Chapter 106 Toxidromes and Their Treatment
Every year almost 2.5 million human exposures to toxic substances are reported to poison centers in the United States.1 Of these, approximately 60% involve children younger than 12 years, and this age group accounts for approximately 4% of the reported fatalities. Although 75% of calls are managed without referral to a health care facility, of the 25% of patients who are seen in a health care facility, one in eight is admitted to a critical care unit.
In most cases of poisoning, the agents involved are known, or at least circumstantial evidence points to a specific toxin or toxins. However, even in cases in which the toxic exposure is unknown or the clinical presentation is inconsistent with the history, the intensivise care physician can find physical and analytical clues of the inciting agent and provide targeted therapy. The term toxidrome, a contraction of toxic syndrome, refers to a constellation of signs associated with certain substances or groups of substances. Several toxidromes have been described; although their expression may not be complete in every case, they can provide valuable information to guide investigation and treatment. A list of toxidromes appears in Chapter 105.
The patient should be examined carefully and thoroughly, paying particular attention to vital signs, mental status, pupil size and reactivity, skin characteristics (color, temperature, moisture), and bowel sounds. Other important aspects of the physical examination include muscle tone, respiratory effort, presence of tremor, and characteristics of the mucous membranes. Laboratory investigations may help narrow the differential diagnosis and determine the need for additional examination while guiding therapy. However, diagnostic laboratory tests should be reviewed with caution. Some assays may further cloud the clinical picture because of false-positive results from cross-reactivity and may lead to an inaccurate diagnosis, inappropriate therapy, or withholding of a specific antidote.2 Utility of a specific test should be based on its probability of indicating or guiding therapy and prognosis of end-organ toxicity.
Opiates
The classic triad of respiratory depression, coma, and miosis is seen with both naturally occurring opiates and synthetic opioids. Additional features include bradycardia, hypotension, and decreased gastrointestinal (GI) motility. A similar clinical picture may be encountered with ingestion of clonidine, other imidazoline derivatives found in over-the-counter eye drops or nasal sprays, or tizanidine, all of which are centrally acting α2-adrenergic agonists that decrease sympathetic tone.3–5 Not all patients exposed to an opiate present with the classic toxidrome. Respiration becomes shallow with increasing central nervous system (CNS) depression, so hypercarbia may occur before overt respiratory depression.6–9 In addition, several opiates and opioids, including morphine, meperidine, pentazocine, diphenoxylate/atropine, and propoxyphene, may result in mid-position or mydriatic pupils either from their pharmacologic activity or from brain anoxia.3,10,11
Effects on respiration are likely a result of action at the μ-receptors. Analgesic effect is exerted through action at both μ- and κ-receptors.6 Several opioids have been designed to function as agonist-antagonists. In general, they provide spinal analgesia through agonist effects at the κ-receptors and simultaneously antagonize μ-receptors. This allows them to provide pain relief at higher doses while reaching a ceiling effect on respiratory depression.7 However, in overdose, receptor selectivity may be lost, and decreased respiratory drive can occur with these medications.
Naloxone is an opiate receptor antagonist that reverses the toxic effects of opioids. A starting dose of 0.1 mg/kg intravenously is recommended to prevent the need for artificial ventilation in life-threatening opiate-induced respiratory depression. If no intravenous access is available, it can be administered subcutaneously, intramuscularly, or via endotracheal tube. However, the intramuscular route exhibits variable pharmacokinetics with an unpredictable duration of action and may therefore allow recurrent sedation after a period during which the patient is thought to be free of further toxicity. Naloxone may precipitate acute withdrawal in opioid-dependent individuals; in such patients a lower starting dose, titrated upward to effect, may be warranted. Because of the different receptor affinities of each opioid, some individuals may not respond to lower doses of naloxone. The titration should proceed upward to 10 mg, at which point if no response has been illicited, naloxone should be discontinued and an etiology other than opioids should be considered for the cause of respiratory depression.12 In patients with apnea, artificial ventilation should be promptly provided before the administration of naloxone. Because the duration of action of naloxone is shorter than that of most opioids, repeated doses or a continuous intravenous infusion may be necessary. The accepted infusion rate is two thirds of the initial reversal dose per hour.12
Reversal of opioid toxicity has been associated with pulmonary edema, although this has also been described in the setting of opiate toxicity itself.13–16 Naloxone has been reported anecdotally to reverse clonidine overdose, although failure of naloxone to reverse clonidine has also been described.17 Naloxone administration has occasionally been associated with significant hypertension.18,19 This is usually short lived and does not require treatment. If hypertension persists, treatment should consist of a short-acting antihypertensive such as nitroprusside so that the drug and its effects can be quickly stopped. This will prevent the development of hypotension as the hypertensive crisis resolves.
