See text for explanations of points a, b, c, and d. PAW = airway pressure; Pes = esophageal pressure.
Another triggering problem can be caused by air trapping. When the patient’s effort begins, the pleural pressure decreases. Unlike the normal situation, there is no immediate decrease in airway pressure because of all the trapped pressure in the chest that must first be overcome before airway pressures in the ventilator circuit can actually change. Consequently, there is a dramatic lag in the triggering process.
The best way to deal with this is to get rid of the air trapping or intrinsic positive end-expiratory pressure (PEEPi). Reduce the minute ventilation, shorten the I:E ratio, and provide better bronchodilators. Once these efforts have been maximized, adding positive end-expiratory pressure (PEEP) at a setting lower than the PEEPi may help reduce the triggering work. Some may question adding PEEP for a patient whose lungs are already hyperinflated. The difference in this case is that the patient will not be getting more PEEP than is already in the chest. If the patient already has approximately 30 cm H2O of trapped pressure, the patient should be given anywhere from 10 to 20 cm H2O of PEEP. The additional PEEP is going to equilibrate the PEEP that is already there. Instead of 30 cm H2O in the chest and 0 cm H2O in the airway, there will now be 30 cm H2O in the chest and 10 to 20 cm H2O in the airway. That will make triggering the ventilator in the presence of all that PEEPi easier. Instead of having to bring the air trapping down to zero or less to trigger the ventilator, the patient now only has to bring the airway pressure down only maybe 5 to 10 cm H2O to trigger the breath. The patient will be much more comfortable, and the PEEPi in the chest is no worse than it was. The peak pressure, volume, and flow are all exactly the same. Applying PEEP does not eliminate the auto-PEEP; it just makes it easier for the patient to deal with the auto-PEEP.
Is an esophageal balloon necessary to do this? Although it helps, it can be done without one. Air trapping is detectable by placing a hand on the patient’s chest and looking at the airway pressure monitor. If a dramatic delay or even skipped breaths can be felt, that is a clue that there is a lot of air trapping. Empirically add 5 cm H2O of PEEP. If it gets easier for the patient to trigger, then consider going to 10 cm H2O of PEEP. If the the airway pressures increase (flow and volume ventilation), or if the volumes and flows decrease (pressure ventilation), those are signals that too much PEEP has been added. As long as the ventilator graphics stay the same while the PEEP is added and the patient is looking more comfortable, it is a good thing.
Flow
The second phase of an assisted breath is flow delivery. Ideally the patient triggers the breath and the ventilator supplies a reasonable amount of pressure, flow, and volume that will reconfigure the patient’s work of breathing to look normal. What should be avoided is the patient triggering and the ventilator support being inadequate, forcing the patient to do an excessive amount of work.
There are 2 basic ways of delivering an assisted breath, one of which is to set the flow. This is usually coupled with a volume-cycling mechanism. The problem with setting flow, which is the oldest form of assisted MV, is that the patient cannot change the flow. If the patient does not like it or wants more, the patient will literally suck the airway pressure graphic downward.
The other way to deliver an assisted breath is to set the I pressure. Both the flow and volume are variable. When the patient wants more flow, it comes out. This type of breath tends to keep up better with the patient’s effort. Pressure breaths also offer another set of tools. One goes by various different names, including the slope adjuster, rise time, or attack rate. It is the rate of rise of the pressure breath. This adjuster changes how fast the pressure setting can be reached, allowing for a very fast rise time or a very slow rise time to optimize comfort. That can be set up by creating a smooth, square wave of pressure. In essence, it involves literally going in and sculpting the breath.
The most current ventilators have feedback modes on pressure breaths for those patients who might not keep the right VT because of fluctuating respiratory drive. One is a volume guarantee that decreases pressure when the effort increases. A downside of this feedback mode is that if the patient is dyspneic and is pulling air in very hard, an inappropriately low tidal volume setting may result in the machine inappropriately bringing the pressure down. Another feedback control is the P0.1 sensor. It refers to the pressure after 100 milliseconds, before all the valves have opened up. By detecting a very vigorous effort, this feedback system will actually increase the support. It works, but whether it really improves outcomes is unclear. Another feedback mode, the end-tidal carbon dioxide (ETCO2) control, can adjust the level of pressure according to the CO2. Whether it improves outcomes also is unclear. Although one study of the ETCO2 mode has been reported, it is of questionable quality.
