CHAPTER 16. Neurologic Trauma
Reneé Semonin Holleran and Katherine Logee
Competencies
Get Clinical Tree app for offline access
1. Perform a neurologic assessment.
2. Provide critical interventions for the patient with neurologic trauma to achieve maximal potential for recovery.
3. Use guidelines in the management of traumatic brain or spinal cord injury during transport.
Traumatic neurologic emergencies involve disorders of both the central and the peripheral nervous systems. In one way or another, most of these disorders ultimately affect the respiratory system; thus, airway management is crucial. However, depending on the patient’s condition, specific treatment may be instituted to lessen the impact of emergencies with which the transport team has to contend. The ultimate result of the disorders may be a progression to coma, often in association with increased intracranial pressure (ICP) or spinal injury. Therefore, the transport team must understand the causes of increased ICP and the neurologic syndromes discussed subsequently in this chapter.
TRAUMATIC BRAIN INJURY
Traumatic brain injury statistics are staggering. Traumatic brain injuries (TBI) are the leading cause of death related to trauma. Estimates are that both fatal and nonfatal traumatic brain injuries in the United States occur in 403 of 100,000 emergency department visits and in 85 of 100,000 that require hospital admission. 13 Two thirds of patients with head injuries are under the age of 30 years. During the past 18 years, United States involvement in the Middle East wars has shown the devastation of traumatic brain injury. The cost of care for people who survive neurologic injuries is in the millions of dollars. 1,2,5,9,13,17
Head injury is the major cause of death related to motor vehicle crashes. 1,2,9 The primary solution to the death and devastation caused by neurologic trauma is prevention.
The outcome for a patient who has sustained a traumatic brain injury (TBI) may be determined by the severity of the injury and the time elapsed before the patient receives adequate medical attention; thus, the need is for rapid evaluation, assessment, and transfer of the patient to an appropriate-level care facility by the transport team. The transport team must possess a basic knowledge of the principles of pathophysiology of traumatic brain injury to apply appropriate diagnostic and therapeutic methods and perform a thorough and ongoing systemic evaluation of the patient.
When trauma to the head occurs, the hair and scalp provide some dampening effect on impact. However, the brunt of the blow is delivered to the skull, which has enough elasticity to be flattened or indented when struck with a blunt object. The maximal depression occurs instantly and is followed within a few milliseconds by several oscillations. A severe blow to the skull actually causes a generalized deformation by flattening in the direction of the impact, with a corresponding widening of the diameter at right angles to the impact line. 16
The skull travels faster under impact than does the brain. Although the unbending skull often contuses the brain at the site of impact, severe brain injuries occur when the brain is hurled against the skull’s rough bony prominence, the crista galli, the major sphenoid wings, or the petrous bones. It is not uncommon for the frontal and temporal poles to be injured. The undersurface of the temporal poles and, less often, the occipital poles are contused or pulped as a result of the unbending skull. Similar damage can also be caused by the edges of the relatively unyielding falx and tentorium. So-called coup lesions develop in opposite areas of the brain on impact. 16
Damage may result from direct injury or from compression, tension, or shearing forces caused by the particular injury. In addition, secondary complications result from the traumatic brain injury. Ischemia and cerebral edema may ensue. An immediate increase in ICP seems to occur on impact; however, a secondary increase also occurs several minutes after the injury. The increase in ICP at the time of impact results from acceleration and deceleration of the head and deformation of the skull, the former being more significant than the latter. 13,14,16
During impact, cerebral spinal fluid may offer some protection to the brain. However, this protective layer is insufficient in the subarachnoid space around the frontal and temporal lobes, the most frequent sites of contusion. 13,16
Types of Traumatic Injuries: Pathologic and Clinical Considerations
Traumatic brain injury may exist in isolation; however, various combinations of injuries usually occur. Each component contributes in a different degree to the overall severity and outcome of the injury. 25,30
Skull Fracture
The skull is composed of three layers: an outer layer, a middle cancellous layer, and an inner layer that is half as thick as the outer layer and contains grooves that have large vessels. Whether a fracture actually occurs in the area of impact depends on the type of injury. The more concentrated and focused the impact tends to be, the greater the likelihood of a fracture.
