Chapter 122 Neuromuscular Blocking Agents
In the pediatric intensive care unit (PICU) setting, there may be circumstances in which total prevention of movement is necessary, thereby mandating the use of neuromuscular blocking agents (NMBAs) (Box 122-1).1 Although these agents can be used as a single dose to facilitate endotracheal intubation, more prolonged administration may be needed in specific circumstances. A survey from the PICU setting suggests that the prolonged administration of these agents is used most commonly as an adjunct in the control of intracranial pressure (ICP).2 With an improved understanding of the techniques for providing sedation and analgesia in the PICU setting and data demonstrating not only their adverse effect profile, but also their lack of efficacy in specific clinical scenarios, there has been a decrease in the prolonged administration of NMBAs.
Box 122–1 Reported Indications for Neuromuscular Blockade in the PICU
The Neuromuscular Junction
Normal neuromuscular transmission results from the release of acetylcholine from the nerve terminal, its movement across the synaptic cleft, and subsequent binding to the postsynaptic nicotinic receptor on the sarcolemma of the skeletal muscle (Figure 122-1). Acetylcholine is synthesized in the cytoplasm from acetyl coenzyme A and choline and stored in synaptic vesicles in the axonal terminals of the presynaptic membrane. Depolarization of the axonal membrane results in the opening of calcium channels (P channel) and the movement of calcium through channels in the presynaptic membrane, resulting in the fusion of the synaptic vesicles with the axonal membrane and the release of acetylcholine into the synaptic cleft. The P channel is blocked by cations such as magnesium and lithium, but not by calcium channel antagonists. As such, the concurrent administration of magnesium or lithium will potentiate the effect of NMBAs, and the excessive administration of either cation can have significant effects on normal neuromuscular function. After its release from the synaptic vesicles, acetylcholine diffuses across the synaptic cleft and binds to acetylcholine receptors on the postsynaptic membrane (sarcolemma). The acetylcholine receptor (nicotinic receptor on the sarcolemma) is a pentameric protein composed of five subunits. There are five classes of subunits (alpha, beta, gamma, delta, and epsilon) each of which is coded for by a different gene. During various stages of development or in pathologic disease states, the composition of the acetylcholine receptor may change. The normal variant of the acetylcholine receptors found in adults includes two alpha subunits combined with one each of the beta, delta, and epsilon subunits. Binding of an acetylcholine molecule to each of the two alpha subunits is necessary for opening of the channel and depolarization of the sarcolemma. Immature and denervated acetylcholine receptors have a gamma subunit instead of the epsilon while a demyelinated neuromuscular junction contains acetylcholine receptors composed of a pentamer of alpha7 subunits. The importance of these variants is that their response (opening of the ion channel) is dramatically different from the normal adult variant of the acetylcholine receptor. These differences can result in devastating consequences following the administration of succinylcholine (see below). The acetylcholine receptor occupies the space from the outside of the muscle through the cell membrane to the inside and thereby regulates the transmembrane movement of ions. It converts the chemical stimulus (acetylcholine) into an electrical impulse (depolarization of the sarcolemma). Stimulation of the acetylcholine receptors opens ion channels allowing the movement of small, positively charged cations such as sodium, potassium, and calcium. The sodium influx depolarizes the muscle membrane leading to excitation-contraction coupling, with the release of calcium from the sarcoplasmic reticulum and muscle contraction. Cessation of muscle contraction and repolarization occurs when acetylcholine is metabolized by a specific enzyme, acetylcholinesterase, which is present in the synaptic cleft. This repolarization sets the muscle for the next round of depolarization and excitation-contraction coupling.
