Neuromuscular weakness from spinal cord injury, peripheral nerve disease processes such as Guillain-Barré syndrome, the neuromuscular junction like myasthenia gravis, or myopathy can be assessed with a negative inspiratory force (NIF) and vital capacity (VC).
A normal NIF should be less than than negative twenty. A normal VC is approximately 65 mL/kg, as shown in Figure 2.5 At a VC of 30 mL/kg, clearance of secretions is compromised. Below 10 and 15 mL/kg, hypoventilation and hypercapnea occurs. As always, rise in Pco2 or a drop in Po2 are late indicators of respiratory failure. To avoid this, intubation and mechanical ventilation typically should occur when the VC rapidly drops which indicates deterioration or if the VC approaches 20 mL/kg.
Figure 2. Respiratory Pathophysiology vs. Ventilatory Support.
During intubation, for patients in whom increased intracranial pressure (ICP) is a concern, intravenous lidocaine dosed at 1 mg/kg has been shown to dramatically blunt the ICP response.6 It is more effective in blunting the ICP elevations when administered intravenously rather than topically during direct laryngoscopy.
Basic Premises of Intracranial Pressure
To understand the impact of mechanical ventilation on the brain, it is important to be familiar with the basic premises of ICP. The Monro-Kellie hypothesis maintains that within the skull, a semi-closed box, are the brain, fluid (ie, interstitial fluid, cerebrospinal fluid), and blood. If a lesion takes up space in the brain, cerebrospinal fluid and cerebral blood volume can decrease to accommodate the increased volume. From the clinical standpoint, efforts to decrease intracranial pressure include maneuvers to decrease intracranial volume. Acutely, these include hyperventilation, which causes vasoconstriction and thereby blood volume; sedation, which lowers cerebral metabolism and requirements for cerebral blood flow; and osmotic therapy with mannitol or hypertonic saline, which draws interstitial fluid from the brain. The extent to which these maneuvers decrease the pressure depends upon the intracranial compliance.
Cerebral atrophy occurs as people age. Compliance (volume related to pressure), is high in these patients as a large change in volume may not greatly affect the ICP. In children and young adults, a small volume will increase the ICP. Following traumatic brain injury (TBI), the compliance curve steepens. In addition, studies have shown that sustained ICPs greater than 20 mm Hg decrease the chance that the patient will return to a good quality of life. For this reason, following (TBI), the goal is typically to maintain an ICP less than 20 mm Hg.2
Another key element to understanding the management of intracranial pressure is the concept of cerebral autoregulation. When blood pressure rises, cerebral blood flow is maintained by vasoconstriction. As blood pressure drops, cerebral vasodilatation results. Autoregulation can no longer maintain blood flow at the extremes of blood pressure. Ischemia occurs during severe hypotension. During accelerated or severe hypertension, hyperemia results with a breakdown of blood brain barrier and cerebral edema and hemorrhage ensue.7 For hypertensive individuals, the entire autoregulation curve is shifted to the right.
In areas of brain injury, the autoregulation is defective. For example, in patients with a contusion following TBI, in the area of contused brain, the relationship of pressure to blood flow is directly proportional. This also is true in the ischemic area of a stroke. When the blood pressure rises, passive vasodilation results causing more hyperemia, increased intracranial pressure and disruption of the blood brain barrier, which in turn creates more edema and hemorrhage. Conversely, as the blood pressure decreases, vessels collapse which results in cerebral ischemia.
Another basic premise in dealing with brain injury is that the difference of mean arterial pressure (MAP) and ICP is cerebral perfusion pressure (CPP). A normal CPP is between 70 and 100 mm Hg. An adequate CCP is between 50 and 60 mm Hg. TBI studies show that a CPP less than 60 mm Hg is predictive of poor outcome. CPP >60 mm Hg is adequate. Higher CPP goals have been associated with increases in acute lung injury and acute respiratory distress syndrome.8 These thresholds are different in children, but no guidelines have been established in this population.