Most skull fractures are linear. A linear skull fracture produces a line that usually extends toward the base of the skull. Impact can produce a single linear fracture or multiple fractures, referred to as linear stellate fractures, that radiate from the compressed area. Although linear fractures may look benign, they can cause serious complications. One such complication is infection. If the fracture line is open a few millimeters at the time of impact, debris such as hair, dirt, and glass may travel into the cranial vault. Linear fractures may also lead to epidural hematoma if the fracture line crosses a groove in the layer of the skull that houses the middle meningeal artery. Another complication occurs when the dura, which is strongly attached to the skull, tears at the fracture site.
Diastatic and Basilar Skull Fractures
Diastatic fracture involves a separation of bones at a suture line or a marked separation of bone fragments; both are usually visible on computed tomographic (CT) scans. Facial fracture may also play a role in head injuries. A blow to the lower jaw when the jaw is closed can cause the mandibular condyles to displace upward and backward against the base of the skull, leading to a concussion or a basilar skull fracture. Another type of facial fracture, which may or may not involve the cranium, is an orbital blowout fracture, which usually involves the floor of the orbit and is caused by blunt impact to the orbit and its contents.
Basilar skull fractures can occur when the mandibular condyles perforate into the base of the skull, but they most often result from extension of fractures of the calvaria. Basilar fractures often produce Battle’s sign (an oval-shaped bruise over the mastoid) or raccoon eyes (ecchymotic areas around the eyes).
Depressed Skull Fracture
The presence of depressed elements of a fracture may warrant specific diagnostic and therapeutic measures. If the depressed fracture is closed, the rationale for surgical correction is to evacuate any local mass if present, repair any dural lacerations to prevent cerebral herniation through the defect, and correct any cosmetic disfigurement caused by the depression. In general, if the depression on the tangential view of the skull is greater than the thickness of the skull, the dura is probably lacerated, and surgery is recommended. Depressions of a lesser degree, unless over the forehead, rarely necessitate surgical exploration.
A compound depressed skull fracture usually requires surgical debridement. If the injury has been caused by a blow to a static head, the patient’s level of consciousness is frequently well preserved, and no neurologic deficits may be seen. When a blow has been sustained to a moving head, consciousness is impaired.
Skull fractures can be the source of various complications, including intracranial infections, hematomas, air within the cranium, and meningeal and brain tissue damage. Traumatic pneumocephalus may occur if the frontal, ethmoid, or sphenoid sinuses or the mastoid processes are fractured. Air that has entered the skull locates in the epidural, subdural, subarachnoid, interventricular, or intercerebral space. Pneumocephalus seldom produces symptoms unless it is under tension and thus produces compression of the underlying brain tissue. The incidence rate of pneumocephalus and cerebral spinal fluid rhinorrhea with sella turcica fractures is small, but a high incidence rate of infection exists if this condition is present. Associated palsies of the oculi motor, trochlear, trigeminal, or abducens nerves may also be seen. 32.33. and 34.
In general, temporal bone fractures can cause pneumocephalus if dural tearing occurs in conjunction with injury to the eustachian tube, the middle ear, or the mastoid process. The patient may have sensory neurologic hearing loss, otorrhagia, or cerebral spinal fluid rhinorrhea in the presence of a temporal bone fracture.
Hemorrhage
Subdural Hematoma
Subdural hematoma is a collection of blood between the brain surface and the dura. It may occur as a result of a contusion or laceration of the brain with bleeding into the subdural space, tearing of the veins that bridge the subdural space, or an extension of an intercerebral hematoma through the brain surface into the subdural space. Subdural hematoma might be unassociated with skull fracture. 1,9,27,39
Subdural hematomas are classified as acute, subacute, or chronic, depending on the time elapsed between the injury and the appearance of signs and symptoms of neurologic dysfunction. As with other types of traumatic brain injury, the time course of development and the degree and rate of neurologic dysfunction depend on many factors. As a general rule, if dysfunction occurs within 24 hours, the hematoma is acute; if it occurs between 2 and 10 days, it is subacute; and if it occurs after 2 weeks, the hematoma is chronic. This particular classification is partially pathologic. The location of the hematoma and the amount of mass effect play important roles in determination of the timing of surgical intervention.