Neuromuscular Blocking Agents: Depolarizing Agents
The two general classes of NMBAs (depolarizing and nondepolarizing agents) differ in their basic mechanism of action. Depolarizing agents such as succinylcholine (suxamethonium in Europe and the United Kingdom) mimic acetylcholine, binding to the acetylcholine receptor at the neuromuscular junction, and activating it. As succinylcholine is resistant to degradation by acetylcholinesterase, there is sustained occupation of the receptor and thereby failure of repolarization, resulting in a more prolonged duration of neuromuscular blockade. This action of succinylcholine accounts for the clinical effects that are seen, which include the initial muscle fasciculations followed by flaccid paralysis that generally lasts 5 to 10 minutes, the time necessary for the degradation of succinylcholine by pseudocholinesterase. The onset of action of succinylcholine is more rapid than any of the nondepolarizing agents, with neuromuscular blockade occurring in 30 to 45 seconds, thereby allowing for rapid control of the airway by endotracheal intubation. Succinylcholine undergoes rapid redistribution and metabolism by the plasma enzyme pseudocholinesterase (plasma cholinesterase), which limits its clinical duration to 5 to 10 minutes.
In isolated cases, a congenital or acquired deficiency of pseudocholinesterase can lead to a prolonged duration of action. In general, there may be issues with the total amount of the enzyme (quantitative defect) or the efficacy of the enzyme (qualitative defect).3 Quantitative problems are generally acquired while qualitative issues are inherited. The inherited form of pseudocholinesterase deficiency results in a qualitative defect in the enzyme. It is an autosomal recessive trait with an incidence of 1 in 2500 to 3500. Only homozygotes have a clinically significant prolongation of the effect of succinylcholine, with neuromuscular blockade lasting up to 4 to 8 hours following a single normal intubating dose of succinylcholine (1 to 2 mg/kg). Disease states that lead to a quantitative decrease in pseudocholinesterase levels include severe liver disease, myxedema, pregnancy, protein-calorie malnutrition, and certain malignancies. Drugs and medications can also affect pseudocholinesterase levels, including chemotherapeutic agents such as cyclophosphamide and echothiopate ophthalmic drops. Deficiency can also result from recent plasmapheresis as the enzyme is removed with the plasma. Treatment is aimed at prompt identification by noting failure of the return of the train-of-four (TOF) with peripheral nerve stimulation (see below). The possibility of such an occurrence stresses the need to ensure return of neuromuscular function following the administration of succinylcholine prior to the administration of a nondepolarizing agent. If such a problem is suspected, primary therapy includes continuation of ventilatory support until the patient’s muscle strength returns and the provision of amnesia with a benzodiazepine or some other anesthetic agent. Although the patient cannot move, he or she will be aware and awake once the effects of the anesthetic induction agents have diminished. The enzyme plasma cholinesterase is contained in fresh frozen plasma (FFP); however, due to the infectious disease risk with the use of blood products, reversal through the administration of FFP cannot be recommended.4–6 More recently, purified human plasma cholinesterase has also been used in such circumstances; however, such a practice is expensive and not available in most centers.
Despite its rapid onset and cessation of effect, the potential adverse effects associated with succinylcholine can be devastating and even fatal (Box 122-2). Direct effects on cardiac rhythm have been described following the administration of succinylcholine, including bradycardia, tachycardia, and atrial or ventricular ectopy.7 Succinylcholine has a chemical structure similar to two molecules of acetylcholine and may result in bradycardia from activation of cardiac muscarinic receptors. This effect is especially common in infants and children, in patients who are anesthetized with halothane (given its negative chronotropic effects), in the presence of hypoxemia, with intravenous as compared to intramuscular administration, when succinylcholine is given concurrently with other medications that have negative chronotropic effects (propofol, fentanyl), and with repeated doses. Therefore, in these scenarios, succinylcholine should always be preceded by an anticholinergic agent such as atropine. The bradycardic effects of succinylcholine may also be accentuated by hypothermia and by elevated ICP. Arrhythmias may also be seen. Although common, occurring in up to 50% of patients, they are generally short-lived and of no clinical significance. The use of an anticholinergic agent will decrease, but not eliminate the incidence of arrhythmias. As with the potential for bradycardia, arrhythmias tend to be more common with repeated doses of succinylcholine.
