. A functional role for the tubuloglomerular feedback mechanism. Kidney Int 1984; 25:739–746.
9. Uchino S, Kellum JA, Bellomo R, et al. Acute renal failure in critically ill patients. A multinational, multicenter study. JAMA 2005; 294:813–818.
10. Subramanian S, Ziedalski TM. Oliguria, volume overload, Na+ balance, and diuretics. Crit Care Clin 2005; 21:291–303.
11. Steiner RW. Interpreting the fractional excretion of sodium. Am J Med 1984; 77:699–702.
12. Vaz AJ. Low fractional excretion of urine sodium in acute renal failure due to sepsis. Arch Intern Med 1983; 143:738–739.
13. Fang LST, Sirota RA, Ebert TH, Lichtenstein NS. Low fractional excretion of sodium with contrast media-induced acute renal failure Arch Intern Med 1980; 140:531–533.
14. Corwin HL, Schreiber MJ, Fang LST. Low fractional excretion of sodium. Occurrence with hemoglobinuric- and myoglobinuric-induced acute renal failure. Arch Intern Med 1984; 144:981–982.
15. Gottfried J, Wiesen J, Raina R, Nally JV Jr. Finding the cause of acute kidney injury: which index of fractional excretion is better? Clev Clin J Med 2012; 79:121–126.
Initial Management
16. Vincent J-L, Gerlach H. Fluid resuscitation in severe sepsis and septic shock: an evidence-based review. Crit Care Med 2004; 32(Suppl):S451–S454.
17. Venkataram R, Kellum JA. The role of diuretic agents in the management of acute renal failure. Contrib Nephrol 2001; 132:158–170.
18. van der Voort PH, Boerma EC, Koopmans M, et al. Furosemide does not improve renal recovery after hemofiltration for acute renal failure in critically ill patients. A double blind randomized controlled trial. Crit Care Med 2009; 37:533–538.
19. Kellum JA, Decker JM. Use of dopamine in acute renal failure: a meta-analysis. Crit Care Med 2001; 29:1526–1531.
20. Holmes CL, Walley KR. Bad medicine. Low-dose dopamine in the ICU. Chest 2003; 123:1266–1275.
Contrast-Induced Renal Injury
21. Pierson PB, Hansell P, Lias P. Pathophysiology of contrast medium-induced nephropathy. Kidney Int 2005; 68:14–22.
22. Ehrmann S, Badin J, Savath L, et al. Acute kidney injury in the critically ill: Is iodinated contrast medium really harmful? Crit Care Med 2013; 41:1017–1025.
23. McCullough PA, Soman S. Acute kidney injury with iodinated contrast. Crit Care Med 2008; 36(Suppl):S204–S211.
24. Triverdi H, daram S, Szabo A, et al. High-dose N-acetylcysteine for the prevention of contrast-induced nephropathy. Am J Med 2009; 122:874.e9–15.
Acute Interstitial Nephritis
25. Ten RM, Torres VE, Millner DS, et al. Acute interstitial nephritis. Mayo Clin Proc 1988; 3:921–930.
26. Bentley ML, Corwin HL, Dasta J. Drug-induced acute kidney injury in the critically ill adult: Recognition and prevention strategies. Crit Care Med 2010; 38(Suppl): S169–S174.
Myoglobinuric Renal Injury
27. Beetham R. Biochemical investigation of suspected rhabdomyolysis. Ann Clin Biochem 2000; 2000:37:581–587.
28. Sharp LS, Rozycki GS, Feliciano DV. Rhabdomyolysis and secondary renal failure in critically ill surgical patients. Am J Surg 2004; 188:801–806.
29. Visweswaran P, Guntupalli J. Rhabdomyolysis. Crit Care Clin 1999; 15:415–428.
Abdominal Compartment Syndrome
30. Malbrain ML, Cheatham ML, Kirkpatrick A, et al. Results from the International Conference of Experts on Intra-abdominal Hypertension and Abdominal Compartment Syndrome. I. Definitions. Intensive Care Med 2006; 32:1722–1723.
31. Al-Mufarrej F, Abell LM, Chawla LS. Understanding intra-abdominal hypertension: from bench to bedside. J Intensive Care Med 2012; 27:145–160.
32. Malbrain ML, Chiumello D, Pelosi P, et al. Prevalence of intra-abdominal hypertension in critically ill patients: A multicenter epidemiological study. Intensive Care Med 2004; 30:822–829.
33. Daugherty EL, Hongyan L, Taichman D, et al. Abdominal compartment syndrome is common in medical ICU patients receiving large-volume resuscitation. J Intensive Care Med 2007; 22:294–299.
34. Sugrue M, Bauman A, Jones F, et al. Clinical examination is an inaccurate predictor of intraabdominal pressure. World J Surg 2002; 26:1428–1431.
35. Cheatham ML, Malbrain ML, Kirkpatrick A, et al. Results from the International Conference of Experts on Intra-abdominal Hypertension and Abdominal Compartment Syndrome. II. Recommendations. Intensive Care Med 2007; 33:951–962.
Renal Replacement Therapy
36. Pannu N, Klarenbach S, Wiebe N, et al. Renal replacement therapy in patients with acute renal failure. A systematic review. JAMA 2008; 299:793–805.