Elderly patients may have larger subdural hematomas with slowly developing symptoms because they have larger potential subdural spaces as a result of cerebral atrophy. In contrast, symptoms may be displayed rapidly and marked increases in intercranial pressure may develop in a younger patient with a small subdural space.
Subdural hematomas generally occur in children under the age of 2 years. Signs and symptoms include a bulging fontanel and a large head (because of separation of the sutures) and retinal hemorrhages (because of increased ICP). In the infant patient, a shock-like state may also develop because a relatively large blood volume loss may be caused by a subdural hematoma.
Acute subdural hematomas are usually associated with high morbidity and mortality rates, which reflects the usually severe nature of the associated injuries and the not-infrequent association of rapidly rising ICP resulting from the mass effect and development of cerebral edema. Two separate related pathophysiologic problems are cerebral contusion and edema and the presence of blood in the subdural space. The CT scan is valuable in determination of whether surgical intervention may be indicated. If the major problem contributing to poor neurologic status is the mass effect, then surgical intervention may be necessary. If the major problem is the cerebral injury, then corrective treatment should be directed toward the increased ICP.
Epidural Hematoma
An epidural hematoma is the collection of blood, usually arterial, between the skull and the dura. Epidural hematomas are classified as acute or subacute. An acute epidural hematoma that is arterial in origin generally produces symptoms within a few hours. Subacute epidural hematomas are venous in origin and take a long-er time to produce symptoms. These hematomas are associated with linear skull fractures in 90% of patients, but they may also occur as a result of blunt injuries in which no evidence of fracture is seen. 9 The classic symptoms displayed with epidural hematoma are transient loss of consciousness, recovery with a lucid interval during which neurologic status returns to normal, and the secondary onset of headache and a decreasing level of consciousness. As a result of the initial injury, the middle meningeal artery may tear and cause traumatic unconsciousness. Spasm and clotting then occur in the middle meningeal artery, and the bleeding stops. During the next several hours, the artery gradually bleeds, and a hematoma is formed, stripping the dura from the inside of the skull. Once a headache with a decreasing level of consciousness becomes obvious, the secondary rise in ICP has already occurred, and distortion of the brain with significant mass effect occurs. Because compensatory mechanisms of the inner cranial space have already been exhausted, the patient’s neurologic status rapidly deteriorates. The patient experiences a downhill course, usually with dilation of the ipsilateral pupil because of third-nerve compression by the herniating temporal structures, progressive unconsciousness with weakness or decerebration of either the contralateral extremities or the ipsilateral extremities, Cheyne-Stokes respirations, and, if no treatment is initiated, loss of pupillary reflexes, caloric responses, bradycardia, and death. Thus, identification of the epidural hematoma in the earliest possible stage, when a headache and drowsiness are the only symptoms, and transfer of the patient for immediate neurosurgical intervention are extremely important. 1,39 The classic history and clinical progression, however, is only seen in one third of patients with epidural hematomas. Another third are unconscious from the time of injury, and the final third are never unconscious. In children, bradycardia and early papilledema may be the only warning signs. 3,4
Cerebral Contusion
Cerebral hemorrhagic contusions frequently occur in patients, particularly adults, after traumatic brain injury. Of the patients who die of traumatic brain injury, 75% have contusions at autopsy. Hemorrhagic contusions are infrequently seen in children, but areas of localized decreased density on a CT scan may represent nonhemorrhagic contusions or possibly local ischemia. 1,9,14,16
Generally, no surgical intervention is recommended for cerebral contusions because brain matter cannot be removed in areas of the brain that control motor, sensory, or visual functioning. If, however, the contusion occurs over the frontal or temporal lobes, with significant edema and shift, surgical removal of contused portions of the brain is feasible. When a temporal lobe contusion is present and signs of herniation are seen, surgical excision of the temporal lobe may be beneficial. Generally, patients with contusion are treated with medical control of elevated ICP.