As succinylcholine activates the acetylcholine receptor prior to producing neuromuscular blockade, depolarization of the muscle end plate occurs, with contraction of the muscle fascicles or fasciculations. These fasciculations are responsible for the myalgias that may occur following succinylcholine administration.8 Although not a significant issue when succinylcholine is used to emergently secure the airway, these fasciculations can result in severe muscle pain; therefore many advise against the use of succinylcholine for outpatient surgery when such issues may interfere with activities of daily living and return to work. The severity of the fasciculations can be diminished by the administration of a small dose of a nondepolarizing agent (curare 0.03 to 0.05 mg/kg, rocuronium 0.05 mg/kg, or pancuronium 0.01 mg/kg) prior to succinylcholine. The dose of the nondepolarizing agent is generally one tenth of the recommended dose for endotracheal intubation.9 This is referred to as a defasciculating dose. The technique is commonly used in the operating room when succinylcholine is administered to adults, as a means of preventing or attenuating the postoperative myalgias that may occur with succinylcholine. One advantage of fasciculations is that their cessation signals that neuromuscular blockade is complete and the patient’s trachea can be intubated.
Defasciculation is not commonly used in the pediatric population for several reasons: (1) children less than 6 years of age do not fasciculate; (2) the use of the defasciculating dose delays the onset of paralysis and increases the succinylcholine dose requirement; (3) in patients with severe respiratory or hemodynamic compromise, the defasciculating dose can cause a significant degree of neuromuscular blockade leading to respiratory insufficiency or laryngeal incompetency with the risk of aspiration; and (4) full efficacy may require up to 2 to 3 minutes, thereby making the technique less optimal when emergent securing of the airway is necessary. If a defasciculating dose is used in patients who are awake and coherent, they should be warned that they may feel effects of the medication, such as diplopia from effects of the drug on the extraocular muscles. Additionally, some patients may feel effects on the muscles of ventilation and complain of shortness of breath.
In addition to myalgias, the fasciculations caused by succinylcholine may result in a transient increase in plasma creatinine phosphokinase (CPK) and myoglobin level. Myoglobinemia occurs most commonly (in up to 40% of patients) when there is concomitant administration of general anesthesia with halothane. In these patients, levels high enough to result in myoglobinuria have been reported in 8% of patients.10 The rise in plasma CPK and myoglobin levels does not occur with intramuscular administration and may be attenuated by the administration of a defasciculating dose of a nondepolarizing agent (see above). These effects should be differentiated from the potentially lethal complication of rhabdomyolysis, which may occur in patients with specific disorders of the neuromuscular junction and in malignant hyperthermia (see later discussion). These latter disorders absolutely contraindicate the use of succinylcholine. Fasciculations may also increase intragastric (IGP) and intraocular pressure (IOP). The transient and minimal rise in intragastric pressure is generally of limited clinical significance and does not increase the risk of vomiting or passive regurgitation during endotracheal intubation. In the emergency setting, when succinylcholine is chosen for endotracheal intubation, rapid sequence intubation will be used, with the application of cricoid pressure to protect against acid aspiration. The contraction of extraocular muscles leads to an increase in intraocular pressure following the administration of succinylcholine. The increase is transient, with a return of the IOP to baseline within 5 to 8 minutes. Given this effect, administration to patients with an open globe injury is generally contraindicated due to the theoretical risk of causing extrusion of the intraocular contents. Although succinylcholine has been safely administered to such patients,11 standard practice generally considers the presence of an open globe injury a contraindication to its use.
The effects of succinylcholine on intracranial pressure and its use in patients with altered intracranial compliance remain controversial. Succinylcholine increases ICP not only through the production of muscle fasciculations and increased venous tone, but also via a direct cholinergic mechanism due to activation of muscle spindles in the peripheral skeletal musculature.12 The effects on ICP are generally mild and transient. Given its rapid onset (30 to 45 seconds), succinylcholine allows for rapid endotracheal intubation and control of arterial oxygenation and ventilation. As the latter are primary determinants of ICP, any mild increase due to the direct effects of succinylcholine are rapidly controlled. Succinylcholine’s effects on muscle spindles have also been postulated as an explanation of the CNS activation and dreaming that has been reported during general anesthesia in patients who received succinycholine.13 The dreaming has not been associated with awareness or recall.