Another feature available on many of today’s machines is called the automatic tube compensation, automatic airway compensation, or pseudo-tracheal pressure monitoring. Basically, it calculates airway pressure near the carina and uses this to target pressure rather than circuit pressure. The reason for this is that the narrow endotracheal tube will distort the pressure breath being delivered to the patient and impose work. By targeting tracheal pressure, this feature eliminates this. The automatic tube compensation can also be used with either CPAP or pressure support (PS).
When should automatic tube compensation be used? If it is available, use it. There is no reason not to. The use of automatic tube compensation seems to make patients more comfortable, which makes using it seem to be a reasonable thing to do.
The newest feature in terms of flow synchrony is proportional-assist ventilation (PAV). The only thing that this new mode of ventilation has in common with the other modes is that it is an assisted breath. It needs a pressure or flow-effort sensor to activate the breath. Once the breath turns on, however, it is different from any other breath type. It uses a sensor at the mouth or top of the endotracheal tube that tries to measure the patient’s I flow. When the patient starts to take a breath, flow is generated out of the ventilator circuitry and the ventilator recognizes how much flow is occurring. Then the PAV ingeniously puts what is called a gain—or boost—on the effort. The more the effort there is, the more the boost; the less effort there is, the lesser the boost. It is akin to power steering, which makes it easier to turn the car wheel. Only 1 parameter has to be set: the percentage of support. That means the ventilator goes through a rather clever mechanism to determine what the compliance and resistance of the lung is. This will enable the ventilator to know how much work is going to be required to deliver a VT. The gain setting is just a simple percentage, from 0% to 100%, that indicates how much support should be supplied to the patient.
One of the problems with this mode has been how to prevent what is called runaway, a situation in which the ventilator does not recognize that the calculations for compliance and resistance need to change because of improvement, so it provides a huge assistance to the patient. Engineers have finally been able to include proper safety mechanisms to prevent this from happening.
In PAV, when effort increases, then flow, volume, and pressure increase. Conversely— and this is another potential problem with PAV—if the effort decreases, everything else decreases as well. The concern is the patient who might receive a little extra sedation and whose respiratory drive then decreases substantially and with it ventilator assistance decreases markedly. There must be a safety net. The alarms must be set up properly to ensure that there is adequate support in the case of a patient whose respiratory drive is suddenly, or even gradually, reduced.
Proportional assist and PS look very different when graphed, as illustrated in Figure 2. The patient on the left is pulling and getting 0, 5, 10, 15, and 20 cm H2O of pressure support. The VT for a given amount of effort gets bigger and bigger and bigger, but there is always a minimal VT with PS. With PS even with the most trivial of patient efforts, there is a VT. Proportional assist on the right radiates out like a fan as support rises from 0% to 90%. The VT gets bigger with more effort and more support by the ventilator, but there is no safety net – if effort is low, there is no minimum VT.
Proportional assist is now available on a couple of brands of ventilators. It performs as designed and gives comfortable support. However, there are no good outcomes trials to date to determine if ventilator times or sedation needs are reduced. It may simply be an incredibly skillful engineering design. Still, it is reasonable to use in patients with flow or cycle dys-synchrony. There will still be the PEEPi issues, and it will require monitors or alarms for patients with a low or unstable respiratory drive.
Figure 2. Peak pressure of respiratory muscles (Pmus).
Left graph: constant pressure and variable volume; right graph: variable pressure and volume. PAV, proportional-assist ventilation; PVS, pressure-support ventilation ; VT, tidal volume.
Cycle
Cycle dyssynchrony can result from breaths that are either too short or too long. In a patient with a very long I effort, the breath cuts off early, resulting in flow reversal because the patient is not yet finished. This is a very uncomfortable situation that can lead to double triggering. On the flip side, the patient whose breath is going on too long may actually create positive pleural pressure to try to shut the breath off. Although these are 2 extremes, they do explain the phenomenon.