Intracerebral Hematoma
Movement of one section of brain tissue over or against another section causes tears in blood vessels, which leads to contusions or intracerebral hematomas. Most intra-cerebral hematomas are found in the frontal and temporal lobes, usually very deep, and are associated with necrosis and hemorrhage. The anatomic relationship between these areas and irregularities of the skull has already been discussed. Intracerebral hematomas are readily identified on the CT scan. The clinical picture may vary from no neurologic defect to deep coma.
Traumatic Brain Injury: Diffuse Axonal Injuries
Diffuse axonal injury (DAI) occurs when the delicate axons of the brain are stretched and damaged as a result of rapid movement of the brain. Mechanisms of injury associated with the acceleration and deceleration that occurs with high-speed motor vehicle crashes or ejection from a vehicle can cause this type of diffuse brain injury. Because the axons have been damaged, interference with neuron transmission is seen, and multiple neurologic deficits can range from headache and amnesia to severe deficits that include deep coma, posturing, and respiratory compromise. Severe DAI is usually associated with a high mortality rate. 10,16
Penetrating Injuries
Gunshot Wounds
When a person is shot at close range, evidence of smoke may be visible on the skin. When the muzzle of the gun is somewhat farther from the scalp but still close, evidence of powder burns may exist. A bullet striking the skull can cause great destruction of the underlying brain tissue.
Although some of the energy of impact may be dissipated by the shattering of bones and soft tissues, the impact on the brain after a bullet penetrates the skull is still great. The bullet’s ability to destroy tissues is directly related to its kinetic energy at the moment of impact. The degree of damage to the brain depends primarily on the muzzle velocity of the bullet and the distance between the gun and its target.
A bullet that passes through the head produces a larger defect on the inner table of the skull than that produced on the outer table. High-velocity bullets cause extensive injury to the brain and cranium. The entrance wound is usually smaller than the exit wound, but a great deal of variation in size may be seen. Multiple linear fractures that radiate from either the entrance or exit wound are common. Some fractures may be far away from the trajectory of the bullet, particularly in thin bones. The transport team should describe the wounds but not attempt to determine whether they are entrance or exit wounds.
Injuries to the major cerebral arteries, veins, or venous sinuses can occur in any of the bullet’s intra-cranial passages. Cerebral injuries cause an immedi-ate but transitory increase in ICP. The eventual ICP depends on the degree of intracranial bleeding, which may be profuse even in the absence of injury to major vessels. Secondary cerebral edema causes a delayed increase in ICP. Damage to the hemisphere causes loss of autoregulation, falling cerebral blood flow, an increase in cerebral blood volume and ICP, and eventually, brain death.
Intracranial hematomas are frequently associated with penetrating wounds to the brain. If the bullet passes close to or transverses the ventricle, an intraventricular hematoma may result.
Infection is seen often in injuries caused by shell fragments because these fragments are more likely than bullets to carry dirt, hair, and bone fragments into the brain. Infections develop most often from retained bone fragments, improper closure of the scalp and dura, and delay of definitive surgery beyond 48 hours.
Whenever the skull has been penetrated, a risk of intracranial infection exists. The injury should be managed to minimize that risk. All patients with penetrating injuries should receive tetanus prophylaxis.
Most stab wounds are caused by assaults with sharp instruments such as knives, scissors, and screwdrivers or when the patient (often a child) falls on a stick or sharp toy. The best method is to transport the patient with a stab wound with the object immobilized, secured, and left in place.
If the penetrating object has been removed, determination of exactly where penetration of the skull occurred may be difficult, particularly if entry occurred at the eyelid or sclera. When the patient arrives at the hospital, the area of injury is explored and debrided, as with an open injury.
Physical Assessment: Traumatic Brain Injury
Examination of a patient who is unconscious requires integration of information from several systems: mental status, pupils, other cranial nerves, motor system, and respiratory function (Table 16-1).