Succinylcholine has also been shown to occasionally result in a transient increase in the tone of the masseter muscles. This effect may be seen in all of the peripheral skeletal musculature, but may be accentuated in the masseter muscles, resulting in what is clinically known as masseter spasm. The effect is generally mild and can be overcome by manual opening of the mouth.14 A defasciculating dose of a nondepolarizing agent may abolish this phenomenon. In rare circumstances, the masseter spasm may be severe, preventing mouth opening and precluding standard oral endotracheal intubation. It has been suggested that patients who manifest masseter spasm to this degree are at risk for malignant hyperthermia (MH), a rare inherited disorder of muscle metabolism which, if untreated, is generally fatal (see below). The data regarding the relationship between masseter spasm and MH are conflicting. In a prospective evaluation with monitoring of masseter muscle tone, patients who developed significant increases in masseter muscle tone did not proceed to develop MH.15 However, retrospective series have suggested that the development of masseter spasm may be a prelude to MH, thereby clouding the issue as to how to deal with such patients.16 In the emergency situation, should patients develop masseter spasm following the administration of succinylcholine, patients should be monitored for signs of MH including hypercarbia, hyperthermia, tachycardia, and rhabdomyolysis with myoglobinuria. Treatment with dantrolene is suggested should there be a concern regarding the development of MH (see below).
The other major concern with succinylcholine is the occurrence of lethal hyperkalemia in patients with certain underlying disorders or comorbid diseases (Box 122-3).17 The reader is referred to reference 17 for a full discussion of the hyperkalemic response following succinylcholine. While many of the disorders listed in Box 122-3 are readily apparent, such as the muscular dystrophies, the occurrence of cardiac arrest following succinylcholine administration to apparently healthy children has led to a restructuring of the recommendations for the use of succinylcholine. The problem is that some children with muscular dystrophy may not manifest symptoms until they are 4 to 6 years of age. If succinylcholine is administered to these children during perioperative care or other clinical scenarios, lethal hyperkalemia can occur. Because of such problems, the current recommendations are that succinylcholine should only be used for emergency airway management when rapid endotracheal intubation is necessary, when there is a concern about the ability to provide endotracheal intubation (potentially or documented difficult airway), or when intramuscular administration is needed because an appropriate intravenous access cannot be secured. Also of concern in the pediatric population are patients with relatively rare genetic, chromosomal, or metabolic defects in whom the effects of succinylcholine have not been evaluated. In such settings, the risk/benefit ratio of succinylcholine must be fully examined. In many of these patients, the use of a rapidly acting, nondepolarizing agent may be the best option. However, succinylcholine is generally an acceptable option in patients with cerebral palsy and related problems. Regardless of the clinical scenario, if problems occur following the administration of succinylcholine, hyperkalemia should be suspected and the resuscitation tailored to treat it.
In emergency situations when intravenous access cannot be readily obtained, succinylcholine can be administered intramuscularly in a dose of 4 to 5 mg/kg. Intramuscular administration will result in neuromuscular blockade sufficient to allow for endotracheal intubation in 2 to 3 minutes and will rapidly (less than 30 seconds) treat laryngospasm occurring during anesthetic induction when intravenous access is not available, thereby allowing for effective bag-valve-mask ventilation. In this scenario, it is generally recommended that succinylcholine be administered into the deltoid muscle as the onset times are more rapid than with administration into the quadriceps. Alternatively, administration into the tongue or the submental space has been suggested as blood flow to this area is generally well-maintained even when peripheral vasoconstriction has occurred. Unlike intravenous administration, there is limited risk of bradycardia with IM administration.18 However, the IM route is not recommended in patients with conditions that decrease cardiac output or blood flow to the muscles such as shock or bradycardia. In the latter situation, the onset of action will be significantly delayed. Given these concerns, IM administration is generally not recommended in critically ill children and intraosseous administration (1 to 2 mg/kg) should be considered when IV access is not available.19
Currently, the package insert and good clinical practice allow for the administration of succinylcholine when there may be a potentially difficult airway, in the emergency situation when rapid securing of the airway is necessary (full stomach when a rapid sequence intubation is performed), and when there is no intravenous access (IM administration), provided that there is no contraindication to its use (see Box 122-3 and reference 17). When dealing with the potentially difficult airway or unrecognized difficult airway, the major advantage of succinylcholine is that there should be return of normal neuromuscular function within 10 minutes as opposed to 60 minutes following a 1 mg/kg intubating dose of rocuronium (see later discussion). Dosing recommendations for succinylcholine vary from 1 to 2 mg/kg.20 Larger doses are not likely to improve intubating conditions, and they slightly prolong the duration of action.