When treating a patient with a high MAP in whom may also have intracranial hypertension, especially if the patient does not have an intracranial monitor, it is important not to overtreat the MAP. As an example, a healthy patient has an ICP of 5 mm Hg and a MAP of approximately 75 mm Hg. The CPP is maintained at 70 mm Hg. However, in a patient with cerebral contusion, stroke, or a cerebral tumor, MAP may be elevated as a response to an elevated ICP as an autoregulatory attempt to maintain an adequate CPP. In this instance, pharmacological attempts to lower blood pressure are ill-advised, as this may result in a lower CPP, poor perfusion and ischemia. In this instance, the strategy should be to treat a potential elevation of ICP. A trial of hypertonic saline can be both therapeutic and diagnostic. If blood pressure drops as a result of an infusion of 250 mL of 3% saline or 30 mL of 23.4%, which would normally be expected to elevate blood pressure, it is typically a result of intracranial pressure decrease with subsequent autoregulatory decrease in MAP when CPP is improved by dropping ICP.
Oxygenation
Following acute head injury, hypotension defined as a systolic blood pressure less than 90 mm Hg and hypoxemia with PaO2 less than 60 mm Hg significantly worsen neurological outcome.9,10 In neurologically injured patients, ventilator management strategies often include maintaining a partial pressure of arterial oxygen (PaO2) 90 mm Hg or higher. In patients with high ICP and steepened cerebral compliance curves, episodes of desaturation and hypoxemia are not well-tolerated. Even incrementally small changes in cerebral blood flow may increase the blood volume and are associated with elevated spikes in intracranial pressure.
Hypercarbia and Hypocarbia
Hyperventilation to a PaCO2 of 25 to 30 mm Hg can cause significant vasoconstriction and a reduction in cerebral blood flow. As a reminder, cerebral autoregulation occurs in relatively intact brain, not in the contusional or ischemic tissue. Vasoconstriction associated with hyperventilation may induce ischemia in areas. This premise has been studied in multiple trials.11-14 In one trial, patients with severe head injury were randomized to either empiric hyperventilation of PaCO2 of 25 mm Hg or ventilated to a PCO2 of 35 mm Hg.15 Patients kept normocapnic had a better outcome as measured by GCS outcome scores at 3 and 6 months although these differences equalized after one year. Secondary cerebral ischemia may occur with hyperventilation and should be avoided.
Guidelines recommend against empiric hyperventilation. When hyperventilation is necessary to lower intracranial pressure, typically PaCO2 <30 mm Hg are avoided.
PaCO2 rapidly equilibrates across the blood–brain barrier. During hyperventilation the concentration of carbonic acid decreases and increases the brain extracellular fluid pH. Bicarbonate does not readily cross the blood–brain barrier, however within hours the bicarbonate level begins to decrease in the extracellular fluid such that within approximately 30 hours, the brain pH is normal. For this reason, hyperventilation should be maintained temporarily while other maneuvers are utilized to lower ICP. In one experimental model, rabbits were hyperventilated to 25 mm Hg from 38 mm Hg, the vessels vasoconstricted by 13%. Every 4 hours, vasoconstriction decreased by 3% until after 20 hours, vessels more dilated than they were at baseline. After 52 hours, with the PaCO2 as low at 25 mmHg, vessel diameter was 105% of baseline; however, at this late time, if the PaCO2 was restored to 38 mm Hg, vessel dilated to 122% of baseline.16 PaCO2 should be normalized quickly to avoid this response and is best used as a rescue therapy for only minutes while other methods to lower ICP are implemented.
Temperature
While normalizing PaCO2, it is essential to remember that muscle activity and warmer temperatures are associated with an increase in metabolism and higher CO2 production. Each increase of 1°C increases the cerebral metabolic rate by 7%. If the cerebral metabolic rate goes up, so follows cerebral blood flow, blood volume, ICP, and blood pressure. In one exercise study, metabolic rate increased 23%.17 Consequently, in patients with fever or who are hypothermic, close attention to minute ventilation is essential ventilator management in patients whom ICP is being managed. For various reasons such as cardiac arrest, hypothermia is utilized as a neuroprotectant and PaCO2 must be followed closely during the cooling and warming periods.
The same principle regarding CO2 applies to shivering. Shivering increases the metabolic rate of the muscles, and so the CO2 production must be increased. Sometimes shivering by itself will increase the ICP and CO2 as well. Shivering has been managed by warming hands and feet, dilaudid use, or in extreme cases, with neuromuscular blockade. Obviously the source of hyperthermia should be evaluated.