Nalmefene is a newer, long-acting opiate receptor antagonist. It is available as a parenteral product with a potency equivalent to naloxone on a milligram-per-milligram basis but with a duration of action nearly five times longer.20–22 Its half-life can range from 2 to 8 hours. Before it is administered, a test dose of naloxone should be used to exclude the possibility of acute withdrawal. In at least one prospective, controlled trial, it offered no advantage over naloxone.21 It has a similar side effect profile, including cases of short-lived noncardiogenic pulmonary edema, and may provide false security in a patient who has ingested a long-acting opioid in which symptoms may recur after the antagonist has worn off.
Sympathomimetic Agents
Symptoms and signs of sympathetic excess may be seen with a number of therapeutic and illicit agents that either mimic endogenous excitatory neurotransmitters or act on receptors to increase their release.23 Drugs that produce the sympathomimetic toxidrome are listed in Box 106-1. Any or all of the manifestations in Box 106-2 may be observed depending on the agent involved. Agents with predominantly β-adrenergic activity are more likely to produce tachycardia and hypotension from peripheral vasodilation compared with agents with predominantly α-adrenergic effects that may produce severe hypertension with reflex bradycardia. Hyperthermia, rhabdomyolysis, and myoglobinuria may result from increased metabolic activity. Additional morbidity, including ischemic or hemorrhagic stroke, have been documented. With cocaine use, for example, cases of myocardial ischemia and infarction have been reported23,24 Thrombolytics should not be used unless the patient has confirmed myocardial infarction and is at low risk for intracranial bleeding.25 The methylxanthines, caffeine and theophylline, are not sympathomimetics per se, but they may produce many of the same clinical features as a result of their β-adrenergic activity and effect on adenosine receptors.26–28 Patients with acute overdose of methylxanthines may initially present with severe GI symptoms and quickly progress to hypotension, tachydysrhythmias, and status epilepticus.27,29,30 Designer amphetamines, such as methylenedioxyamphetamine (MDMA) and its derivatives, additionally cause a release of serotonin and may precipitate hallucinations in addition to the sympathomimetic effects listed above. These patients are at risk for seizures, dysrhythmias, hyperthermia, rhabdomyolysis, the syndrome of inappropriate secretion of antidiuretic hormone resulting in hyponatramia, and disseminated intravascular coagulation.28,31
Benzodiazepines are the first-line agent to reduce CNS catecholamine release, thereby eliminating severe hypertension, tachycardia, agitation, and muscle overactivity. Large doses may be required. Caution should be exercised when adrenergic antagonists are used to treat tachycardia and hypertension; β-adrenergic antagonist use may result in unopposed α-adrenergic receptor stimulation and cause a paradoxic worsening of hypertension. Although labetalol is a β-antagonist with weak α-blocking activity, it has not been shown to be effective in this scenario. Concomitant administration of a vasodilator in refractory hypertension is recommended.23,24,32 Agents such as cocaine may deplete norepinephrine, leading to cardiovascular collapse. Therefore short-acting agents are the only advisable treatment for hypertension if benzodiazepines administration has failed. Seizures should also be managed with benzodiazepines. In patients refractory to this therapy, a barbitruate such as phenobarbital should be considered. Phenytoin does not play a role in seizures resulting from toxicity and, in the case of methylxanthines, may actually worsen patient outcome by lowering the seizure threshold.28 Confirmation by urinary drug screening is of little use in the emergency setting. While a positive test result for cocaine is likely confirmatory for its use, a positive result for amphetamines is not. Several medications cross-react to produce a false-positive amphetamine presence, including buproprion2 and pseudoephedrine.23
Anticholinergic Agents
The anticholinergic toxidrome, more appropriately referred to as an antimuscarinic toxidrome, is produced by a number of agents that possess antimuscarinic properties as their primary effect or as a side effect. Examples of such toxins are provided in Box 106-3. Muscarinic receptors are located in the CNS, in the target organs of the parasympathetic nervous system (PNS), and in the sweat glands (sympathetic nervous system).33 The syndrome may have features that are similar to those of the sympathomimetic toxidrome (Box 106-4). Examination of the skin usually provides clues to differentiate between the two; the patient will be dry in this scenario versus increased diaphoresis with sympathomimetic toxicity. Hypertension and tachycardia are typically less severe than when seen with sympathomimetics. Also, the dilated pupils in the anticholinergic syndrome are nonreactive because there is associated cycloplegia.33,34