Neuromuscular Blocking Agents: Nondepolarizing Agents
The nondepolarizing NMBAs act as competitive antagonists at the neuromuscular junction, and block the effects of acetylcholine at the receptor. Unlike succinylcholine, these agents do not activate the acetylcholine receptor and therefore do not result in fasciculations. Nondepolarizing NMBAs are commonly used in the operating room to facilitate endotracheal intubation and to provide ongoing muscle relaxation for specific surgical procedures such as exploratory laparotomy. When used to provide ongoing neuromuscular blockade in the operating room or the intensive care unit, these agents can be administered by intermittent bolus dosing or by continuous infusion. There are two basic chemical structures of the nondepolarizing NMBAs available for clinical use: aminosteroid and benzylisoquinolinium compounds (Box 122-4). The difference in their chemical structure has limited clinical significance. Of more importance are differences regarding onset, duration of action, cardiovascular effects, metabolism, metabolic products, and cost. These principles will be reviewed in the remainder of this chapter.
The first nondepolarizing NMBAs (curare, gallamine, metocurine), which were introduced into clinical practice in the 1940s, are rarely, if ever, used in today’s clinical practice. The past 20 years have seen a rapid growth in the development and introduction of nondepolarizing NMBAs for clinical use. As these agents have more favorable profiles (onset times, recovery times, metabolic fate), they have displaced the original group introduced in the 1940s. However, with the introduction of new agents comes the potential for unrecognized morbidity and even mortality related to adverse physiologic effects. This potential is illustrated by the introduction and subsequent withdrawal of rapacuronium (see below). Given the potential for problems with succinylcholine, the search continues for a nondepolarizing NMBA with similar onset and cessation of action.
Pancuronium
Pancuronium is an aminosteroid compound. It is generally available in a solution containing 1 mg/mL of pancuronium, although other concentrations are available, depending on the manufacturer. The common clinical dose, 0.1 to 0.15 mg/kg, provides adequate conditions for endotracheal intubation in approximately 90 to 120 seconds. Although the higher end of the dosing range may speed the onset time to acceptable conditions for endotracheal intubation, the clinical duration is prolonged from 40 to 60 minutes to 70 to 80 minutes. Given its duration of action, pancuronium is considered a long-acting NMBA (Box 122-5). The effective dose in 95% of children (ED95) is 52 μg/kg during halothane anesthesia and 81 to 93 μg/kg during opioid-based anesthesia. The latter is more applicable to the PICU setting.21 Further study has shown that the ED95 in children is slightly higher than that of adolescents (77 μg/kg).22 Following a dose of 70 μg/kg, the onset of neuromuscular blockade occurs more quickly in children when compared to adults, with 90% twitch ablation occurring at an average of 2.4 minutes in children and 4.3 minutes in adults.23 The time to return of the twitch height to 10% of baseline was 25 minutes in children and 46 minutes in adults.