The Effects of Positive End-Expiratory Pressure on the Brain
The effect of positive end-expiratory pressure (PEEP) is relevant to ICP when it increases the right atrial pressure and superior vena cava pressure subsequently decreasing venous outflow of the brain.18,19 When the central venous pressure (CVP) is higher than the ICP, then CVP rather than ICP becomes the determinant pressure for CPP. PEEP also affects CPP when the PEEP decreases venous return to the heart and decreases the MAP which subsequently decreases CPP.
For PEEP to be relevant, the pressures must be transmitted across the alveoli to affect the intrapleural pressure. This will be seen in patients with compliant lungs but poor chest wall compliance. Studies evaluating this suggest that PEEP will make a difference provided that it is upward of 20 cm H2O and only when the chest wall is extremely stiff.
One study looked at the effects of PEEP on CVP in dogs who were prone and those whose heads were elevated.20 They found that PEEP increases CVP in prone dogs but had only a small effect if the dogs’ heads were elevated and with the chest wall was strapped tightly. It was suggested that PEEP’s effect was most likely attenuated by collapsible venous channels. If one were to extrapolate this information, in patients who upright, PEEP should not influence ICP.
The Impact of Ventilation Modes
The different modes of ventilation can also affect the brain. For example, high-frequency oscillation ventilation (HFOV) decouples oxygenation and ventilation. When using HFOV, oxygenation is determined by the mean airway pressure and the fraction of inspired O2 (FIO2). Lower oscillatory frequency and higher pressure amplitudes results in a lower PaCO2.
The effect of HFOV on ICP has been the subject of a couple of studies. Mean airway pressure greater 30 mm cm H2O appears to increase ICP.21,22 High airway pressures decrease the MAP, which will decrease the CPP. Also, unless there is careful attention to the PaCO2, typically a change to HFOV as the mode of ventilation is associated with an increase in PaCO2 and ICP. Despite this, HFOV has been used successfully in patients with TBI. In one study, the ratio of PaO2 to FIO2 improved with the switch to HFOV in all patients.23 Most of the patients also experienced an increase in PaCO2 but ICP was successfully managed.
Another ventilation mode, airway pressure-release ventilation (APRV) affects ICP dependents on the elevation of airway pressure due to its affect on CPP. Overall, pressure-controlled modes of ventilation typically make ICP management challenging since the lack of set minute ventilation varies the PaCO2 unpredictably.
With APRV, the oxygenation is maintained by FIO2 and how high the pressure is set. Ventilation will depend on the timing, duration of pressure release, and lung compliance. Interestingly APRV has been reported to increase venous return, which may improve ICP and CPP. This mode also increases the cardiac index, so giving patients APRV may facilitate cerebral oxygenation.
Brain Tissue Oxygenation
Various studies suggest that brain tissue oxygenation (PBt o2) should be monitored to optimize neurological outcome. Although this mode of cerebral monitoring has not been widely adopted and does not have guidelines, some suggest that a PBt o2 of 15 or 20 mm Hg should be maintained.24
This can be achieved utilizing two concept of cerebral blood flow determined directly by blood pressure, radius of the vessel and inversely to viscosity. Also oxygen delivery can be manipulated by increases in cardiac output, hemoglobin and oxygen saturation. It is not clear that actual goal-directed manipulation of these parameters improve neurological outcome. Studies to evaluate this are on-going.
In practical management, the clinician can increase the brain oxygenation by increasing CPP, raising the PaCO2 to increase the radius of the vessel or avoiding polycythemia. In animals, a hematocrit of 30 ±3, optimizes delivery of O2 while balancing the viscosity of the blood. Other strategies include the use of an inotrope to increase cardiac output. Although increases in FIO2 increase the measured PBto2, it is not clear that this is a strategy that should be used long-term due to its known pulmonary toxicity. Other caveats included the concern during manipulated elevation in CPP for the potential to worsen hyperemia with worsened edema in contusional or ischemic brain and the increase in PaCO2 to increase ICP.
Neurogenic Pulmonary Edema
Neurogenic pulmonary edema (NPE) is another neurologic cause of respiratory failure. Classic NPE occurs within minutes to hours after a massive head injury. The delayed phase or type looks very much like acute respiratory distress syndrome. It is a slow progression, occurring within 12 to 72 hours after a massive head injury. In a delayed form, it is more difficult to recognize since other causes of pulmonary infiltrates and worsening oxygenation are often seen in this population. NPE is most commonly associated with head trauma but is also seen with subarachnoid hemorrhage, intracranial hemorrhage, seizures, and ischemic stroke.25
Clinical features of NPE include dyspnea if the patient is awake and hemoptysis. Signs include tachypnea, tachycardic, and rales. Laboratory findings reveal hypoxemia and mild leukocytosis. Chest radiographs show a bilateral alveolar filling process.