*May be normal with isolated medullary injury. | ||||||
Parameters | Cerebral Cortex | Diencephalon | Thalamus | Midbrain | Pons | Medulla |
---|---|---|---|---|---|---|
Mental status | Awake, alert, lethargic, obtunded | Light stupor | Deep stupor | Coma | Coma | Coma |
Motor response | Appropriate | Focal response to pain | General response to pain | Decerebrate posturing, decorticate posturing | Flaccid | Flaccid |
Pupil response | Normal size and reactivity | Small | Small | Midposition | Small | Small |
Oculocephalic, oculovestibular reflex | Not testable | Normal response | Normal response | Abnormal | Abnormal | Abnormal* |
Respiratory status | Variable | Variable | Cheyne- Stokes | Central neurogenic hyperventilation | Apneustic pattern | Apnea |
Level of Consciousness
The best indicator of changes in intracranial pressure, especially from a mass lesion, is a patient’s level of consciousness. 16,30Consciousness is a mental state in which the person is stimulated by the environment and can react appropriately to it. A useful way of describing the conscious state is to divide it into alert, lethargic, or obtunded stages (Box 16-1).
BOX 16-1
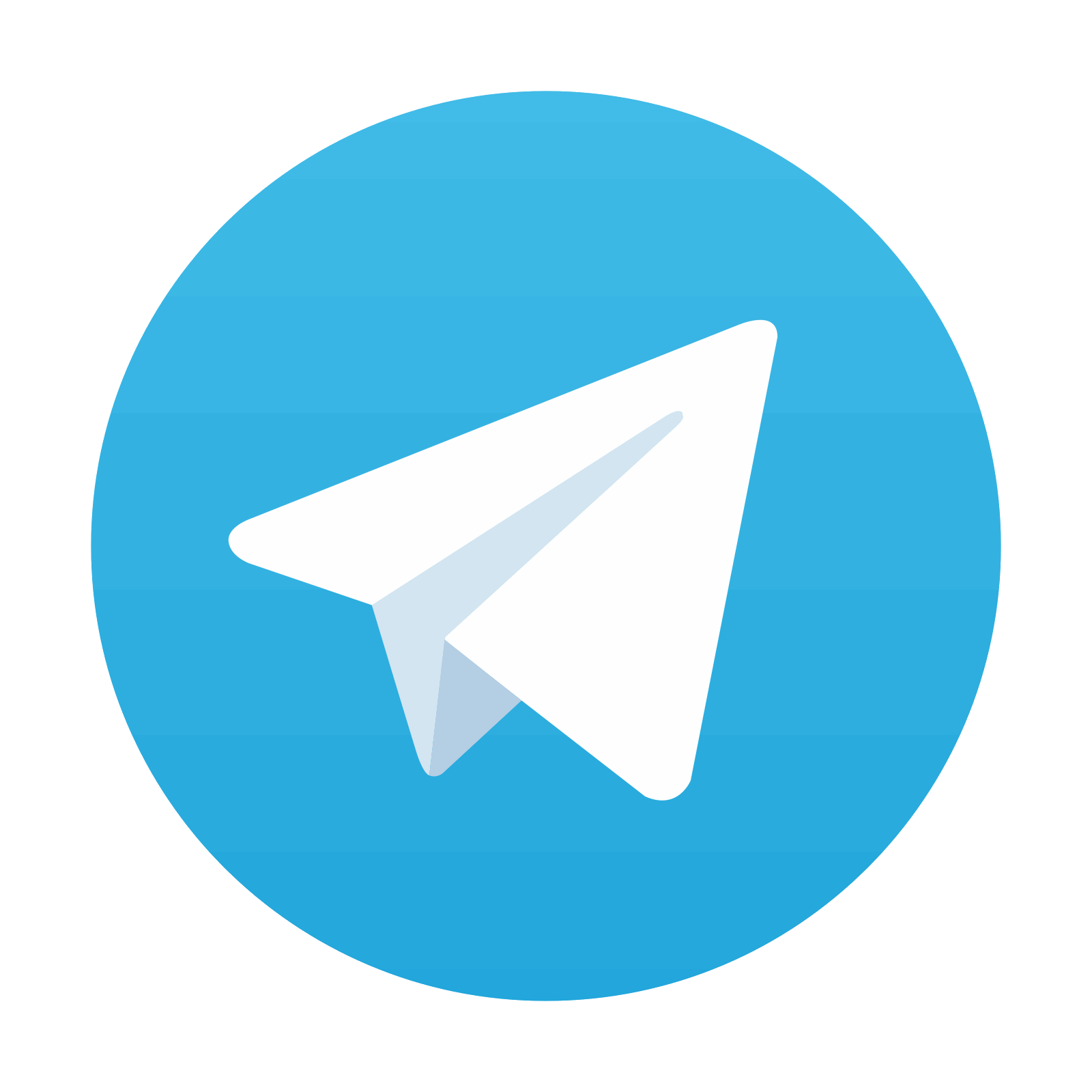
Stages in Progression from Consciousness to Unconsciousness
Conscious State
Alert: Patient responds readily but may have some confusion, speech disturbance, or motor deficit.