Given its long half-life, pancuronium is generally administered intermittently to provide ongoing neuromuscular blockade in the PICU setting. One prospective study has evaluated dosing requirements in the PICU population.24 The study cohort included 25 patients, ranging in age from 3 months to 17 years and in weight from 3.2 to 68 kilograms. Pancuronium was administered as an initial bolus dose of 0.1 mg/kg, followed by an infusion starting at 0.05 mg/kg/hr. The infusion was titrated up and down to maintain one to two twitches on TOF testing (see below). Pancuronium infusion requirement averaged 0.07 ± 0.03 mg/kg/hr for the 1798 hours of the infusion. Approximately 70% of the time, the infusion requirements were within the range of 0.05 to 0.08 mg/kg/hr. Seven patients were receiving anticonvulsant agents, including pentobarbital, carbamazepine, felbamate, valproic acid, phenytoin, and phenobarbital. Increased pancuronium infusion requirements were noted in these patients (0.14 ± 0.06 vs. 0.056 ± 0.03 mg/kg/hr) and in patients that received pancuronium for more than 5 days. The requirements on day 1 were 0.059 mg/kg/hr versus 0.083 mg/kg/hr on day 5. Upon discontinuation of the infusion, time to spontaneous recovery of neuromuscular function (return of the TOF to baseline and sustained tetanus to 50 Hz) varied from 35 to 75 minutes. No adverse effects directly related to pancuronium were noted. The authors concluded that pancuronium could be effectively administered by continuous infusion to provide neuromuscular blockade in the PICU setting and that it provided a cost-effective alternative to other available agents.
Vecuronium
At doses of 0.1 to 0.15 mg/kg, acceptable intubating conditions are provided in 90 seconds, with a clinical duration of action of 30 to 40 minutes, making it an intermediate-acting agent.25 Increasing the dose to 0.3 mg/kg speeds the onset time to acceptable conditions for endotracheal intubation to 60 to 75 seconds, but also prolongs the duration of neuromuscular blockade to 60 to 90 minutes. Even with higher doses, vecuronium is devoid of cardiovascular effects. Metabolism is primarily hepatic (70% to 80%); however, hepatic metabolism results in the production of pharmacologically active metabolites, which are water soluble and therefore dependent on renal excretion. These metabolites possess roughly half of the neuromuscular blocking effects of the parent compound. This combined with the 20% to 30% renal excretion of the parent compound results in a prolonged clinical duration in patients with renal insufficiency. Given its 70% to 80% dependency on hepatic metabolism, the duration of action is also prolonged with hepatic insufficiency. Additionally, due to immaturity of the hepatic microsomal enzymes, a prolonged duration can be expected in neonates. Vecuronium in doses of 0.1 and 0.15 mg/kg maintained neuromuscular blockade at greater than or equal to 90% of baseline for 59 and 110 minutes in neonates and infants, 18 and 38 minutes in children, and 37 and 68 minutes respectively in adolescents.26 The opposite effect occurs with the chronic administration of anticonvulsant agents, with resistance to the neuromuscular blocking effects and increased dose requirements in patients receiving phenytoin.27 A similar effect has been reported with other anticonvulsant agents and NMBAs of the aminosteroid group. Given its lack of hemodynamic effects and its current availability in generic form, thereby providing a cost-effective agent for neuromuscular blockade, vecuronium remains a commonly used agent when administered by continuous infusion for ongoing neuromuscular blockade in the pediatric ICU setting.