Because sweating is inhibited in intoxicated patients, treatment of agitation is important to prevent hyperthermia. Benzodiazepines are the drug of choice. Physostigmine is a cholinesterase inhibitor that may be used to reverse the central and peripheral manifestations of anticholinergic toxicity.33 Because of case reports of convulsions35 or asystole36 associated with administration of physostigmine, it should not be used to treat the anticholinergic manifestations of tricyclic antidepressant overdose. Diphenhydramine overdose can present with anticholinergic toxicity and also manifest electrocardiographic (ECG) changes, including sodium channel blockade. Therefore the use of physostigmine to reverse toxicity in this patient population should be approached with caution. In general, physostigmine has a short duration of action compared with the anticholinergic agent ingested; because of its severe side effects, it has fallen out of favor as an antidote for most previously indicated ingestions.
Cholinergic Agents
Cholinergic agents are best divided into the following three categories: muscarinic agents, nicotinic agents, and cholinesterase inhibitors. The muscarinic agents act in the CNS, at postganglionic parasympathetic nerve endings, and in the sweat glands. Nicotinic agents act in the CNS, in the autonomic ganglia (both sympathetic and parasympathetic), and at the neuromuscular junction. Cholinesterase inhibitors increase the available acetylcholine in the cholinergic synapse and present with a combination of symptoms that result from action at both the nicotinic and muscarinic receptors.37,38 Agents with cholinergic activity are listed in Box 106-5. Signs and symptoms of cholinergic excess are listed in Box 106-6.
Box 106–5 Drugs Causing Cholinergic Excess
Inhibitors of Acetylcholinesterase
Direct-acting muscarinic agents produce typical features of excessive parasympathetic activity. Nicotine produces salivation, nausea, and vomiting.37,38 Hypotension, bradycardia, and respiratory depression can be preceded by tachycardia, hypertension, and tachypnea. Central features include initial stimulation followed by seizures, lethargy, and coma. Neuromuscular blockade may occur. Management of nicotine poisoning, which can be severe, is entirely supportive.39–41 Children are at increased risk of nicotine poisoning from ingestion of cigarettes or chewing tobacco as well as from exposure to smoking cessation products, including gums, lozenges, patches, and inhalers.
Cholinesterase inhibitors, such as organophosphate pesticides and nerve agents, produce a mixed picture of toxicity. Parasympathetic manifestations tend to dominate the autonomic component of toxicity. Organophosphates bind to and inactivate acetylcholinesterase, preventing the normal termination of cholinergic stimulation at the postsynaptic receptors. The end result is excessive nicotinic and muscarinic activity in the PNS and CNS. At the neuromuscular junction, the result is depolarizing neuromuscular blockade. Over time the enzyme becomes phosphorylated, a process referred to as aging. Not all organophosphorous agents age at the same rate. Some nerve agents may age in as quickly as a few minutes, whereas some pesticides can take as long as 72 hours to permanently inactivate the cholinesteraseses.42 Cholinesterase activity is only restored by synthesis of a new enzyme.38 Carbamates bind reversibly to acetylcholinesterase, and the enzyme/chemical complex undergoes spontaneous hydrolysis, generally restoring cholinesterase function within hours. Carbamates do not penetrate the CNS well, so central manifestations are less severe. Because these agents cause bronchorrhea, bronchospasm, decreased respiratory drive, and paralysis of the muscles involved in breathing, death results from respiratory failure. The order of appearance of signs and symptoms depends on the route of administration. With dermal exposure, the first manifestation may be local hyperhidrosis, followed by systemic manifestations once the agent is absorbed through the skin. Inhalational exposure results in initial upper airway manifestations and respiratory distress. Ingestion presents with drooling and vomiting as the earliest expression of toxicity.