One of the biggest cycling problems results from trying to use pressure support in obstructive airway disease. In a patient with an acute exacerbation of COPD, for example, PS behaves problematically because in the presence of a narrow airway, not much of a peak flow gets into the lungs before the pressure target is hit. Because PS is flow cycled, the patient may have an extremely long I time, which can create air trapping.
This cycle dys-synchrony can be fixed in 2 ways. The first is to put the patient in pressure-assist control and turn off the breath rate. Now all that is left are pressure-assisted breaths. They are patient-triggered, pressure-targeted, and time-cycled. The I time can be set. Thus, the pressure assist is a good replacement for PS if the clinician wants to control the I time. The second fix is to use a new feature on many ventilators that can adjust the flow-cycling criteria. Instead of its being set at 25% of peak flow, it can be adjusted to 50%, for example.
What if all of these measures in the airway are bypassed and the nerves themselves drive the ventilator? This is known as diaphragmatic electromyographic (EMG) control of ventilation. A commercial device now available can be put on the end of a nasogastric tube or an esophageal balloon. It sits on top of the diaphragm and measures the diaphragmatic contraction—or the diaphragmatic EMG control—which drives all three phases of breath delivery.
The ventilator now “knows” what the patient really wants. This is called neurally adjusted ventilatory assist (NAVA). It is a fascinating idea, but the question is whether it is worth the time, trouble, and expense, especially given that an electrode sensor must be put down the patient’s esophagus. Investigators are currently studying external sensors that work the same way. A ventilator that can actually read the patient’s neuromuscular signals could dramatically revolutionize the whole notion of synchrony.
Optimizing patient ventilator synchrony during all three phases of breath delivery is critical to reduce imposed loading, patient discomfort and the need for unnecessary sedation. Pressure targeted approaches appear to be a significant advance over older flow targeted breaths. Newer advances such as PAV and NAVA have theoretical appeal but studies showing outcome benefits to these expensive adjuncts are lacking.
Bibliography
- Mitrouska J, Xirouchaki N, Patakas D, et al. Effects of chemical feedback on respiratory motor and ventilatory output during different modes of assisted mechanical ventilation. Eur Respir J. 1999;13:873–882.
- Sinderby C, Navalesi P, Beck J, et al. Neural control of mechanical ventilation in respiratory failure. Nat Med. 1999;5:1433–1436.
Self-Assessment
- What does supplying positive end-expiratory pressure (PEEP) that is lower than intrinsic PEEP (PEEPi) do to a patient who is having PEEPi triggering difficulties?
(a) The additional PEEP will help equilibrate the PEEPi
(b) The additional PEEP will eliminate the PEEPi
(c) The additional PEEP will increase the level of PEEPi in the chest
(d) The additional PEEP will increase the peak pressure, volume, and flow
- Proportional assist ventilation (PAV) will increase which parameter(s) in patients with increasing inspiratory effort?
(a) Increase pressure
(b) Increase flow
(c) Increase volume
(d) All of the above
Stay updated, free articles. Join our Telegram channel

Full access? Get Clinical Tree
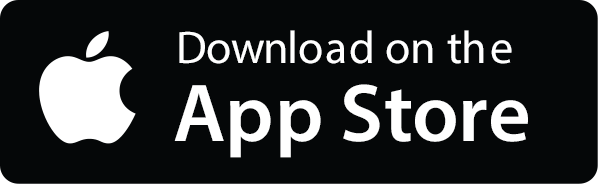
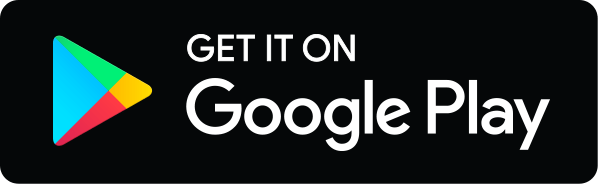