Lethargic: Patient appears drowsy or sleepy but can be aroused to respond to questioning.
Obtunded: Patient is extremely drowsy, is difficult to arouse, and rarely answers in complete sentences; examiner may have to repeatedly stimulate to gain patient’s attention.
Unconscious State
Stuporous: Patient does not verbalize appropriately or coherently; may moan and groan or utter monosyllables; responds to painful stimuli by moving extremities.
Comatose: Patient gives no evidence of awareness.
The alert patient readily responds to the examiner, although, depending on the state of the central nervous system (CNS) injury, some confusion, speech disturbance, and motor deficits may be seen. The lethargic patient appears to be drowsy or asleep but can be aroused easily and can respond reasonably appropriately to the examiner’s questions. However, if left alone, the patient slowly returns to an apparent sleep state or certainly lacks attentiveness. The obtunded patient is extremely drowsy, arouses with greater difficulty than a lethargic patient, rarely answers in complete sentences, and certainly does not volunteer information. In fact, during the active questioning period, the examiner may have to repeatedly stimulate the patient to gain attention.
Deterioration beyond the obtunded level results in the unconscious state. This state may be classified as either stupor or coma.
The stuporous patient does not verbalize appropriately or coherently. Two distinct levels of activity can characterize this state. The patient in a lightly stuporous state may moan and groan in response to stimulation or may utter an occasionally recognizable monosyllabic word, often a slang or curse word. The patient who is in a light stuporous condition responds to pain by moving all extremities, unless a primary motor system injury exists, and appears to crudely localize the site of the pain. However, a patient who is in a deeply stuporous state does not appear to localize and protect against pain. The patient who is in true coma may have decorticate posturing, decerebrate posturing, or flaccid motor response.
In examination of the pattern of motor response, the examiner must be aware of the possibility of primary motor system injury. For example, a left cortical lesion or a lesion in the left internal capsule may cause a contralateral hemiparesis that even in the awake patient may distort the motor response.
The comatose state roughly divides into three levels of reflex motor activity: decorticate posturing, decerebrate posturing, and flaccidity, to use clinically descriptive terms rather than more precise neurophysiologic descriptions. The patient in a decorticate state is unconscious and gives no evidence of awareness. Painful stimulation causes extensor rigidity in the lower extremities combined with a flexor posture of the upper extremities. Depending on the extent of the underlying damage to the motor system, this posturing may occur spontaneously or after painful stimulation and may be more prominent on one side than the other. Decerebrate posturing is exhibited by extensor rigidity in all four extremities. The patient who is flaccid has no motor response to painful stimulation.
For consciousness to be present, a stimulus must be presented to the CNS and must pass through the brain stem (with the exception of visual stimulation) to the diencephalon. From there, the stimulus must reach the cerebral cortex, where it is recorded. The patient must have sufficient cortical function so that the stimulus can excite associations through memory, which lets the patient acknowledge the presence of the stimulus and make use of that stimulus to relate appropriately to the external environment.