37. O’Reilly P, Tolwani A. Renal replacement therapy III. IHD, CRRT, SLED. Crit Care Clin 2005; 21:367–378.
38. Morgera S, Haase M, Kuss T, et al. Pilot study on the effects of high cutoff hemofiltration on the need for norepinephrine in septic patients with acute renal failure. Crit Care Med 2006; 34:2099–2104.
A Final Word
39. Farmelo G. The Strangest Man. The Hidden Life of Paul Dirac, Mystic of the Atom. New York: Basic Books, 2009.
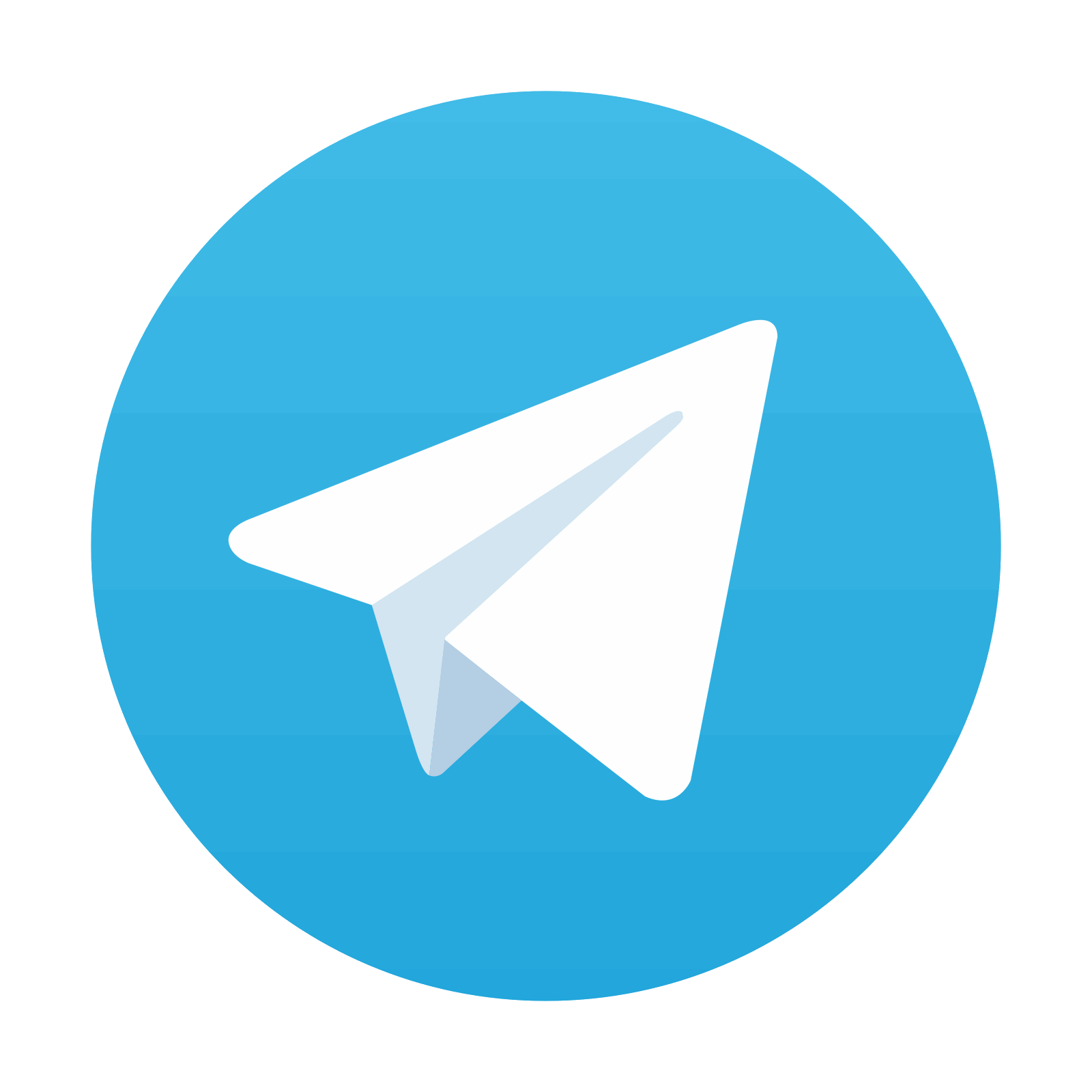
Stay updated, free articles. Join our Telegram channel

Full access? Get Clinical Tree
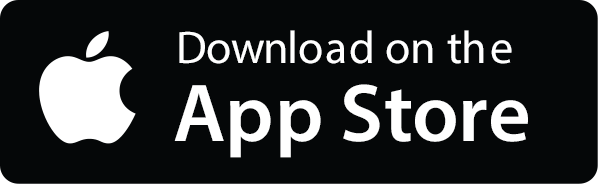
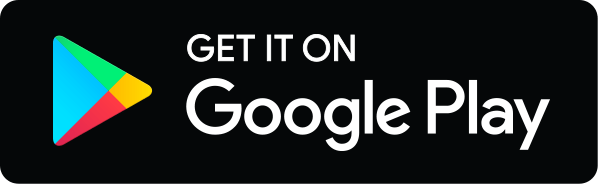