The mechanism of pulmonary edema after head trauma entails both hydrostatic and permeability changes. When acute brain injury occurs, the hypothalamus is disturbed as depicted in Figure 3. There is a massive α-adrenergic discharge that triggers sympathetic vasoconstriction. The aortic systemic blood pressure increases and the left ventricular compliance decreases. There is increased left atrial pressure, which will increase the pulmonary capillary pressure. Also, the sympathetic vasoconstriction shifts blood from systemic to pulmonary, increasing the pulmonary blood volume and pulmonary capillary pressures. With the α-adrenergic discharge, the pulmonary vasoconstriction increases the pulmonary venous pressures which results in pulmonary edema.26 There are animal studies showing that sympathetic discharge cause constriction of sphincters found in the post-capillary venules.27
Figure 3. Acute Brain Injury.
With permission from Theodore J, Robin ED. Speculation on neurogenic pulmonary edema (NPE). Am Rev Resp Dis. 1976;113:405-411.
Therapy for NPE is supportive. These patients are managed with positive-pressure ventilation and a high level of supplemental O2. Sometimes diuretics can be helpful. Although counter-intuitive, inotropes has been used successfully in some of these patients. Some suggest using α-adrenergic blockers although the entity is sufficiently uncommon to preclude randomized controlled trials in its management. Typically if the patient is supported with mechanical ventilation, within a few hours the chest radiograph, oxygenation including the ratio of PaO2 to FIO2 are normalized.
In summary, when approaching a patient with neurological injury, one must consider the neurologic causes of respiratory failure: mental status, drive to breathe, neuromuscular strength and ability to swallow (ie, dysphagia). Neurologically injured patients should be well oxygenated. If they must be hyperventilated for ICP management, it should not be by too much or for too long. Be aware that manipulation of the ventilator can impact cerebral perfusion and oxygenation. Finally, neurogenic pulmonary edema should be recognized as it can arise quickly, though transiently. It can be associated with head injury, subarachnoid hemorrhage, and seizures.
References
- Teasdale G, Jennett B. Assessment of coma and impaired consciousness: a practical scale. Lancet. 1974;2:81-84.
- Brain Trauma Foundation, American Association of Neurological Surgeons, Joint Section on Neurotrauma and Critical Care. Guidelines for the management of severe traumatic brain injury. J Neurotrauma. 2000;17:457-554.
- Plum F, Posner JB. The Diagnosis of Stupor and Coma. 3rd ed. Philadelphia, PA: FA Davis Company; 1980.
- DeVivo MJ, Krause MJ, Lammertse DP. Recent trends in mortality and causes of death among persons with spinal cord injury. Arch Phys Med Rehabil. 1999;80:1411-1419.
- Ropper AH, Gress DR, Diringer MN, et al. Neurological and Neurosurgical Intensive Care. 4th ed. Philadelphia, PA: Lippincott Williams & Wilkins; 2004.
- Hamill JF, Bedford RF, Weaver DC, et al. Lidocaine before endotracheal intubation: intravenous or laryngotracheal? Anesthesiology. 1981;55:578–581.
- Lang EW, Lagopoulos J, Griffith J, et al. Cerebral vasomotor reactivity testing in head injury: the link between pressure and flow. J Neurol Neurosurg Psychiatry. 2003;74:1053–1059.
- Robertson CS, Valadka AB, Hannay HJ, et al. Prevention of secondary ischemic insults after severe head injury. Crit Care Med. 1999;27:2086-2095.
- Chestnut RM, Marshall LF, Kaluber MR, et al. The role of secondary brain injury in determining outcome from severe head injury. J Trauma. 1993;34:216-222.
- Marmarou A, Anderson RL, Ward JD, et al. Impact of ICP instability and hypotension on outcome in apateints with severe head trauma. J Neurosurg. 1991;75:S159-S166.
- Coles JP, Minhas PS, Fryer TD, et al. Effect of hyperventilation on cerebral blood flow in traumatic head injury: clinical relevance and monitoring correlates. Crit Care Med. 2002;30:1950–1959.
- Diringer MN, Videen TO, Yundt K, et al. Regional cerebrovascular and metabolic effects of hyperventilation after severe traumatic brain injury. J Neurosurg. 2002;96:103–108.