Treatment of cholinergic toxicity involves atropine to reverse the muscarinic effects, an oxime to reverse neuromuscular blockade, and benzodiazepines to treat seizures. Extremely large doses of atropine may be necessary, and the end point of therapy is the drying of secretions, not heart rate or pupil size. Organophosphate-poisoned patients may manifest tachycardia as a result of their exposure, and atropine is not contraindicated in these patients. Tachycardia during therapy is limited and not life-threatening.42,43 Depending on the agent involved, repeated doses or a constant infusion may be necessary. Pralidoxime is the oxime available and indicated for organophosphate poisoning in North America. It works by preventing aging of the cholinesterase and reactivating the enzyme. Pralidoxime is generally not indicated in carbamate overdose because of the reversible binding of toxin to acetylcholinesterase, which limits the duration of toxicity.38 Pralidoxime does not readily cross the blood-brain barrier, and benzodiazepines should initially be provided for the prevention of seizures.43 Cholinesterase levels can be obtained in poisoned patients and may confirm exposure and indicate the severity of toxicity. However, they are usually not available in real time and should not be used to guide therapy because this will likely delay treatment of the severely poisoned patient.
Methemoglobinemia
Methemoglobinemia results from the oxidization of iron in hemoglobin from the ferrous (2+) form to the ferric (3+) form. It results in the inability to carry oxygen; by shifting the oxygen saturation curve to the left, it decreases off-loading of any bound oxygen at the tissues.44 Methemoglobinemia is associated with drugs and toxins that cause oxidative stress (Box 106-7). Clinically, the patient appears cyanotic, and the cyanosis does not respond to the administration of oxygen. The blood may have a chocolate color, and exposure of the blood sample to oxygen does not restore a normal appearance. The diagnosis is confirmed by multiple-wavelength co-oximetry. Standard pulse oximetry, which uses only two wavelengths of light, cannot reliably be used to assess the degree of methemoglobinemia. However, newer models of pulse oximeters that use several different wavelengths of light may be used to measure methemoglobin levels. Standard pulse oximetry may overestimate or underestimate true oxygen saturation depending on the methemoglobin level.45,46 In a patient with cyanosis and a normal partial pressure of oxygen, methemoglobinemia should be suspected.