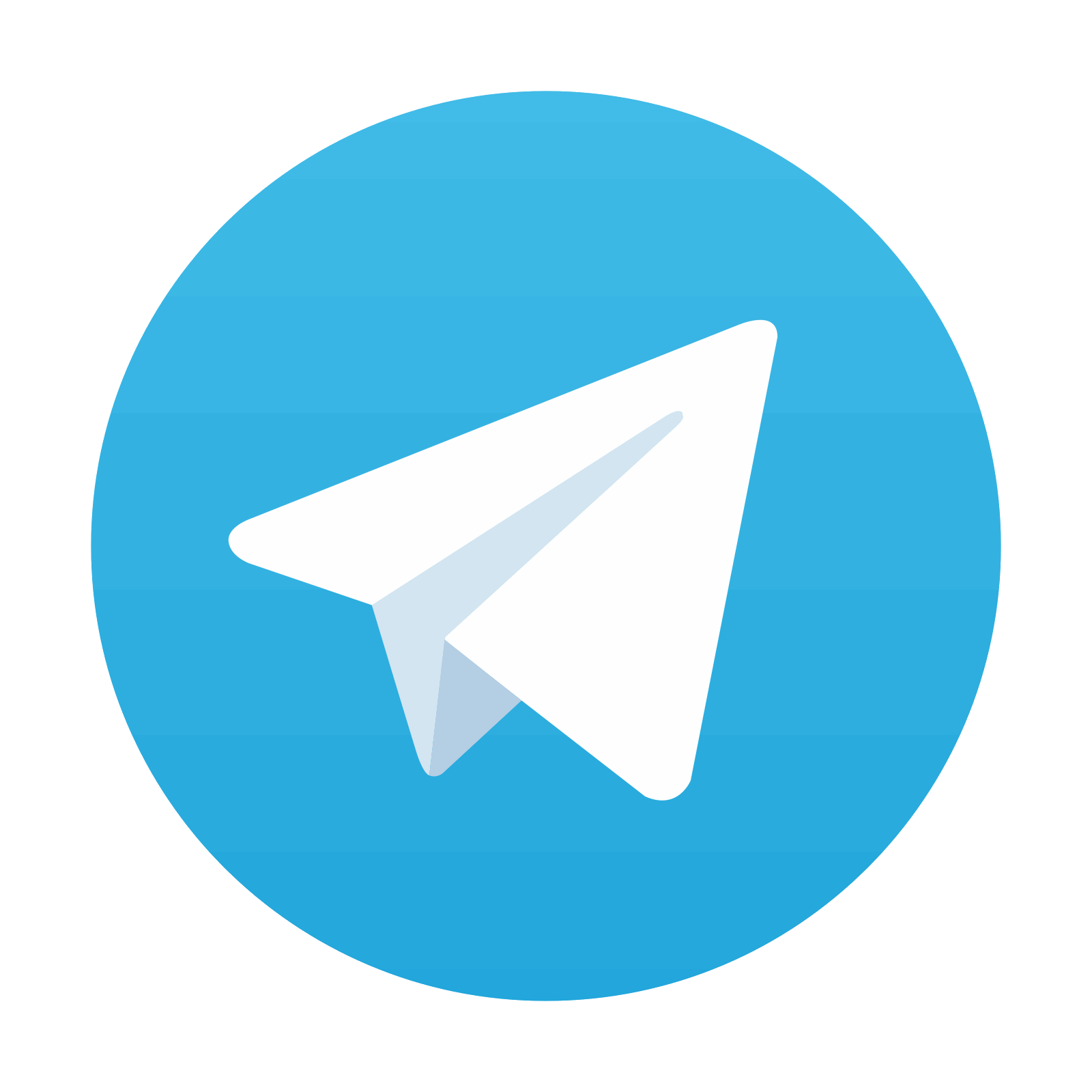
Stay updated, free articles. Join our Telegram channel

Full access? Get Clinical Tree
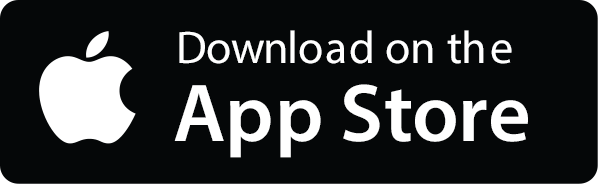
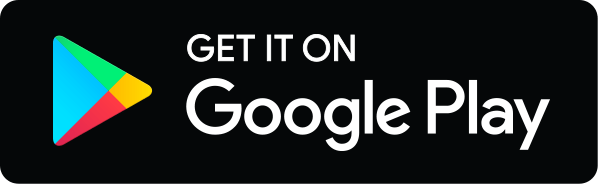