- Imberti R, Bellinzona G, Langer M. Cerebral tissue PO2 and SjvO2 changes during moderate hyperventilation in patients with severe traumatic brain injury. J Neurosurg. 2002;96:97–102.
- Raichie ME, Plum F. Hyperventilation and cerebral blood flow. Stroke. 1972;3:566–575.
- Muizelaar JP, Marmarou A, Ward JD, et al. Adverse effects of prolonged hyperventilation in patients with severe head injury: a randomized clinical trial. J Neurosurg. 1991;75:731–739.
- Muizelaar JP, van der Poel HG, Li ZC, et al. Pial arteriolar vessel diameter and CO2 reactivity during prolonged hyperventilation in the rabbit. J Neurosurg. 1988;69:923–927.
- Nunneley SA, Martin CC, Slauson JW, et al. Changes in regional cerebral metabolism during systemic hyperthermia in humans. J Appl Physiol. 2002;92:846–851.
- Huseby JS, Pavlin EG, Butler J. Effect of positive end-expiratory pressure on intracranial pressure in dogs. J Appl Physiol. 1978;44:25–27.
- Luce JM, Huseby JS, Kirk W, et al. Mechanism by which positive end-expiratory pressure increases cerebrospinal fluid pressure in dogs. J Appl Physiol. 1982;52:231–235.
- Toung TJK, Aizawa H, Traystman RJ. Effects of positive end-expiratory pressure ventilation on cerebral venous pressure with head elevation in dogs. J Appl Physiol. 2000;88:655–661.
- O’Rourke J, Sheeran P, Heaney M, et al. Effects of sequential changes from conventional ventilation to high-frequency oscillatory ventilation at increasing mean airway pressures in an ovine model of combined lung and head injury. Eur J Anaesthesiol. 2007;24:454–463.
- David M, Markstaller K, Depta AL, et al. Initiation of high-frequency oscillatory ventilation and its effects upon cerebral circulation in pigs: an experimental study. Br J Anaesth. 2006;97:525–532.
- David M, Karmrodt J, Weiler N, et al. High-frequency oscillatory ventilation in adults with traumatic brain injury and acute respiratory distress syndrome. Acta Anaesthesiol Scand. 2005;49:209–214.
- Nortje J, Gupta AK. The role of tissue oxygen monitoring in patients with acute brain injury. Br J Anaesth. 2006;97:95-106.
- Simon RP. Neurogenic pulmonary edema. Neurol Clin. 1993;11:309-323.
- Theodore J, Robin ED. Speculation on neurogenic pulmonary edema (NPE). Am Rev Resp Dis. 1976;113:405-411.
- Schraufnagel DE, Patel KR. Sphincters in pulmonary veins. Am Rev Respir Dis. 1990;141:721-726.
Self-Assessment
- At what point should a patient with Guillain-Barré syndrome whose vital capacity decreases from 50 mL/kg to 30 mL/kg within a couple of hours be intubated?
(a) When the patient’s vital capacity is 15 mL/kg
(b) When the patient’s vital capacity is 20 mL/kg
(c) When the patient’s vital capacity is 30 mL/kg
(d) When the patient’s vital capacity is 40 mL/kg
- What is the preferred cerebral perfusion pressure after traumatic brain injury?
(a) 30 to 40 mm Hg
(b) 50 to 60 mm Hg
(c) ≥60 mm Hg
(d) 70 to 100 mm Hg
Stay updated, free articles. Join our Telegram channel

Full access? Get Clinical Tree
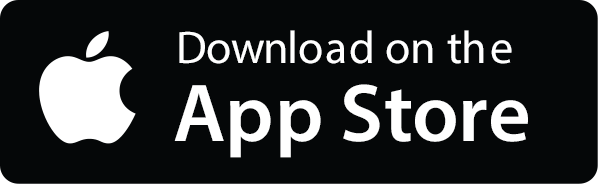
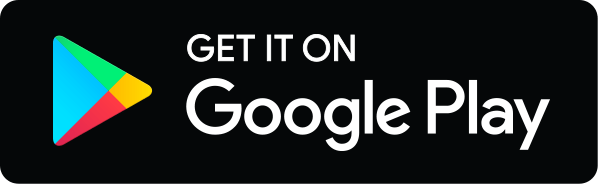