For example, when an intracranial mass lesion develops after head trauma and unconsciousness does not initially result, the patient may be expected, as the mass lesion increases, to progress systematically through the various levels and stages just described. The mass lesion may be a hematoma or a significant cerebral edema. A patient with a traumatic brain injury resulting in a primary upper brain stem lesion might be unconscious and may immediately evidence a comatose state without ever having had cortical or diencephalic deterioration. A person who survives a near drowning or delayed cardiopulmonary resuscitation may have severe bilateral cortical injury and may not progress significantly. A person with a spontaneous hemorrhage in the brain stem, particularly in the region of the pons or midbrain, is expected to become suddenly comatose with no evidence of an orderly progression through the stages noted previously.
Examination of the Pupils
The pupils are innervated by both the parasympathetic (third-nerve) and the sympathetic systems, with the former causing constriction and the latter causing dilation. The size of the pupil depends on the degree to which each system influences the pupil at the time of examination. The normal pupil constricts promptly to light. Examination of the pupils consists of assessment of the relative size of the two pupils and their reactivity to light. Injury to the parasympathetic system results in pupillary dilation.
Injury to the parasympathetic system may occur within the midbrain at the origin of the parasympathetic contribution to the third nerve, or it may occur outside the brain stem where the third nerve exits and proceeds forward beneath the brain into the region of the cavernous sinus. The sympathetic innervation begins in the posterior hypothalamus, descends the length of the brain stem and cervical cord, and exits in the lower cervical upper thoracic area, where it proceeds up the neck in the cervical sympathetic chain to the base of the skull and then out to the orbit where innervation occurs.
Injury to the sympathetic system results in pupillary constriction because of the actions of the unopposed third nerve. The sympathetic system can be injured within the CNS anywhere along its pathway and during its course through the chest and neck. Because of the relatively small size of the structures involved, lesions within the brain or brain stem are unlikely to affect either the parasympathetic or the sympathetic systems unilaterally. Therefore, we can assume that if bilateral pupil abnormalities are seen, a lesion in the brain or brain stem has affected the nerve supply to the pupils. For example, bilaterally small pupils may very well be caused by a lesion within the brain stem that affects both descending sympathetic tracts. On the other hand, a unilaterally affected pupil can be expected to be caused by a lesion of the tracts outside the brain or brain stem (extraaxial). A unilaterally dilated pupil may be caused by compression of the third nerve by a herniating temporal lobe after it has exited the midbrain and as it crosses the floor of the skull. A unilateral small pupil that results from sympathetic denervation reacts more sluggishly to light. Bilaterally dilated and fixed pupils are generally caused by global hypoxia or by bilateral temporal lobe herniation from central cerebral edema with bilateral third-nerve compression. Bilaterally constricted pupils may be caused by central herniation of the posterior hypothalamus at the site of origin of the sympathetic fibers through the tentorial notch or by bilateral involvement within the brain stem, such as from a pontine hemorrhage. Midbrain lesions that affect the parasympathetic bilaterally yield pupils that are in midposition and are nonreactive to light. Examination of other cranial nerves is helpful because they can reveal the competency of brain stem function including the III, IV, and VI nerves.