Patients may appear blue with methemoglobin concentrations as low as 15 g/L, treatment may not be required. The appearance of cyanosis depends on the total methemoglobin present. Although anemic patients have less hemoglobin to convert to methemoglobin, this is usually a larger percent of their functioning hemoglobin. So, although they may not manifest cyanosis, they are less tolerant of methemoglobin at a lower percentage and are more likely to express symptoms of hypoxia.44 Therapy should be provided according to the signs of hypoxia and not the methemoglobin level or the presence of cyanosis. If required, treatment begins with the administration of 100% oxygen (to maximize oxygen-carrying capacity with the certainty that unaffected hemoglobin is fully saturated), followed by intravenous methylene blue at a dose of 1 mg/kg. Methylene blue facilitates the reduction of the oxydized heme iron of methemoglobin to its normal state by NADPH-dependent methemoglobin reductase. Response is rapid and occurs within 30 minutes. Depending on the toxin involved, in the case of dapsone, recrudescent methemoglobinemia may be seen and may require repeated doses of methylene blue. The total (cumulative) dose should not exceed 7 mg/kg because methylene blue can itself be an oxidizing agent, cause additional methemoglobinemia, and potentially lead to hemolysis. Methylene blue may be ineffective in patients with glucose-6-phosphate dehydrogenase (G6PD) deficiency, in whom it may increase the risk of hemolysis or methemoglobinemia. In general, the patient can safely be treated with two doses of methylene blue; if no response is elicited, G6PD deficiency should be suspected. G6PD testing, however, should not be conducted until several months after resolution of the methemoglobinemia. When done concommittantly with the insult, patients will have a false-positive result for the deficiency. In nonresponding patients who are severely ill, exchange transfusion should be considered. Although hyperbaric oxygen increases the percent of oxgen-saturated blood, this is likely not beneficial for treatment of methemoglobinemia because no functioning hemoglobin has been added and patients will return to their previous state when removed from the chamber.44,47
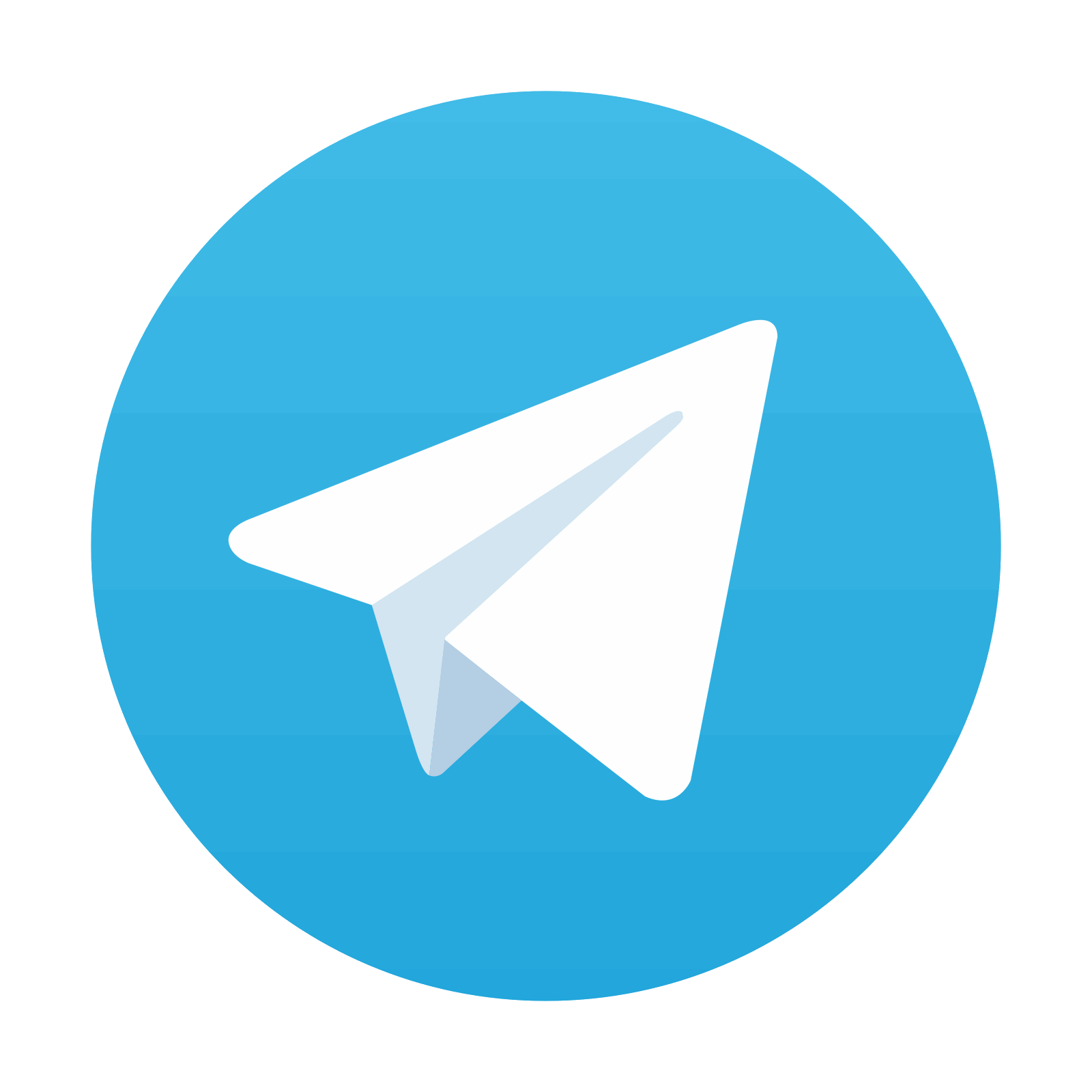