Brain Stem and Cranial Nerves
The integrity of the brain stem can be evaluated with examination of certain cranial nerves, especially those related to conjugate gaze. In the patient who is awake, conjugate gaze is controlled by visual input through the complex system that coordinates the function of the extraocular muscles by way of cranial nerves III, IV, and VI. In the patient who is unconscious, however, visual input gives way to vestibular input to control conjugate gaze. This is best evaluated with examination of the oculocephalic or oculovestibular reflexes. 16,37
< div class='tao-gold-member'>
Only gold members can continue reading. Log In or Register a > to continue
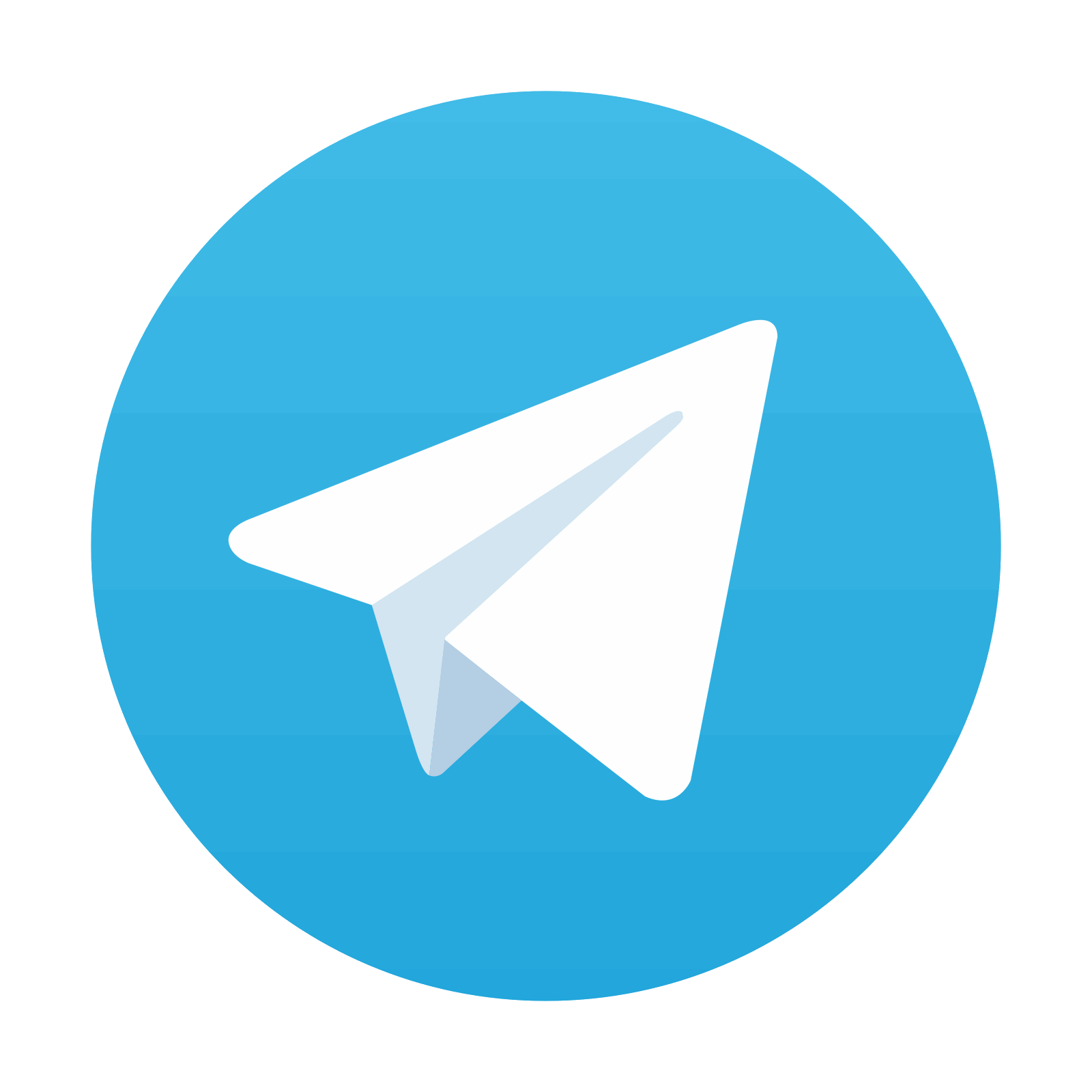
Stay updated, free articles. Join our Telegram channel

Full access? Get Clinical Tree
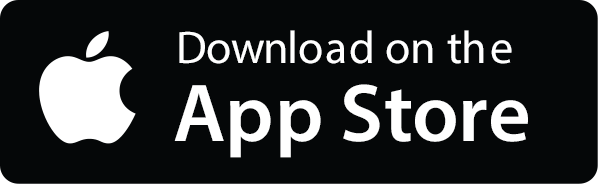
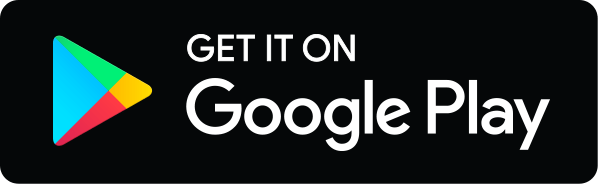
